The intact and the imperilled: contrasting mammal population trajectories between two large adjacent islands
Georgina Neave


A
B
Abstract
Native mammals continue to suffer widespread and severe declines across northern Australia’s tropical savannas. There is an increasing body of evidence that the primary driver of these declines is predation by feral cats (Felis catus) and that this is exacerbated by high-severity disturbance regimes (frequent high-intensity fires, and grazing and trampling by exotic megaherbivores) that simplify habitat, thereby increasing hunting efficiency. The large islands off the northern Australian coast – where some threats are either reduced or absent – provide a means of testing the conceptual model’s predictions.
To compare the trajectory and distribution of native mammal populations on two large, adjacent islands with markedly different disturbance regimes.
In 2020 and 2021, we resurveyed 111 historical sites across the two largest of the Tiwi Islands, Bathurst Island (42 sites) and Melville Island (69 sites) that were previously surveyed between 2000 and 2002. The Melville Island sites had also been resurveyed in 2015. We used the same live trapping method used in 2000–2002, supplemented with camera trapping.
On Bathurst Island, feral cats are rare, and we found no significant decrease in native mammal trap success or species richness, and the threatened brush-tailed rabbit-rat (Conilurus penicillatus melibius) appears stable. Conversely, cats occurred at relatively high abundance on Melville Island, and there was a 52% decline in trap success, a 47% reduction in species richness, and a 93% decline in trap success for the brush-tailed rabbit-rat over the 20-year period. The highest decreases in native mammal abundance and richness were in areas that were frequently burnt and had higher activity of feral cats. In contrast, in the absence of cats on Bathurst Island, native mammal abundance increased in frequently burnt areas.
While Bathurst Island remains one of Australia’s most important refuges for native mammals, neighbouring Melville Island is experiencing severe and ongoing mammal decline. We contend that this pattern primarily reflects the high abundance of cats on Melville Island compared to Bathurst Island.
Native mammal decline in northern Australian savannas is associated with abundant feral cats, but the relative contribution of disturbances in driving cat abundance remains less clear. An improved understanding of the constraints to feral cat populations in tropical savannas could enhance conservation management.
Keywords: Conilurus penicillatus, disturbance regimes, feral cats, feral herbivores, fire, native mammal declines, Tiwi Islands, tropical savannas.
Introduction
Australia has the tragic distinction of being the world leader in modern mammal extinctions. Since European colonisation in 1788, at least 30 terrestrial mammal species, all endemic, have become extinct, and many others have suffered significant range contractions (Woinarski et al. 2015). Until just a few decades ago, the mammal assemblages of northern Australia’s monsoon tropics were thought to have avoided such declines (Ziembicki et al. 2015). However, dramatic declines have since been documented across the monsoon tropics (Woinarski et al. 2010, 2011a; Ziembicki et al. 2013; Davies et al. 2018a; Campbell et al. 2022). As in other parts of Australia, declines in northern Australia have been concentrated in the ‘critical weight range’ (species with body weight of 35–5500 g (Burbidge and McKenzie 1989; Murphy and Davies 2014)) and in more open habitats and less rugged areas, especially in lower rainfall zones (Fisher et al. 2014; Stobo-Wilson et al. 2019; von Takach et al. 2020). Presently, only a few isolated regions in the monsoon tropics are known to support comparatively healthy mammal populations: the Tiwi–Cobourg bioregion (consisting of the Tiwi Islands and Cobourg Peninsula) in central northern Australia; parts of the North Kimberley bioregion in northwestern Australia; and some offshore islands in the Arnhem Coast bioregion, of which the largest is Groote Eylandt (Start et al. 2007; Murphy and Davies 2014; Heiniger et al. 2020).
Halting and reversing the decline of mammals in monsoonal northern Australia is now one of Australia’s most urgent conservation challenges, but land managers have been largely unable to implement an effective large-scale management response. This is because the underlying causes of the northern mammal declines remain inadequately understood, although a range of threatening processes have been implicated, most notably: predation by feral cats (Felis catus) (Frank et al. 2014; Davies et al. 2017); increased fire frequency and severity (Pardon et al. 2003; Andersen et al. 2012; Griffiths et al. 2015); and habitat degradation by high densities of managed (i.e. pastoral livestock) and unmanaged (i.e. feral) exotic megaherbivores, such as cattle and buffalo (Kutt and Woinarski 2007; Legge et al. 2011; Woinarski et al. 2011a; Radford et al. 2015, 2021). Indeed, there is an increasing body of evidence that the primary driver of declines is predation by feral cats, and that this is exacerbated by disturbances (such as fire, and grazing and trampling by exotic megaherbivores) that simplify habitat, thereby increasing the hunting efficiency (McGregor et al. 2014, 2015; Leahy et al. 2015) and potential abundance of cats (Hradsky 2020).
Understanding the northern mammal decline has been largely based on the observed correlation between mammal decline and the implicated threats (e.g. abundance of cats and exotic megaherbivores, frequency and severity of fires (Davies et al. 2020; Stobo-Wilson et al. 2020a; Radford et al. 2021). However, as these threats are near ubiquitous across northern Australia, and often strongly intercorrelated themselves, it has been difficult to gauge the relative importance of each threat in isolation. Northern Australia’s numerous islands provide an excellent natural laboratory to help us understand the relative importance of drivers of mammal decline, because the various potential factors present on the mainland are often absent, or much reduced in their severity. In particular, the Tiwi Islands (comprising two of Australia’s largest islands, Melville and Bathurst) are an excellent model system for studying the drivers of northern Australian mammal declines. Both islands are free of the toxic cane toad (Rhinella marina), an invasive species that has devastated populations of some predatory native vertebrates on the mainland (Woinarski et al. 2008; Doody et al. 2009; Shine 2010). However, the islands differ markedly from each other in their densities of cats and exotic megaherbivores. Bathurst Island has no exotic megaherbivores (although pigs (Sus scrofa) are widespread and abundant) and low densities of feral cats were recently reported from two locations (Davies et al. 2022a). On neighbouring Melville Island, water buffalos (Bubalus bubalis), horses (Equus caballus) and cats are relatively abundant (Puruntatameri 2001; Kalippa et al. 2003; Davies et al. 2022a). Compared to mainland areas, the Tiwi Islands remain an important refuge as they support relatively healthy populations of native mammals. Of particular note are remnant populations of the brush-tailed rabbit-rat (Conilurus penicillatus subsp. melibius), a species that has declined severely on the mainland (Firth et al. 2010; Woinarski et al. 2014), on both islands. However, evidence of marked declines in native mammal populations has been documented for Melville, the larger of the two islands (Davies et al. 2018a), and this is linked to the occurrence of feral cats, at least for the brush-tailed rabbit-rat (Davies et al. 2017). The stability of mammal populations on neighbouring Bathurst Island has never been assessed.
In this study we explore the importance of key hypothesised drivers of the northern mammal decline, by comparing the rates of decline of native mammal populations with particular focus on the brush-tailed rabbit-rat, given its apparent vulnerability to decline in other regions. We collected and analysed data from historical survey sites across both islands, to determine the status of native mammal populations on Bathurst Island and to determine if the declines initially reported on Melville Island are ongoing. We hypothesised that the island with a low abundance of feral cats and no exotic megaherbivores (i.e. Bathurst) would support stable populations of the brush-tailed rabbit-rat and other native mammals, in marked contrast to the island with abundant feral cats and megaherbivores (i.e. Melville Island).
Materials and methods
Study site
Melville (5786 km2) and Bathurst (1639 km2) Islands are the largest of the Tiwi Islands, and are Australia’s second and fifth largest islands, respectively. They receive the highest rainfall totals in northern Australia’s wet–dry monsoon tropics. A gradient in annual rainfall exists across the islands, ranging from 1400 mm on eastern Melville to 2000 mm on northwestern Melville and central north Bathurst. Temperatures are high year-round, with a mean annual minimum and maximum temperature of 19°C and 33°C, respectively (Australian Bureau of Meteorology 2023). Narrowly separated by the Aspley Strait (<500 m), both islands are topographically simple and of low relief (≤103 m above sea level), with a notable absence of dissected sandstone escarpment that dominates parts of the adjacent mainland. The Tiwi Islands are Aboriginal freehold land with a population of approximately 2500 people across three small towns: Wurrumiyanga, Milikapiti and Pirlangimpi (Fig. 1).
Location of the 111 sites surveyed in 2021 and 2020 on Melville (69) and Bathurst Island (42). Filled black circles represent the 107 sites where both camera trapping and live trapping were conducted. Grey circles represent the two sites on Melville Island where only live trapping occurred. Open circles represent the two sites on Bathurst Island where only camera trapping occurred. Current extent of Acacia mangium plantation on Melville Island is shown in grey shading. Exotic mammal icons denote what species are present on each island. Boxed species are present in low abundance. Symbols courtesy of the NESP Resilient Landscapes Hub (nesplandscapes.edu.au).
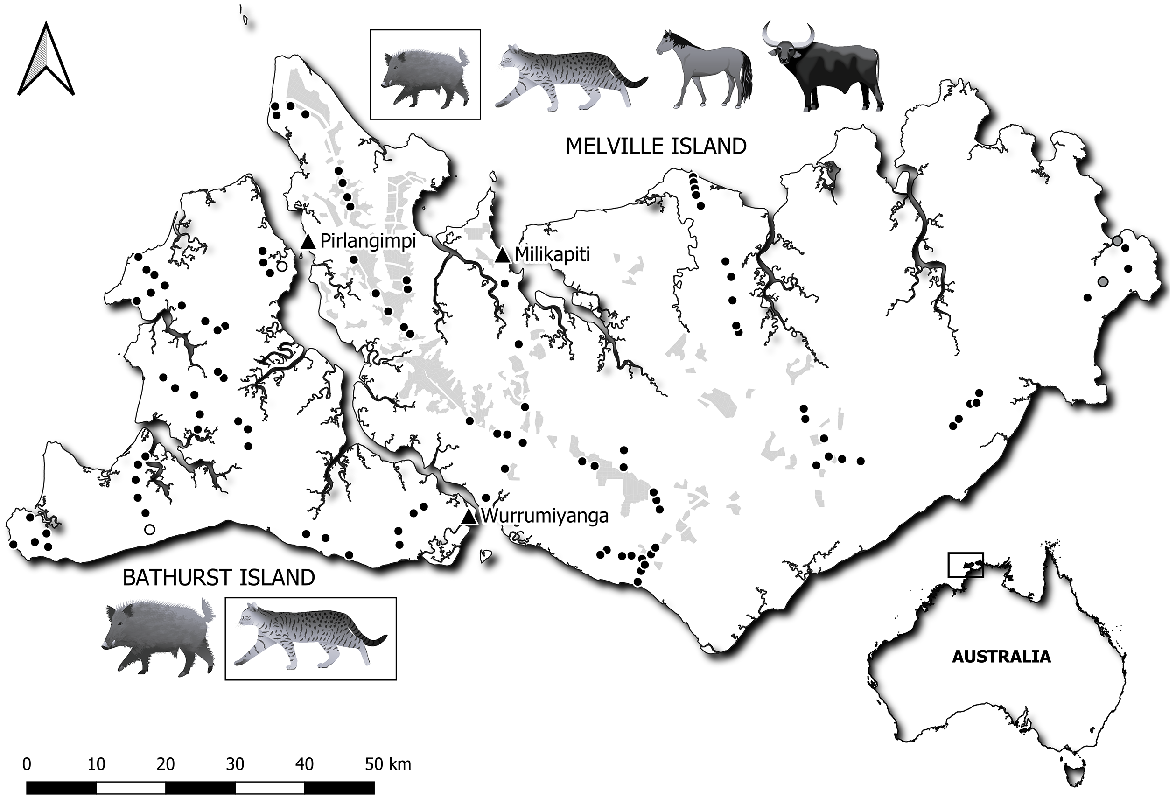
Eucalypt (Eucalyptus and Corymbia spp.) open forest and woodland (both forms of the tropical savanna biome) cover approximately 75% of both islands (Woinarski et al. 2003). They are dominated by Darwin woollybutt (Eucalyptus miniata), Darwin stringybark (E. tetrodonta) and Melville Island bloodwood (Corymbia nesophila), over a grass or shrub-dominated understorey. Fire frequency is generally high on both islands, as is typical of tropical savannas. Between 2000 and 2021, the mean proportion of the island that burnt each year was approximately 41% of Melville Island (with 85% burning in the late dry season: July to November) and 32% of Bathurst Island (with 75% burning in the late dry season) (Darwin Centre for Bushfire Research 2023). In 2017, the Tiwi landowners commenced a project to generate carbon credits through savanna fire management, using prescribed burning to reduce the frequency and extent of late dry season fires. The project covers approximately 75% of the Tiwi Islands. Melville Island has northern Australia’s largest forestry plantation, largely established in 2001. It involves 35,000 ha of short-rotation Acacia mangium plantations, representing ca. 5% of its land area (Fig. 1). There are no plantations on Bathurst Island.
A range of feral animal species have been introduced to the Tiwi Islands over the last two centuries. Melville Island has water buffalo, introduced in 1826, and horses, likely introduced in the 1890s (Morris 2001; Puruntatameri 2001). Buffalo are common and widespread across most of the island except for the northwestern peninsula around Pirlangimpi (Fig. 1). Some wetland areas of eastern Melville have recorded extremely high densities (>10 individuals km−2, Bayliss et al. 1989). Horses are widespread, but their total population is much smaller than that of buffalo. Recent aerial surveys estimated total population sizes of ca. 7500 buffalo and 575 horses on Melville Island (G. Neave unpublished data). Neither buffalo nor horse are present on Bathurst Island. Pigs (Sus scrofa) were introduced to Bathurst Island in the early 1900s and are now abundant and widespread. Pigs were introduced to Melville Island in the late-1990s and remain restricted to the western-half of the island (Kalippa et al. 2003). Feral cats are present on both islands but are in higher densities on Melville Island (0.10–0.21 cats km−2, Davies et al. 2022a), whereas cat abundance is so low on Bathurst Island (0 detections out of 12,600 camera trap nights), that density has not been able to be estimated (Davies et al. 2022a). The timing of the arrival of cats to the Tiwi Islands is ambiguous due to their cryptic nature and the lack of historical records (Abbott 2008). Cats could have arrived as far back as the establishment of the British military outpost, Fort Dundas, in 1824, or even earlier with Macassan traders. However, they also could have arrived appreciably later, alongside Catholic missionaries in the early 20th Century. Dingoes (Canis familiaris) are present on both islands, having been brought to the Australian mainland and some of its larger islands by humans sometime during the Holocene (between 4000 and 12,000 years ago (Fillios and Taçon 2016)). Cane toads are absent from both islands.
Data collection – live trapping
Between 2000 and 2002, small (c. 10 g cf. 2 kg), non-volant mammals had been systematically surveyed at 236 sites on Melville Island and 115 sites on Bathurst Island (Firth et al. 2006). Each site comprised a 50 × 50 m quadrat with 20 metal box traps (33 × 10 × 9 cm) spaced equally around the perimeter with one cage trap (56 × 20 × 20 cm) on each of the four corners. Traps were baited with a mixture of peanut butter, oats and honey and set for three consecutive nights. Many of the sites on Melville Island were resurveyed in 2015 (Davies et al. 2018a) but using a different protocol and so the results are not directly comparable.
We replicated the same live trapping protocol at a subset of the sites between 2020 and 2021. Between July and October 2020, 40 of the original 115 sites on Bathurst Island were surveyed. On Melville Island, 69 of the original 236 sites were resurveyed between June and October 2021. All resampled sites were in lowland savanna woodland or open forest and separated by a minimum distance of 1 km. Resurveyed sites were chosen to capture the variation in rainfall and fire frequency across the two islands.
The original surveys included some methods not repeated in 2020 and 2021: pitfall trapping and 10-min active searches (Firth et al. 2006). Therefore, to directly compare live trapping results between the sampling years, we omitted observations from the original surveys that were recorded using pitfall trapping and 10-min active searches. We acknowledge the possibility of bias resulting from excluding pitfall trap records, as an animal caught in a pitfall trap on a particular night would no longer be available for capture in a metal box or cage trap. However, this bias relates only to species small enough to be caught in pitfall traps, such as native mice and small dasyurids (Smithopsis spp.) In 2000–2002 there were no small mammals caught in pitfall traps at the resampled sites. We excluded records of the savanna glider (Petaurus ariel) as the ground-based survey methods used in this study were considered unreliable for surveying this strongly arboreal species (Stobo-Wilson 2018).
Data collection – camera trapping
Our 2020–2021 resampling of Melville and Bathurst Island sites also included camera trapping, although this sampling method was not used in 2000–2002. At each site we deployed five Reconyx Hyperfire camera traps (Reconyx Inc., Holmen, WI, USA) 50 m apart in a diamond formation. The central camera was positioned to be in the middle of the live trapping quadrat. Two of the five cameras had a white-flash (Reconyx models: Hyperfire 1 PC850 or Hyperfire 2 HP2W) and three had an infrared-flash (Reconyx models: Hyperfire 1 PC800 or Hyperfire 2 HP2X). The same combination of models was used at each site to control for operational variability between the models (Heiniger and Gillespie 2018). Cameras were attached to trees 40 cm above the ground (measured to the top of the camera housing) and positioned to face south. Cameras were angled towards a bait station placed 150 cm away containing a peanut butter, oats and honey mixture. Care was taken to ensure that the base of the bait station was in the centre of the camera image field of view. Understorey vegetation and leaf litter in the camera’s field of view was cleared to prevent false triggers and reduce the fire risk. All cameras were programmed to operate continuously (24-h per day) for a minimum of 5 weeks and overlapped in timing with the live trapping surveys. Cameras were set to take five photographs per trigger, with no delay between images and sensitivity was set to ‘high’ on Reconyx Hyperfire 1 models and ‘very high’ on Hyperfire 2 models.
The images generated from Bathurst Island were manually identified to species. The image dataset generated from Melville Island was first processed in MegaDetector (MDv4), an object detection model created by Microsoft specifically for processing camera trap data (Beery et al. 2019). Images that were classified as containing animals by MegaDetector were then manually identified to species in the program Timelapse (Greenberg 2020).
Data analysis – live trapping data
Our analysis focused on mammal abundance and richness. As an index of mammal abundance (overall and for each species), we used nightly trap success (following Firth et al. 2006): total number of captures/(number of traps × number of nights traps open). As an index of mammal richness, we used the number of mammal species detected at a site across all trap types during the survey period. To investigate differences in these indexes on the two islands, we used Wilcoxon matched-pairs tests to compare overall trap success, site-level species richness and species-specific trap success at the 109 sites where live trapping was undertaken in both 2000–2002 and 2020–2021. In addition, we also calculated naïve site occupancy for each species (the proportion of sites where the species was detected on each island) using live trapping data, and compared this between the 2000–2002 and 2020–2021 surveys.
Data analysis – camera trapping data
To aid in the interpretation of live trapping data, naïve occupancy was calculated from camera trap data for each native mammal species, using pooled data across the five cameras at each site. We combined records of Butler’s dunnart (Sminthopsis butleri) and red-cheeked dunnart (S. virginiae) into a single taxon, Sminthopsis spp., due to the difficulty in species-level identification from camera trap images (Potter et al. 2019).
For horses, buffalos, pigs, cats and dingoes recorded by camera traps, we calculated naïve occupancy as well as relative activity indices (RAI) for each island. For each species, the RAI was the number of independent detections (defined as at least a 30-min time interval between successive camera triggers of the same species on any of the five cameras at a site) divided by the number of camera trap nights, and multiplied by 100 (O’Brien et al. 2003). To compare dingo, cat, and pig (the species detected on both islands) RAI between islands, Wilcoxon rank-sum tests were performed. Fisher’s exact tests were used to compare naïve occupancy rates by calculating the relative abundance of sites with and without species detections on each island. Fisher’s exact tests determined if there were more non-detection sites on Bathurst or Melville than expected due to chance.
The 2000–2002 surveys included 2880 live trapping nights on Bathurst and 4968 live trapping nights on Melville Island. The 2020–2021 surveys included the same live trapping effort with an additional 10,074 camera trapping nights on Bathurst Island and 12,632 camera trapping nights on Melville Island.
Correlates of change in mammal abundance and richness
To examine correlates of change in mammal species richness and relative abundance at sampling sites we derived a range of explanatory variables based on hypotheses for mammal decline and/or distribution in northern Australia, focusing particularly on those that have potentially undergone changes in the last two decades on the Tiwi Islands or that differ considerably between the islands (Table S1). These hypotheses relate to environmental productivity (rainfall), the distribution and abundance of key predators (feral cats and dingoes), and disturbances driving changes in habitat (Anthropogenic disturbances: plantation area, fire activity, exotic megaherbivore and feral pig activity). We examined two fire variables: fire frequency, the number of times a site had burnt during the intervening years between sampling and fire activity, the proportion of area burnt each year out to a 3.2-km radius from each site and averaged over the intervening years between sampling (as per Lawes et al. 2015). We generated RAI for exotic megaherbivores, pigs, cats and dingoes from detections across the five cameras at each site. All other explanatory variables were generated using remotely sensed or GIS layers. Refer to Table 1 for a description of the final set of explanatory variables used in modelling.
Explanatory variable | Description | Island model | |
---|---|---|---|
Fire frequency – years burnt | The number of times a site had been burned between sampling sessions (2002–2019). Derived from Northern Australia Fire Information (NAFI) dataset (https://firenorth.org.au/nafi3/). | Melville Island and Bathurst Island | |
Rainfall | Mean annual rainfall (1991–2020) resolution ~5 km; source: Australian Bureau of Meteorology. | Melville Island and Bathurst Island | |
Dingo relative activity index | The number of independent dingo detections at each site (defined as at least a 30-min time interval between successive camera triggers of the same species on any of the five cameras at a site) divided by the number of camera trap nights, and multiplied by 100 (O’Brien et al. 2003). | Melville Island and Bathurst Island | |
Feral cat relative activity index | The number of independent cat detections at each site (defined as at least a 30-min time interval between successive camera triggers of the same species on any of the five cameras at a site) divided by the number of camera trap nights, and multiplied by 100 (O’Brien et al. 2003). | Melville Island and Bathurst Island | |
Pig relative activity index | The number of independent pig detections at each site (defined as at least a 30-min time interval between successive camera triggers of the same species on any of the five cameras at a site) divided by the number of camera trap nights, and multiplied by 100 (O’Brien et al. 2003). | Melville Island and Bathurst Island | |
Exotic megaherbivore relative activity index | The number of independent water buffalo and horse detections at each site (defined as at least a 30-min interval between successive camera triggers of either species on any of the five cameras at a site) divided by the number of camera trap nights, and multiplied by 100 (O’Brien et al. 2003). | Melville Island only | |
Plantation area | The area of land (hectares) under forestry plantation. Calculations were made using an area with radius of 3.2 km from each trapping site. | Melville Island only |
We explored the correlation between our explanatory variables and the change in native mammal abundance, and species richness. Standard regression models (e.g. generalised linear models (GLMs)) are not suitable for response variables like the proportional change in species richness or abundance due to the excessive number of values of −1, which indicate a complete loss of species or individuals. Furthermore, a portion (12%) of the dataset had values of zero for species richness and abundance in the original baseline surveys (2000–2002), making it impossible to calculate proportional change, and consequently, these sites would have been excluded. Therefore, to represent change in response variables, rather than calculating proportional change (i.e. the relative difference between the initial and the final values) we used a metric that calculates the proportion of the final value relative to the sum of the initial and final values. The change in abundance metric was calculated as A2/(A2 + A1) where A is the total number of individuals of all species live trapped at a site in either sampling session 1 or 2. Similarly, the change in species richness metric was calculated as R2/(R2 + R1) where R is the number of species trapped at a site in either sampling session 1 or 2. If abundance or richness is zero in either sampling session, this metric can still be calculated. Sites where no animals were caught in both sampling sessions were removed from the analyses.
To identify explanatory variables most correlated with changes in the response variables, we fitted GLMs in the program R version 4.3.1 (R Core Team 2023). Due to overdispersion in both response variables, quasi-binomial error structures were used. All explanatory variables were centred and standardised prior to analysis. Preliminary analysis identified a strong overriding effect of island as a categorical explanatory variable when combining data for both islands. As such, separate analyses were conducted for Bathurst and Melville Island data. Due to correlations between our explanatory variables, separate models which included each single explanatory variable were fit for each island. Modelled regression coefficients with 95% confidence intervals were plotted to evaluate the relative effect size of each variable on changes in native mammal abundance and richness.
Results
Live trapping
A total of 11 and 8 mammal species were recorded on Melville and Bathurst Islands, respectively. Two of these species have a mean body weight below the critical weight range: delicate mouse (Pseudomys delicatulus) and Butler’s dunnart. The introduced black rat (Rattus rattus) was not detected during surveys on either island, although it is known to occur in the small towns on both islands.
On Melville Island, the proportion of sites at which no native mammals were live trapped more than tripled over two decades, from 12% in 2000–2002 to 45% in 2021 (Fig. 2). There was also a significant reduction in both trap success and site-level species richness over this period. There was a 52% decline in overall trap success (3.76% ± 0.41 s.e. cf. 1.81% ± 0.28 s.e.; P < 0.001), and a 47% decline in site-level species richness (1.61 ± 0.1 s.e. cf. 0.86 ± 0.1 s.e., P < 0.001) (Fig. 2). Two of the four species recorded from at least 10% of sites in either sampling period showed a significant decrease in trap success: the northern brown bandicoot (Isoodon macrourus) decreasing by 72% (P < 0.001); and brush-tailed rabbit-rat (Conilurus penicillatus melibius) by 93% (P < 0.01) (Table S2; Fig. 3). Large (66–85%) decreases in naïve occupancy were also reported for these two species. Both the northern brushtail possum (Trichosurus vulpecula arnhemensis) and black-footed tree-rat (Mesembriomys gouldii melvillensis) showed non-significant declines in trap success. There was no change in northern brushtail possum naïve occupancy, whereas black-footed tree-rat naïve occupancy declined by 42%. The remaining seven species (mostly reported from too few sites or captures) showed no significant change in trap success (Table S2).
Total trap success, site-level species richness, and the proportion of sites with any mammal live-captures in 2000–2002 and 2020–2021 for Melville Island and Bathurst Island. Asterisks indicate a statistically significant change in trap success and species richness between sampling years (*P < 0.05).
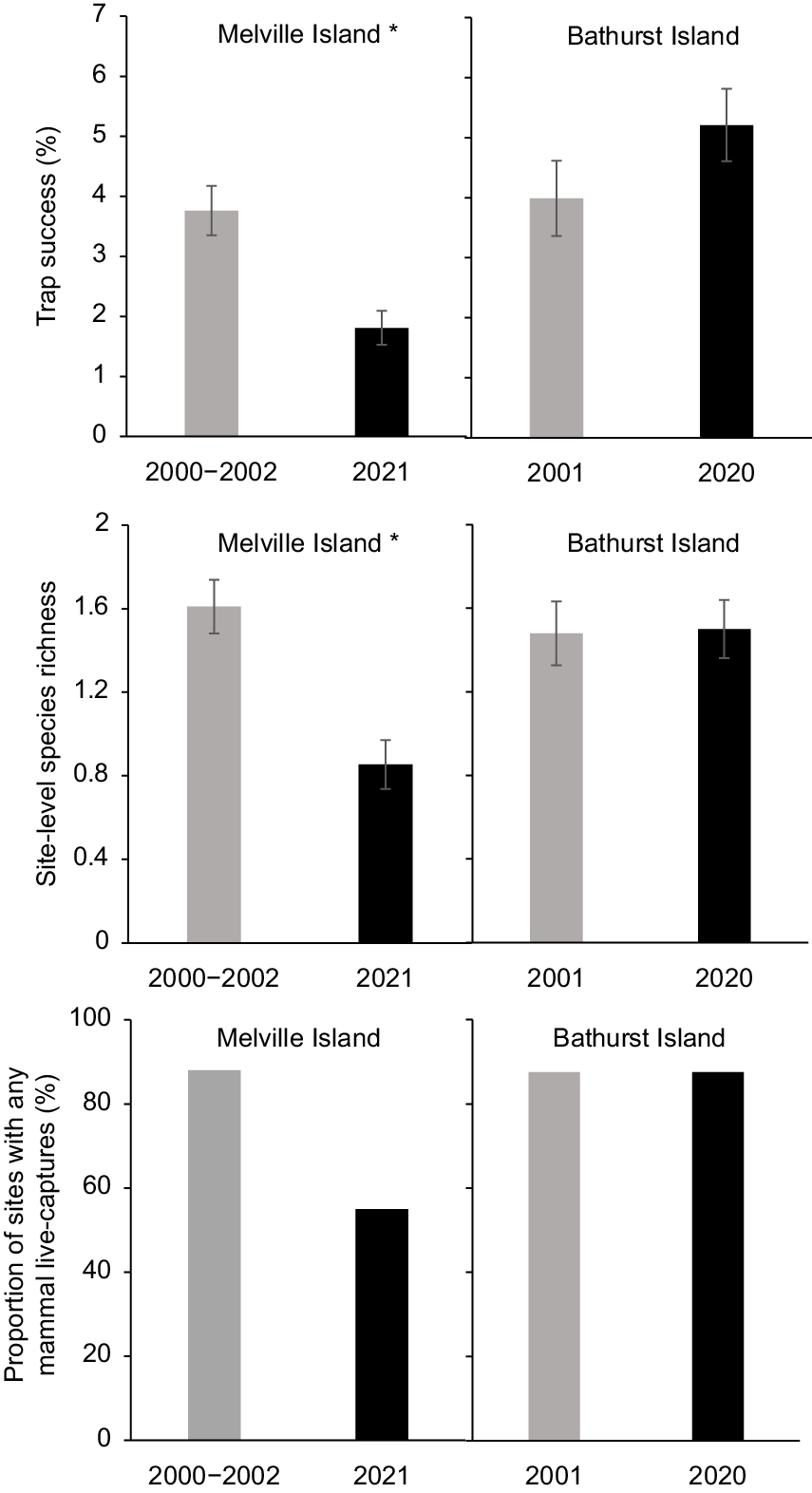
Brush-tailed rabbit-rat trap success and naïve occupancy in 2000–2002 and 2020–2021 for Melville Island and Bathurst Island. Asterisks indicate a statistically significant change in trap success between sampling years (**P < 0.005).
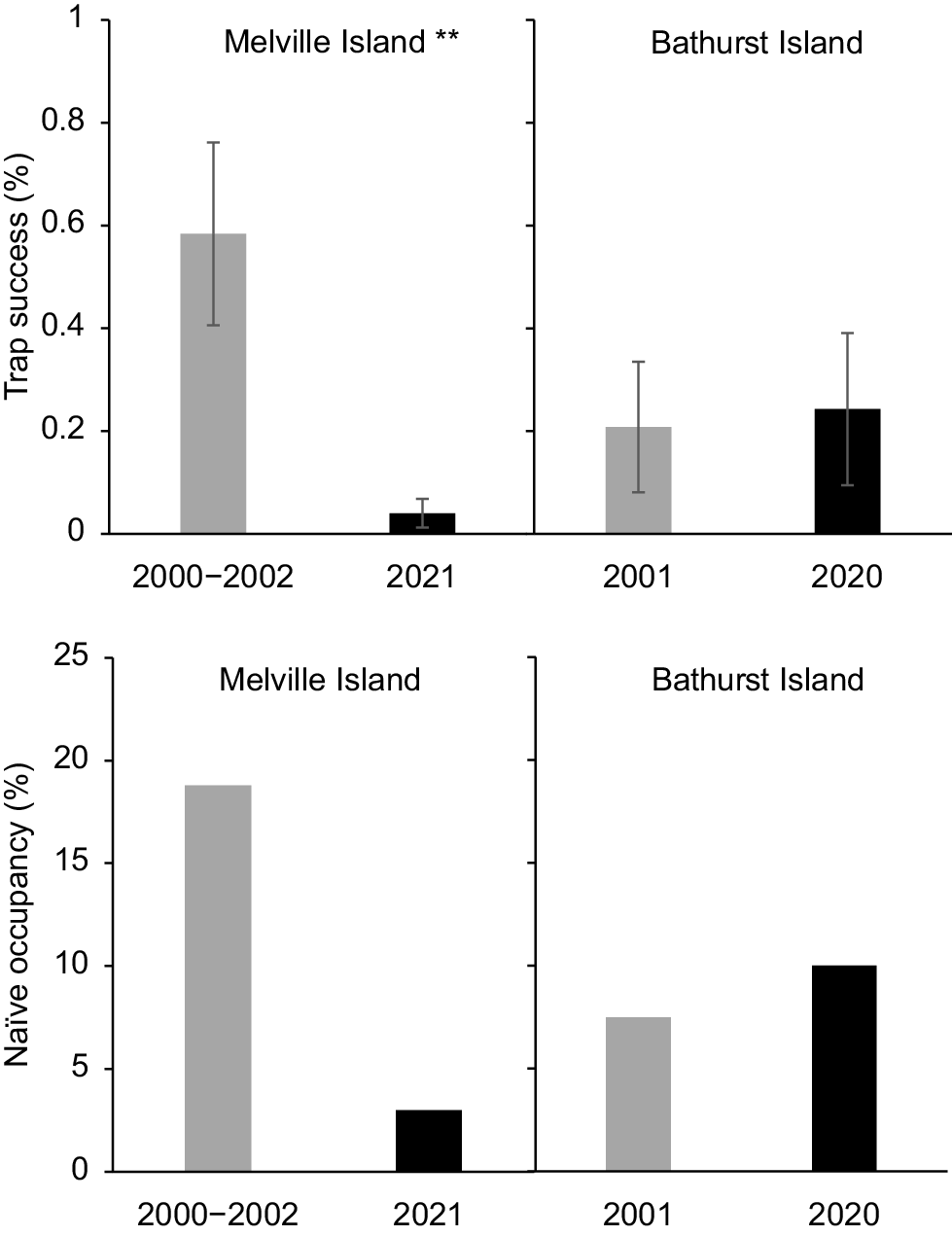
In marked contrast to Melville Island, there was no recorded decrease in the abundance or diversity of native mammals on Bathurst Island between 2001 and 2020. The proportion of sites at which no native mammals were trapped (12.5%) remained unchanged, and there was no statistically significant change in overall trap success or site-level species richness (Fig. 2). In fact, there was a slight, though not statistically significant, increase in overall trap success (3.99% ± 0.63 s.e. cf. 5.21% ± 0.60 s.e.; P = 0.138), and site-level species richness remained very similar (1.48 ± 0.15 s.e. cf. 1.50 ± 0.14 s.e., P = 0.991) (Fig. 2). Unlike in the 2001 surveys, the 2020 survey failed to live trap the grassland melomys (Melomys burtoni), pale field-rat (Rattus tunneyi) or western chestnutmouse (Pseudomys nanus); however, all three species were detected with camera traps (Table S3). Trap success for the northern brushtail possum more than tripled between 2001 (1.18% ± 0.364 s.e.; P < 0.001) and 2020 (3.58% ± 0.442 s.e.), while grassland melomys trap success dropped significantly from 0.72% ± 0.40 s.e. in 2001 to 0% in 2020 (P = 0.03). Trap success did not differ significantly between 2001 and 2020 for the northern brown bandicoot, brush-tailed rabbit-rat, western chestnut mouse, delicate mouse or pale field-rat (Table S3).
Camera trapping
In 2020 and 2021, the northern brushtail possum and northern brown bandicoot were the most widespread native species and were detected at 100% of sites on Bathurst Island, and at 97% and 87% of sites on Melville Island, respectively. The brush-tailed rabbit-rat was detected at 50% of sites on Bathurst and only 18% of sites on Melville Island. Of the remaining native species that occur on both islands, naïve occupancy rates were consistently higher on Bathurst Island, except for the pale field-rat (Tables S2 and S3). No buffalos or horses were recorded on Bathurst Island. Naïve occupancy and RAI for dingoes were more than twice as high on Melville compared with Bathurst Island, and were an order of magnitude higher for cats (Table 2). Pig naïve occupancy and RAI were far higher on Bathurst Island (Table 2).
Species | Naïve occupancy | P (Fisher’s test) | Mean RAI (±s.e.) | P (Wilcoxon’s test) | |||
---|---|---|---|---|---|---|---|
Melville | Bathurst | Melville | Bathurst | ||||
Buffalo | 60% | 0% | **** | 1.69 ± 0.26 | 0.00 ± 0.00 | NA | |
Horse | 33% | 0% | **** | 0.89 ± 0.28 | 0.00 ± 0.00 | NA | |
Dingo | 51% | 23% | ** | 0.66 ± 0.11 | 0.21 ± 0.07 | ** | |
Cat | 24% | 2% | ** | 0.25 ± 0.07 | 0.02 ± 0.01 | ** | |
Pig | 3% | 67% | **** | 0.02 ± 0.01 | 1.09 ± 0.27 | **** |
‘NA’ indicates species for which RAI could not be compared between islands, because the species were not detected on Bathurst Island (and is known to be absent).
Naïve occupancy was calculated as the proportion of sites where a species was detected. *P < 0.05, **P < 0.01, ***P < 0.001, ****P < 0.0001.
Correlates of change in mammal abundance and richness
On Melville Island, fire frequency and feral cat RAI were the only identified correlates of change in both mammal abundance and species richness (Fig. 4). Variables such as dingo, pig and exotic megaherbivore activity, and rainfall and proportion of surrounding area under plantation were not significant predictors of change. Models confirmed that mammal abundance and richness declined at sites where there was higher cat activity or that had burnt more frequently between sampling years (Figs 5 and 6). Of the explored fire metrics, fire frequency was the strongest predictor of change in mammal abundance and richness.
Model averaged regression coefficient estimates for (a) change in mammal abundance on Melville Island, (b) change in mammal species richness on Melville Island, (c) change in mammal abundance on Bathurst Island and (d) change in mammal species richness on Bathurst Island. Error bars represent 95% confidence intervals; asterisks indicate statistically significant effects, i.e. where they do not overlap zero.
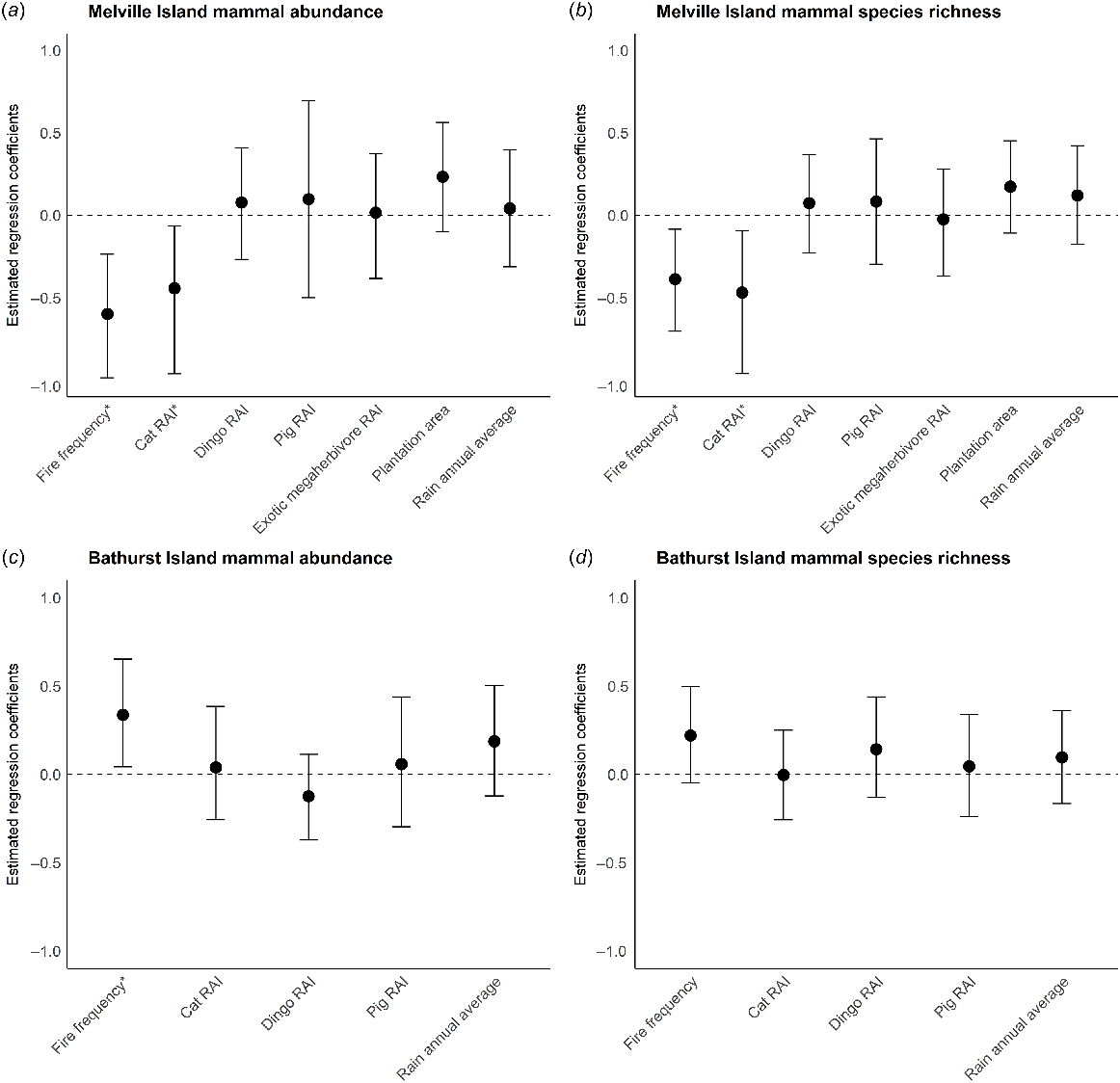
Modelled relationship between change in (a) mammal abundance and (b) mammal species richness and feral cat activity on Melville Island. The black lines represent the prediction of generalised linear models expressed as proportional change. Thin lines indicate the 95% confidence intervals of the regressions.
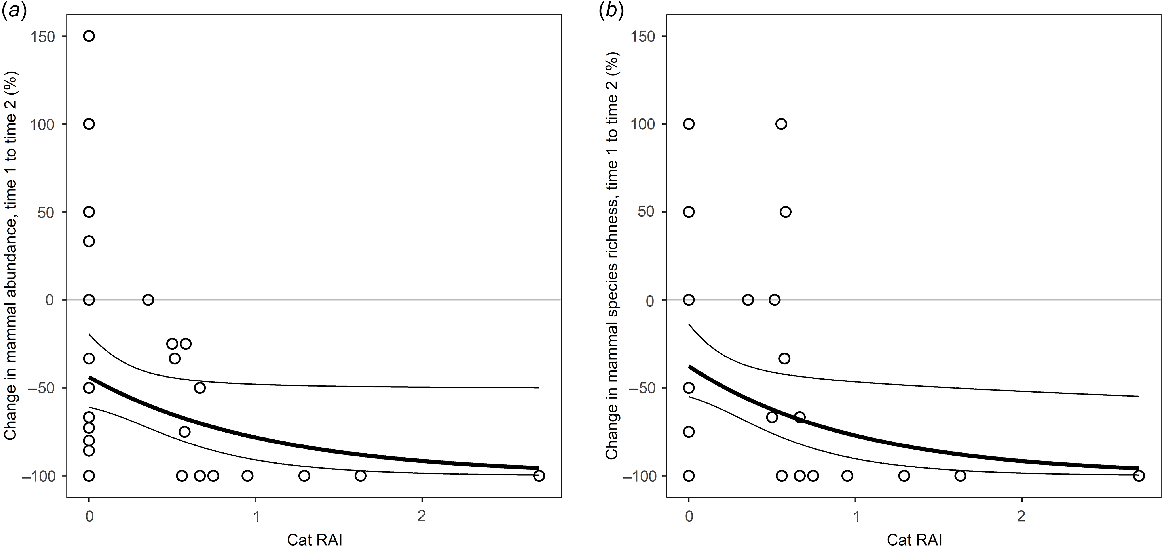
Modelled relationship between change in mammal abundance and species richness and fire frequency for (a) Melville Island and between mammal abundance and fire frequency for (b) Bathurst Island. The black lines represent the predictions of generalised linear models expressed as proportional change. Thin lines indicate the 95% confidence intervals of the regressions.
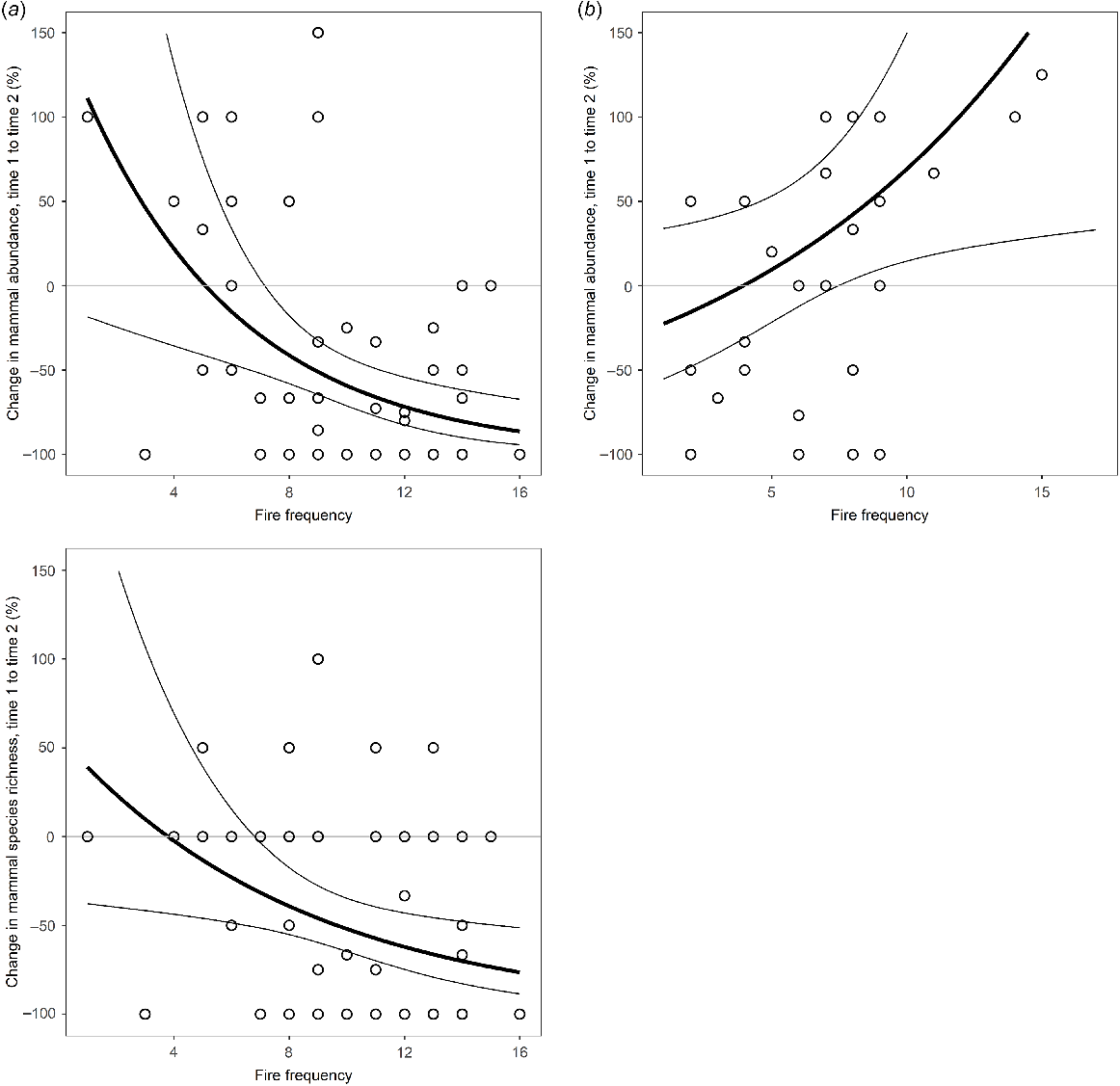
On Bathurst Island, fire frequency was a significant predictor of change in mammal abundance but not species richness (Fig. 4). Counter to Melville Island, mammal abundance increased at sites that had burnt more frequently during the years intervening the sampling periods (2002–2019).
Discussion
Since the precipitous decline of native mammals in monsoonal northern Australia was detected in recent decades (Firth et al. 2010; Woinarski et al. 2010; Stobo-Wilson et al. 2019), very few regions have retained an intact mammal assemblage. One such region is the Tiwi Islands, but we have shown that the two largest of these islands have markedly contrasting population trajectories. While there has been no apparent decline in mammals on Bathurst Island, our study has demonstrated a severe decline in site-level species richness and abundance of a range of mammals on Melville Island over a 20-year period. Overall trap success and species richness both declined significantly between 2000–2002 and 2021, and the proportion of sites where no native mammals were live trapped increased from 12% to 45%. These observations further support the mammal decline initially documented on Melville Island by Davies et al. (2018a). Worryingly, our study suggests a continuation of decline of the brush-tailed rabbit-rat on Melville Island, which has now exhibited a 93% decrease in trap success over the last 20 years. The percentage of sites where the brush-tailed rabbit-rat was detected decreased from 23% in 2015 (Davies et al. 2018a) to 18% in our study in 2021.
A strong inter-island difference was also evident for the correlates of change for native mammal abundance and species richness. On Melville Island, native mammal abundance and richness decreased most severely in areas that were frequently burnt and had a higher activity of feral cats. This strongly aligns with previous work that suggests frequent fire can exacerbate the impact of feral cats in northern Australian savannas (McGregor et al. 2014, 2015; Leahy et al. 2015). Importantly, native mammal abundance on Bathurst Island increased in frequently burnt areas. This again aligns with previous work on the Tiwi Islands suggesting that in areas with few feral cats, native mammal species can persist in, and potentially benefit from frequent fire (Davies et al. 2018b, 2022b). However, this is likely contingent on frequent fire being of low intensity. We found no evidence that mammal decline on Melville Island was associated with rainfall, plantation areas, dingo activity, pig activity or exotic megaherbivore activity.
Our results likely reflect the marked inter-island differences in the abundance of feral cats, with Melville having relatively high densities, similar to the mainland, and Bathurst having very low densities, rendering them virtually undetectable using camera traps (Davies et al. 2022a). Our study recorded only one detection of a feral cat from more than 10,000 camera trap nights, providing further evidence that feral cat density on Bathurst Island remains exceptionally low. This is a particularly intriguing finding due to the length of time cats have been present on the island coupled with the abundant mammalian prey. There are several factors that have potentially contributed to the observed differences in feral cat abundance between the two islands (Table S1). A plausible explanation is the higher severity of disturbance regimes on Melville is promoting higher cat densities by increasing hunting efficiency (McGregor et al. 2014, 2015; Leahy et al. 2015; Davies et al. 2020; Hradsky 2020). The fire regime of Melville and Bathurst Islands are mostly similar, with 41% vs 32% of each island burnt on average each year, respectively, with only a slightly higher proportion of fires occurring late in the dry season on Melville, when fire intensity tends to be higher. While a more detailed comparison of the fire regimes of each island could offer more insight, the stark difference in feral cat populations between the two islands is difficult to explain due to these differences alone.
A pronounced difference in the disturbance regimes of the two islands is the high density of buffalo on Melville, compared to their absence from Bathurst. In northern Australia, feral cats have been reported to be in lower abundance and density in areas with fewer or no feral megaherbivores, including on Melville Island (Davies et al. 2020; Heiniger et al. 2020). However, the mechanisms behind this association remain poorly understood. Grazing of ‘green pick’ by megaherbivores may exacerbate the impact of fire on small mammals by extending the length of time the ground layer vegetation – which can provide cover from predators – is suppressed (Legge et al. 2019). As with fire, grazing can also cause longer-term impacts on vegetation such as simplifying habitat by removing the shrub layer (Vigilante and Bowman 2004), reducing perennial native grass abundance (Russell-Smith et al. 2003; Orr and O’Reagain 2011), also potentially benefiting predators (Hradsky 2020). Another possibility is that the abundant ‘game trails’ created by megaherbivores enable cats (and other predators, such as dingoes) to move efficiently through the landscape. While this effect of megaherbivores on savanna predators has not been investigated, linear features such as tracks and roads benefit wolves and other predators elsewhere in the world (Dickie et al. 2017, 2022; DeMars and Boutin 2018). However, this prior research largely relates to increased hunting efficiency, and we acknowledge that much uncertainty remains regarding the population-level effects of these mechanisms, especially in isolation of each other. If buffalo are a key facilitator of cat populations on Melville Island, we may have expected native mammals to have suffered more severe and earlier declines given buffalo have been widespread and abundant on Melville Island for almost 200 years.
Understanding what limits cat abundance on Bathurst Island, or conversely, what promotes cat abundance on Melville Island, remains a key research question with potentially important implications for Australian conservation. Progressing our mechanistic understanding will lead to increasingly informed and effective management actions aimed at conserving Australia’s native mammal populations. Currently, we also need to ensure areas that have so far remained less degraded (Tiwi Islands, Groote Eylandt, parts of the northern Kimberley) are maintained in their relatively intact state, given there has been little demonstrated recovery of mammal populations following management interventions in highly degraded areas. For example, mammals continued to decline in Kakadu National Park after broadscale exotic megaherbivore control as part of the Bovine Brucellosis and Tuberculosis Eradication Campaign (BTEC; Woinarski et al. 2010). The lack of mammal recovery following BTEC could be interpreted as evidence against the role of megaherbivores in the northern Australian mammal decline. However, it should also be noted that the effects of megaherbivore removal on feral cat populations may not be readily apparent given lag effects or hysteresis, especially in the absence of a specifically designed and optimised monitoring program to detect mammal recovery in response to BTEC.
In future, to better understand the predominant pathways through which predators impact northern Australian mammals, we suggest a broader focus on the predator assemblage beyond feral cats. In this paper, we have focused on cats as the primary driver of small mammal decline on Melville Island. However, our study also reported higher abundances of another mammalian predator on Melville Island: dingo. Widely considered a ‘native’ species, dingoes have been present in Australia for at least 3500 years (Fillios and Taçon 2016) and presumably have also been on the Tiwi Islands for a similar length of time. Their protracted presence alongside sympatric small-medium sized mammal species sets the depredation risk by dingoes apart from those of other more recently introduced predators. For example, since their introduction ca. 200 years ago, cats have been implicated as the primary cause of extinction for at least 20 fauna species and responsible for the ongoing declines of many others (Dickman 1996; Abbott et al. 2014; Woinarski et al. 2015; Stobo-Wilson et al. 2022). It is also possible that the relatively severe disturbance regimes observed on Melville Island (Table S1) could also facilitate dingo predation through mechanisms similar to those discussed for cats. This interaction was previously suggested by Stobo-Wilson et al. (2020b), which reported higher occurrences of dingoes in areas with reduced habitat structural complexity— often resulting from abundant exotic megaherbivores and frequent, high-severity fires. Therefore, we would expect that if intensifying disturbance regimes facilitate increased dingo abundance on Melville Island, predation pressure on dingo prey would also increase. Our study did not detect a strong effect of site-level dingo activity on changes in mammal abundance or richness on either island. However, detailed information on dingo and cat densities in a region coupled with diet analysis and prey availability is lacking and more research is needed to quantify exact differences in predation rates between these predators. The impact of dingoes on small mammal species is likely to be more limited than that of cats, as dingoes tend to exhibit a stronger preference for medium to large-sized (≥5 kg) mammals (Corbett 2001; Brook and Kutt 2011; Stokeld et al. 2018; Doherty et al. 2019), such as the agile wallaby (Notamacropus agilis), which is both widespread and abundant on the Tiwi Islands (G. Neave, unpublished data). In contrast, feral cats consistently demonstrate significantly higher predation rates on small mammals as evidenced by dietary studies conducted across Australia (Kutt 2012; Doherty et al. 2015; Stokeld et al. 2018; Murphy et al. 2019; Woolley et al. 2019).
There are potential limitations associated with inferring mammal population change from two surveys conducted two decades apart that warrant discussion regarding the validity of our conclusions. First, there can be difficulties when interpreting a decrease in trap success as a decrease in the abundance of a species. For example, although not statistically significant, we recorded a 32% decrease in northern brown bandicoot trap success on Bathurst Island. Rather than interpreting this as a decrease in abundance, we strongly suspect that it is a sampling artefact caused by a saturation of available cage traps by the northern brushtail possum, whose trap success tripled. Importantly, no species showed a significant increase in trap success on Melville Island, so trap saturation was not a contributing factor to the recorded decrease in overall trap success. Such methodological limitations highlight the care needed when interpreting changes in live trapping success as true changes in abundance. Our live trapping surveys did not detect the grassland melomys, pale field-rat and western chestnut mouse on Bathurst Island in 2020, despite these species being detected in 2001. However, given that these species were all rarely detected in 2001, there is little inference that can be drawn from not detecting them in 2020. Additionally, while our analyses of the correlates of change for mammal abundance and richness provided additional inference and facilitated further discussion of the potential explanations for the inter-island differences, it is important to note that these remain speculative. For example, we acknowledge that the use of overall abundance metrics could be biased by only a few species, and that our analyses were limited by the lack of detailed information on how some explanatory variables have changed over time in addition to the possible interactive effects of these variables.
Another limitation of our study is that native mammal populations can exhibit large inter-annual fluctuations in abundance, potentially making it difficult to differentiate between natural fluctuations and ongoing population decline, especially with sparse temporal data. However, given the similarities between the geographic and taxonomic patterns of mammal decline on Melville Island and those recorded across the northern Australian mainland over the past 30 years (Woinarski et al. 2001, 2010, 2011a; Ziembicki et al. 2015), we can confidently discount the possibility that the declines recorded on Melville Island reflect natural fluctuations in abundance. For example, as first identified by Davies et al. (2018a), mammal decline on the Melville Island is most pronounced for species that have suffered the earliest and most severe declines on the mainland, such as the brush-tailed rabbit-rat which has contracted from areas of the island with high feral cat and exotic megaherbivore activity (Davies et al. 2017; Penton et al. 2021). We provided further evidence that this species has continued to decline on Melville Island between 2015 and 2021.
Despite the above limitations, it is clear that Bathurst Island remains as a nationally significant refuge for critical weight range mammals, including for the brush-tailed rabbit-rat, which is included in the list of Australia’s 20 mammals most likely to go extinct by 2038 (Geyle et al. 2018). We detected this species at 50% of sites in 2020, making it likely to be Australia’s largest remaining stable population. We also recorded a substantial increase in trap success for the northern brushtail possum on Bathurst Island, which tripled between 2001 and 2020. This is despite it suffering widespread decline across northern Australia (Woinarski et al. 2001, 2010; Stobo-Wilson et al. 2019; Campbell et al. 2022), and being listed as Vulnerable under Australian legislation. It is unlikely that this dramatic increase in brushtail possum trap success can be attributed to any substantial reductions in the threat milieu on Bathurst Island. No large-scale management of pig, cat or dingo populations has been undertaken in the last two decades. Analysis of fire regimes shows a slight shift in fire seasonality with an overall decline in late dry season fires, although this is not significant. Interestingly, the high rates of brushtail possum trap success reported in 2020 are supported by high RAI derived from camera trapping at the same sites (G. Neave, unpublished data). Indeed, the mean RAI for brushtail possums on Bathurst was 2.5 times the mean RAI of brushtail possums on neighbouring Melville Island; as such, we can be confident that possums are in very high abundance on Bathurst Island. Whilst the 3-fold increase in brushtail possum trap success between 2001 and 2020 could reflect natural population fluctuations to some degree, it could also be that this rate of increase has been amplified due to differences in the probability of live trapping between sampling sessions. In both sessions, sampling was undertaken in the dry season; however, in 2001, all surveys were completed in the early dry season (May–June) and in 2020, surveys were conducted in the late dry season (July–September). Large differences in the probability of detection with live trapping in the early versus the late dry season have been reported for semi-arboreal species, such as the brush-tailed rabbit-rat (Geyle et al. 2019) and for brushtail possums (H. Davies, unpublished data) on the Tiwi Islands. For some species, live trapping data is more sensitive to changes in animal behaviour linked to resource shortages, and without historical camera data from the 2001 surveys, we can only speculate that seasonality is a contributing factor in exaggerating the increase in possum trap success.
Naïve occupancy rates, derived from camera trapping, for northern brown bandicoots, northern brushtail possums, black-footed tree-rats and brush-tailed rabbit-rats on the Tiwi Islands were much higher compared to sites in lowland woodland savanna across mainland Northern Territory (Heiniger et al. 2020). Therefore, it is important to note that compared to most areas of mainland northern Australia, both Melville and Bathurst Island currently support regionally significant populations of native mammals. The Tiwi Islands receive the highest rainfall totals in the Northern Territory and contain its tallest and highest-biomass eucalypt open forests (Woinarski et al. 2003). The high productivity of these islands has likely contributed to the persistence of native mammals via heightened resource-driven population resilience (Stobo-Wilson et al. 2020a). Given the presence of threatening factors (exotic mammals and frequent fire), it is plausible that the declines starting to become apparent across the less-productive areas of Melville Island could extend to the more productive Bathurst Island sometime in the future. Therefore, regardless of their current healthy state, we warn against complacency based on the assumption that native mammal populations on Bathurst Island will remain safe from future decline. To ensure the persistence of native mammals on the Tiwi Islands we need the effective management of both fire and feral animals, coupled with ongoing monitoring across both islands.
Our study is an important addition to the large existing body of work investigating the drivers of the northern Australia mammal declines. Using the Tiwi Islands as a natural laboratory to compare the impact of their different disturbance regimes, we have provided important insights into the links between feral cats, and disturbance regimes as facilitators of cats in these ecosystems. This is more difficult in other parts of Australia where the implicated drivers are ubiquitous and mammal populations already depauperate. While our study has highlighted the critical role of islands for both biodiversity conservation and applied ecological studies, it has also highlighted the complexity and uncertainty over the potential facilitators of feral cats on Melville Island. In particular, we recognise that the role of megaherbivores as agents of disturbance that can benefit cats has been understudied compared to fire. The Tiwi Islands currently remain a unique refuge for native mammals. Their large size affords protection to resident populations from stochastic events that can extirpate populations on small refugial islands or isolated areas (Woinarski et al. 2011b; Alzate et al. 2019; Fernández-Palacios et al. 2021). Sadly, the current patterns of mammal decline on Melville Island are all too familiar across northern Australia, and the continent more broadly. Yet, a silver lining of this familiarity is that we now find ourselves with a sufficient understanding of the drivers of mammal decline to effectively mitigate, or at least attempt, to mitigate these declines. However, if nothing is done to prioritise management actions that reduce the severity of the key threats on the Tiwi Islands, we reiterate the warnings widely touted in many ecological studies from northern Australia. Additional species will be driven to extinction, and the ramifications of this will be far wider than the loss of biodiversity; it will include irreversible impacts on Tiwi culture.
Data availability
The data used in this publication is owned by the Tiwi Land Council. Access to this data can be discussed with the Tiwi Land Council (office@tiwilandcouncil.com), and the Northern Territory Government for sampling years 2000–2002.
Declaration of funding
This research was supported by funding from the Australian Government through the Australian Research Council (LP170100305) and the Indigenous Land and Sea Council (ILSC).
Author contributions
G.N., B.P.M. and H.F.D. conceived the ideas and designed methodology; G.N., H.F.D. and Tiwi Rangers collected and collated the data; G.N., B.P.M. and H.F.D. analysed the data; G.N. led the writing of the manuscript; B.P.M., H.F.D. and A.N.A. manuscript review and editing, and supervision; B.P.M. funding acquisition. All authors contributed critically to the drafts and gave final approval for publication.
Acknowledgements
We would first like to thank the Tiwi Land Council and Tiwi Traditional Owners from the eight land owning groups (Malawu, Mantiyupwi, Munupi, Marrikawuyanga, Jikilaruwu, Wurankuwu, Wulirankuwu and Yimpinari) for their ongoing support of biodiversity research on their land and their ongoing collaboration with Charles Darwin University. Many people were involved in providing valuable field assistance to this project. We would like to recognise the Tiwi Land Rangers: Colin Kerinaiua, the late S. Austral, Marbine Austral and Derek Puruntatameri and volunteers: Toby Barton, Alys Young, Teigan Cremona, Jo McCormack, Tess O’Reilly, Eridani Mulder, Daniel Costa, Ange Trnka, Eric Andersen, Paris O’Rourke, Brenton von Takach and Nicola Hanrahan for their contribution. A very special thanks to the late Willie Rioli Snr, whose commitment to caring for country and unwavering support of this work will never be forgotten. We recognise also the contributions of Martin Armstrong, Kym Brennan, Ronald Firth, Alaric Fisher, Jenni Low Choy, Simon Ward and John Woinarski to the 2000–2002 sampling and the Northern Territory Government for making this data available.
References
Abbott I (2008) The spread of the cat, Felis catus, in Australia: re-examination of the current conceptual model with additional information. Conservation Science Western Australia 7, 1-17.
| Google Scholar |
Alzate A, Etienne RS, Bonte D (2019) Experimental island biogeography demonstrates the importance of island size and dispersal for the adaptation to novel habitats. Global Ecology and Biogeography 28, 238-247.
| Crossref | Google Scholar |
Andersen AN, Woinarski JCZ, Parr CL (2012) Savanna burning for biodiversity: fire management for faunal conservation in Australian tropical savannas: savanna burning for faunal diversity. Austral Ecology 37, 658-667.
| Crossref | Google Scholar |
Bayliss P, Bayliss P, Yeomans KM, Yeomans KM (1989) Distribution and abundance of feral livestock in the ‘Top End’ of the Northern Territory (1985-86), and their relation to population control. Wildlife Research 16, 651-676.
| Crossref | Google Scholar |
Beery S, Morris D, Yang S (2019) Efficient pipeline for camera trap image review. Available at http://arxiv.org/abs/1907.06772 [Accessed 4 December 2022]
Brook LA, Kutt AS (2011) The diet of the dingo (Canis lupus dingo) in north-eastern Australia with comments on its conservation implications. The Rangeland Journal 33, 79-85.
| Crossref | Google Scholar |
Burbidge AA, McKenzie NL (1989) Patterns in the modern decline of western Australia’s vertebrate fauna: causes and conservation implications. Biological Conservation 50, 143-198.
| Crossref | Google Scholar |
Campbell BL, Gallagher RV, Ens EJ (2022) Expanding the biocultural benefits of species distribution modelling with Indigenous collaborators: case study from northern Australia. Biological Conservation 274, 109656.
| Crossref | Google Scholar |
Darwin Centre for Bushfire Research (2023) North Australia and Rangelands Fire Information. Available at http://www.firenorth.org.au/nafi3/ [Verified 18 July 2024]
Davies HF, McCarthy MA, Firth RSC, Woinarski JCZ, Gillespie GR, Andersen AN, Geyle HM, Nicholson E, Murphy BP (2017) Top-down control of species distributions: feral cats driving the regional extinction of a threatened rodent in northern Australia. Diversity and Distributions 23, 272-283.
| Crossref | Google Scholar |
Davies HF, McCarthy MA, Firth RSC, Woinarski JCZ, Gillespie GR, Andersen AN, Rioli W, Puruntatameri J, Roberts W, Kerinaiua C, Kerinauia V, Womatakimi KB, Murphy BP (2018a) Declining populations in one of the last refuges for threatened mammal species in northern Australia. Austral Ecology 43, 602-612.
| Crossref | Google Scholar |
Davies HF, McCarthy MA, Rioli W, Puruntatameri J, Roberts W, Kerinaiua C, Kerinauia V, Womatakimi KB, Andersen AN, Murphy BP (2018b) An experimental test of whether pyrodiversity promotes mammal diversity in a northern Australian savanna. Journal of Applied Ecology 55, 2124-2134.
| Crossref | Google Scholar |
Davies HF, Maier SW, Murphy BP (2020) Feral cats are more abundant under severe disturbance regimes in an Australian tropical savanna. Wildlife Research 47, 624-632.
| Crossref | Google Scholar |
Davies HF, Rangers TL, Rees MW, Stokeld D, Miller AC, Gillespie GR, Murphy BP (2022a) Variation in feral cat density between two large adjacent islands in Australia’s monsoon tropics. Pacific Conservation Biology 28, 18-24.
| Crossref | Google Scholar |
Davies HF, Rangers TL, Nicholson E, Murphy BP (2022b) Northern brown bandicoot (Isoodon macrourus) and common brushtail possum (Trichosurus vulpecula) density on the Tiwi Islands: insights and implications. Pacific Conservation Biology 28, 224-230.
| Crossref | Google Scholar |
DeMars CA, Boutin S (2018) Nowhere to hide: effects of linear features on predator–prey dynamics in a large mammal system. Journal of Animal Ecology 87, 274-284.
| Crossref | Google Scholar | PubMed |
Dickie M, Serrouya R, McNay RS, Boutin S (2017) Faster and farther: wolf movement on linear features and implications for hunting behaviour. Journal of Applied Ecology 54, 253-263.
| Crossref | Google Scholar |
Dickie M, Serrouya R, Avgar T, McLoughlin P, McNay RS, DeMars C, Boutin S, Ford AT (2022) Resource exploitation efficiency collapses the home range of an apex predator. Ecology 103, e3642.
| Crossref | Google Scholar |
Dickman CR (1996) Impact of exotic generalist predators on the native fauna of Australia. Wildlife Biology 2, 185-195.
| Crossref | Google Scholar |
Doherty TS, Davis RA, van Etten EJB, Algar D, Collier N, Dickman CR, Edwards G, Masters P, Palmer R, Robinson S (2015) A continental-scale analysis of feral cat diet in Australia. Journal of Biogeography 42, 964-975.
| Crossref | Google Scholar |
Doherty TS, Davis NE, Dickman CR, Forsyth DM, Letnic M, Nimmo DG, Palmer R, Ritchie EG, Benshemesh J, Edwards G, Lawrence J, Lumsden L, Pascoe C, Sharp A, Stokeld D, Myers C, Story G, Story P, Triggs B, Venosta M, Wysong M, Newsome TM (2019) Continental patterns in the diet of a top predator: Australia’s dingo. Mammal Review 49, 31-44.
| Crossref | Google Scholar |
Doody JS, Green B, Rhind D, Castellano CM, Sims R, Robinson T (2009) Population-level declines in Australian predators caused by an invasive species. Animal Conservation 12, 46-53.
| Crossref | Google Scholar |
Fernández-Palacios JM, Kreft H, Irl SDH, Norder S, Ah-Peng C, Borges PAV, Burns KC, de Nascimento L, Meyer J-Y, Montes E, Drake DR (2021) Scientists’ warning – the outstanding biodiversity of islands is in peril. Global Ecology and Conservation 31, e01847.
| Crossref | Google Scholar | PubMed |
Fillios MA, Taçon PSC (2016) Who let the dogs in? A review of the recent genetic evidence for the introduction of the dingo to Australia and implications for the movement of people. Journal of Archaeological Science: Reports 7, 782-792.
| Crossref | Google Scholar |
Firth RSC, Woinarski JCZ, Brennan KG, Hempel C (2006) Environmental relationships of the brush-tailed rabbit-rat, Conilurus penicillatus, and other small mammals on the Tiwi Islands, northern Australia. Journal of Biogeography 33, 1820-1837.
| Crossref | Google Scholar |
Firth RSC, Brook BW, Woinarski JCZ, Fordham DA (2010) Decline and likely extinction of a northern Australian native rodent, the Brush-tailed Rabbit-rat Conilurus penicillatus. Biological Conservation 143, 1193-1201.
| Crossref | Google Scholar |
Fisher DO, Johnson CN, Lawes MJ, Fritz SA, McCallum H, Blomberg SP, VanDerWal J, Abbott B, Frank A, Legge S, Letnic M, Thomas CR, Fisher A, Gordon IJ, Kutt A (2014) The current decline of tropical marsupials in Australia: is history repeating? Global Ecology and Biogeography 23, 181-190.
| Crossref | Google Scholar |
Frank ASK, Johnson CN, Potts JM, Fisher A, Lawes MJ, Woinarski JCZ, Tuft K, Radford IJ, Gordon IJ, Collis M-A, Legge S (2014) Experimental evidence that feral cats cause local extirpation of small mammals in Australia’s tropical savannas. Journal of Applied Ecology 51, 1486-1493.
| Crossref | Google Scholar |
Geyle HM, Woinarski JCZ, Baker GB, Dickman CR, Dutson G, Fisher DO, Ford H, Holdsworth M, Jones ME, Kutt A, Legge S, Leiper I, Loyn R, Murphy BP, Menkhorst P, Reside AE, Ritchie EG, Roberts FE, Tingley R, Garnett ST (2018) Quantifying extinction risk and forecasting the number of impending Australian bird and mammal extinctions. Pacific Conservation Biology 24, 157.
| Crossref | Google Scholar |
Geyle HM, Guillera-Arroita G, Davies HF, Firth RSC, Murphy BP, Nimmo DG, Ritchie EG, Woinarski JCZ, Nicholson E (2019) Towards meaningful monitoring: a case study of a threatened rodent. Austral Ecology 44, 223-236.
| Crossref | Google Scholar |
Greenberg S (2020) Timelapse: an image analyser for camera traps (version 2.2.3.6). Available at https://www.saul.cpsc.ucalgary.ca/timelapse/ [Accessed 10 April 2022]
Griffiths AD, Garnett ST, Brook BW (2015) Fire frequency matters more than fire size: testing the pyrodiversity–biodiversity paradigm for at-risk small mammals in an Australian tropical savanna. Biological Conservation 186, 337-346.
| Crossref | Google Scholar |
Heiniger J, Gillespie G (2018) High variation in camera trap-model sensitivity for surveying mammal species in northern Australia. Wildlife Research 45, 578-585.
| Crossref | Google Scholar |
Heiniger J, Davies HF, Gillespie GR (2020) Status of mammals on Groote Eylandt: safe haven or slow burn? Austral Ecology 45, 759-772.
| Crossref | Google Scholar |
Hradsky BA (2020) Conserving Australia’s threatened native mammals in predator-invaded, fire-prone landscapes. Wildlife Research 47, 1-15.
| Crossref | Google Scholar |
Kutt AS (2012) Feral cat (Felis catus) prey size and selectivity in north-eastern Australia: implications for mammal conservation: feral cat prey size and selection. Journal of Zoology 287, 292-300.
| Crossref | Google Scholar |
Kutt AS, Woinarski JCZ (2007) The effects of grazing and fire on vegetation and the vertebrate assemblage in a tropical savanna woodland in north-eastern Australia. Journal of Tropical Ecology 23, 95-106.
| Crossref | Google Scholar |
Lawes MJ, Murphy BP, Fisher A, Woinarski JCZ, Edwards AC, Russell-Smith J (2015) Small mammals decline with increasing fire extent in northern Australia: evidence from long-term monitoring in Kakadu National Park. International Journal of Wildland Fire 24, 712-722.
| Crossref | Google Scholar |
Leahy L, Legge SM, Tuft K, McGregor HW, Barmuta LA, Jones ME, Johnson CN (2015) Amplified predation after fire suppresses rodent populations in Australia’s tropical savannas. Wildlife Research 42, 705-716.
| Crossref | Google Scholar |
Legge S, Kennedy MS, Lloyd R, Murphy SA, Fisher A (2011) Rapid recovery of mammal fauna in the central Kimberley, northern Australia, following the removal of introduced herbivores: recovery of tropical savanna mammals. Austral Ecology 36, 791-799.
| Crossref | Google Scholar |
Legge S, Smith JG, James A, Tuft KD, Webb T, Woinarski JCZ (2019) Interactions among threats affect conservation management outcomes: livestock grazing removes the benefits of fire management for small mammals in Australian tropical savannas. Conservation Science and Practice 1, e52.
| Crossref | Google Scholar |
McGregor HW, Legge S, Jones ME, Johnson CN (2014) Landscape management of fire and grazing regimes alters the fine-scale habitat utilisation by feral cats. PLoS ONE 9, e109097.
| Crossref | Google Scholar | PubMed |
McGregor H, Legge S, Jones ME, Johnson CN (2015) Feral cats are better killers in open habitats, revealed by animal-borne video. PLoS ONE 10, e0133915.
| Crossref | Google Scholar | PubMed |
Murphy BP, Davies HF (2014) There is a critical weight range for Australia’s declining tropical mammals: critical weight range for Australia’s declining tropical mammals. Global Ecology and Biogeography 23, 1058-1061.
| Crossref | Google Scholar |
Murphy BP, Woolley L-A, Geyle HM, Legge SM, Palmer R, Dickman CR, Augusteyn J, Brown SC, Comer S, Doherty TS, Eager C, Edwards G, Fordham DA, Harley D, McDonald PJ, McGregor H, Moseby KE, Myers C, Read J, Riley J, Stokeld D, Trewella GJ, Turpin JM, Woinarski JCZ (2019) Introduced cats (Felis catus) eating a continental fauna: the number of mammals killed in Australia. Biological Conservation 237, 28-40.
| Crossref | Google Scholar |
O’Brien TG, Kinnaird MF, Wibisono HT (2003) Crouching tigers, hidden prey: Sumatran tiger and prey populations in a tropical forest landscape. Animal Conservation 6, 131-139.
| Crossref | Google Scholar |
Orr DM, O’Reagain PJ (2011) Managing for rainfall variability: impacts of grazing strategies on perennial grass dynamics in a dry tropical savanna. The Rangeland Journal 33, 209-220.
| Crossref | Google Scholar |
Pardon LG, Brook BW, Griffiths AD, Braithwaite RW (2003) Determinants of survival for the northern brown bandicoot under a landscape-scale fire experiment. Journal of Animal Ecology 72, 106-115.
| Crossref | Google Scholar |
Penton CE, Davies HF, Radford IJ, Woolley L-A, Rangers TL, Murphy BP (2021) A hollow argument: understory vegetation and disturbance determine abundance of hollow-dependent mammals in an Australian tropical savanna. Frontiers in Ecology and Evolution 9, 739550.
| Crossref | Google Scholar |
Potter LC, Brady CJ, Murphy BP (2019) Accuracy of identifications of mammal species from camera trap images: a northern Australian case study. Austral Ecology 44, 473-483.
| Crossref | Google Scholar |
Radford IJ, Gibson LA, Corey B, Carnes K, Fairman R (2015) Influence of fire mosaics, habitat characteristics and cattle disturbance on mammals in fire-prone savanna landscapes of the northern Kimberley. PLoS ONE 10, e0130721.
| Crossref | Google Scholar | PubMed |
Radford IJ, Corey B, Carnes K, Shedley E, McCaw L, Woolley L-A (2021) Landscape-scale effects of fire, cats, and feral livestock on threatened savanna mammals: unburnt habitat matters more than pyrodiversity. Frontiers in Ecology and Evolution 9, 739817.
| Crossref | Google Scholar |
R Core Team (2023) ‘R: a language and environment for statistical computing. R version 4.3.1.’ (R Foundation for Statistical Computing: Vienna, Austria) Available at https://www.R-project.org/
Russell-Smith J, Whitehead PJ, Cook GD, Hoare JL (2003) Response of eucalyptus-dominated savanna to frequent fires: lessons from Munmarlary, 1973–1996. Ecological Monographs 73, 349-375.
| Crossref | Google Scholar |
Shine R (2010) The ecological impact of invasive cane toads (Bufo marinus) in Australia. The Quarterly Review of Biology 85, 253-291.
| Crossref | Google Scholar | PubMed |
Start AN, Burbidge AA, McKenzie NL, Palmer C (2007) The status of mammals in the North Kimberley, Western Australia. Australian Mammalogy 29, 1-16.
| Crossref | Google Scholar |
Stobo-Wilson A (2018) Ecology of the savanna glider (Petaurus ariel) in tropical northern Australia. PhD Thesis, Charles Darwin University, Darwin. doi:10.25913/5EA9028A3A782
Stobo-Wilson AM, Murphy BP, Cremona T, Carthew SM (2019) Contrasting patterns of decline in two arboreal marsupials from Northern Australia. Biodiversity and Conservation 28, 2951-2965.
| Crossref | Google Scholar |
Stobo-Wilson AM, Stokeld D, Einoder LD, Davies HF, Fisher A, Hill BM, Mahney T, Murphy BP, Scroggie MP, Stevens A, Woinarski JCZ, Bawinanga Rangers, Warddeken Rangers, Gillespie GR (2020a) Bottom-up and top-down processes influence contemporary patterns of mammal species richness in Australia’s monsoonal tropics. Biological Conservation 247, 108638.
| Crossref | Google Scholar |
Stobo-Wilson AM, Stokeld D, Einoder LD, Davies HF, Fisher A, Hill BM, Mahney T, Murphy BP, Stevens A, Woinarski JCZ, Rangers B, Warddeken Rangers, Gillespie GR (2020b) Habitat structural complexity explains patterns of feral cat and dingo occurrence in monsoonal Australia. Diversity and Distributions 26, 832-842.
| Crossref | Google Scholar |
Stobo-Wilson AM, Murphy BP, Legge SM, Caceres-Escobar H, Chapple DG, Crawford HM, Dawson SJ, Dickman CR, Doherty TS, Fleming PA, Garnett ST, Gentle M, Newsome TM, Palmer R, Rees MW, Ritchie EG, Speed J, Stuart J-M, Suarez-Castro AF, Thompson E, Tulloch A, Turpin JM, Woinarski JCZ (2022) Counting the bodies: estimating the numbers and spatial variation of Australian reptiles, birds and mammals killed by two invasive mesopredators. Diversity and Distributions 28, 976-991.
| Crossref | Google Scholar |
Stokeld D, Fisher A, Gentles T, Hill B, Triggs B, Woinarski JCZ, Gillespie GR (2018) What do predator diets tell us about mammal declines in Kakadu National Park? Wildlife Research 45, 92-101.
| Crossref | Google Scholar |
Vigilante T, Bowman DMJS (2004) Effects of fire history on the structure and floristic composition of woody vegetation around Kalumburu, North Kimberley, Australia: a landscape-scale natural experiment. Australian Journal of Botany 52, 381-404.
| Crossref | Google Scholar |
von Takach B, Scheele BC, Moore H, Murphy BP, Banks SC (2020) Patterns of niche contraction identify vital refuge areas for declining mammals. Diversity and Distributions 26, 1467-1482.
| Crossref | Google Scholar |
Woinarski JCZ, Milne DJ, Wanganeen G (2001) Changes in mammal populations in relatively intact landscapes of Kakadu National Park, Northern Territory, Australia. Austral Ecology 26, 360-370.
| Crossref | Google Scholar |
Woinarski J, Oakwood M, Winter J, Burnett S, Milne D, Foster P, Myles H, Holmes B (2008) Surviving the toads: patterns of persistence of the northern quoll Dasyurus hallucatus in Queensland. Report to the Australian Government’s natural Heritage Trust, Canberra. doi:10.13140/RG.2.2.24203.41767
Woinarski JCZ, Armstrong M, Brennan K, Fisher A, Griffiths AD, Hill B, Milne DJ, Palmer C, Ward S, Watson M, Winderlich S, Young S (2010) Monitoring indicates rapid and severe decline of native small mammals in Kakadu National Park, northern Australia. Wildlife Research 37, 116-126.
| Crossref | Google Scholar |
Woinarski JCZ, Legge S, Fitzsimons JA, Traill BJ, Burbidge AA, Fisher A, Firth RSC, Gordon IJ, Griffiths AD, Johnson CN, McKenzie NL, Palmer C, Radford I, Rankmore B, Ritchie EG, Ward S, Ziembicki M (2011a) The disappearing mammal fauna of northern Australia: context, cause, and response. Conservation Letters 4, 192-201.
| Crossref | Google Scholar |
Woinarski JCZ, Ward S, Mahney T, Bradley J, Brennan K, Ziembicki M, Fisher A (2011b) The mammal fauna of the Sir Edward Pellew island group, Northern Territory, Australia: refuge and death-trap. Wildlife Research 38, 307-322.
| Crossref | Google Scholar |
Woinarski J, Burbidge A, Harrison P (2014) ‘Action plan for Australian mammals 2012.’ (CSIRO Publishing: Melbourne, Vic, Australia) doi:10.1071/9780643108745
Woinarski JCZ, Burbidge AA, Harrison PL (2015) Ongoing unraveling of a continental fauna: decline and extinction of Australian mammals since European settlement. Proceedings of the National Academy of Sciences 112, 4531-4540.
| Crossref | Google Scholar |
Woolley L-A, Geyle HM, Murphy BP, Legge SM, Palmer R, Dickman CR, Augusteyn J, Comer S, Doherty TS, Eager C, Edwards G, Harley DKP, Leiper I, McDonald PJ, McGregor HW, Moseby KE, Myers C, Read JL, Riley J, Stokeld D, Turpin JM, Woinarski JCZ (2019) Introduced cats Felis catus eating a continental fauna: inventory and traits of Australian mammal species killed. Mammal Review 49, 354-368.
| Crossref | Google Scholar |
Ziembicki MR, Woinarski JCZ, Mackey B (2013) Evaluating the status of species using Indigenous knowledge: novel evidence for major native mammal declines in northern Australia. Biological Conservation 157, 78-92.
| Crossref | Google Scholar |
Ziembicki MR, Woinarski JCZ, Webb JK, Vanderduys E, Tuft K, Smith J, Ritchie EG, Reardon TB, Radford IJ, Preece N, Perry J, Murphy BP, McGregor H, Legge S, Leahy L, Lawes MJ, Kanowski J, Johnson CN, James A, Griffiths AD, Gillespie G, Frank ASK, Fisher A, Burbidge AA (2015) Stemming the tide: progress towards resolving the causes of decline and implementing management responses for the disappearing mammal fauna of northern Australia. Therya 6, 169-226.
| Crossref | Google Scholar |