Diet of fallow deer suggests potential for invasion of novel habitats in Tasmania
Thomas R. Guy


A
B
C
D
Abstract
Understanding the diet of invasive species can inform the potential for their distribution into novel habitats. Fallow deer are well established in the grassy woodlands of central Tasmania, Australia, in environments generally considered to be their optimum habitat. They are also increasing their range. The potential range of fallow deer in Tasmania will depend on their ability to vary their diet to exploit new habitats. Diet flexibility will also determine the ecological impacts that fallow deer might have in novel habitats.
We compared the diets of fallow deer in a lowland grassy woodland, where deer have been established for over 150 years, with diets of deer in highland woodlands and forest with less grass cover and higher rainfall, where deer have been established for a shorter time (<50 years). We expected that fallow deer in grassy woodlands would mainly eat grass and forbs, and we wanted to know to what extent the diet of deer differed between habitats.
A metagenomic analysis was performed on fallow deer faecal pellets collected at one lowland and three highland study areas. The method was chosen to maximise information on taxonomic composition of diet and identify plant species that might be affected by deer herbivory to the lowest possible taxonomic level.
Fallow deer ate a wide variety of plant taxa. Diets varied among study areas. In the lowland study area, deer predominantly ate forbs and grasses. In the highland study area deer were more likely to browse on eucalypts and a variety of shrubs.
Fallow deer in Tasmania have a broad dietary niche. Availability of specific plant taxa is unlikely to limit fallow deer expansion into most new habitats.
Without stronger management strategies, deer are likely to further increase their range in Tasmania, including into areas with high conservation values. The potential impacts on these areas may be high.
Keywords: Australia, browsing impacts, Dama dama, Eucalyptus, fallow deer, feeding ecology, invasive species, metagenomics, Tasmania, ungulates.
Introduction
Large herbivores have strong effects on the structure and composition of vegetation in many ecosystems (Burkepile and Parker 2017). Information on the diets of large herbivores is essential to understand and manage their impacts on vegetation (Holechek et al. 1982). This information is especially important in forecasting the diversity of habitats that might be occupied by invasive large herbivores and anticipating the potential impacts of those species on native biodiversity (Clavel et al. 2011; Slatyer et al. 2013). A flexible diet should allow herbivores to invade a wide range of habitats and to have significant impacts on vegetation in novel habitats (Lurgi et al. 2014).
Deer are among the most successful of invasive large herbivores worldwide (Genovesi 2005; Chakanya et al. 2016). The European Fallow deer (Dama dama dama) originally occurred in a small part of Anatolia (Masseti et al. 2008; Wilson and Mittermeier 2011). It is now one of the most widely distributed cervids globally (Chapman and Chapman 1980), having established populations in much of western and southern Europe (Genovesi 2005) as well as North America, New Zealand, South Africa and Australia (Chapman and Chapman 1980; Chakanya et al. 2016; Esattore et al. 2022). The preferred habitat of fallow deer is a mosaic of grassland and forest with a grassy understorey (Chapman and Chapman 1975). The species is categorised as an intermediate feeder, consuming both grass and browse (Chapman and Chapman 1975; Kerridge and Bullock 1991; Spitzer et al. 2020). Although its food preferences may be wide (Esattore et al. 2022), it generally shows a higher preference for grasses (Chapman and Chapman 1975; Hofmann 1989), especially during the growing season (Spitzer et al. 2020).
Fallow deer were introduced to Tasmania (and to other parts of Australia) in the early nineteenth century (Bentley 1998; Hall and Gill 2005). The initial introductions of deer in Tasmania were to grassy woodlands in the low-elevation, warm and dry Midlands of Tasmania. These habitats resemble the preferred habitats of fallow deer elsewhere in their global geographic range. Initially, the Tasmanian population grew slowly. By the 1970s, its size was estimated to be ~8000 animals (Chapman and Chapman 1975). Since the mid-1980s, however, the population has been increasing at 11.5% per year and extending its distribution such that it currently occurs over 27% of Tasmania (Cunningham et al. 2022), including areas with high conservation value such as the Tasmanian Wilderness World Heritage Area (TWWHA). A 2019 aerial survey estimated the population in the eastern part of the state to be ~53 000 (Lethbridge et al. 2020). Cunningham et al. (2022) suggest that because the survey was conducted late in the 2019 hunting season, during which approximately 30 000 deer were killed (Lethbridge et al. 2020), the population size was at least ~83 000 at its 2019 seasonal peak.
Very little is known about the diet of fallow deer in Tasmania, or elsewhere in Australia. Rumen-content analysis of a population in New South Wales, Australia found that grass ranged from 99% of diet composition in agricultural areas to 66% in more densely wooded areas, the balance of the diet consisting of browse (Calleja 2001). Davis et al. (2023) also found that fallow deer ranged from a grazing to a browsing diet that included eucalypts. In Tasmania, Duncan (1992) used histological analysis of faecal material to estimate dietary overlap of eastern grey kangaroos (Macropus giganteus) and fallow deer in the Midlands of Tasmania. Both species consumed grasses as the main component of their diet. However, kangaroos had a higher proportion of grasses in their diet than fallow deer (61% versus 46%), but fallow deer ate a greater diversity of plant species overall, including a higher proportion of shrub species.
In the absence of management intervention, such as the 2023 aerial deer cull in the TWWHA (Powell 2023), fallow deer are predicted to further expand their abundance and distribution in Tasmania; there are large areas of the island with apparently suitable climate and habitat but which are not yet occupied (Potts et al. 2015; Cunningham et al. 2022). The portions of Tasmania that are not currently occupied by fallow deer include areas with high conservation value, which support many vegetation types that differ from the grassy woodlands of the Midlands. In ungulates in general, behaviours related to habitat selection are largely driven by satisfying dietary needs (Spitzer et al. 2020). Therefore, the degree to which the Tasmanian fallow deer population will be able to increase its distribution will depend partly on its ability to broaden its dietary niche so that it can occupy a wider range of habitats, especially those that differ from the grassy woodlands in which deer currently occur.
In this study, we aimed to describe variation in diet of fallow deer in Tasmania by comparing diets of deer in grassy woodlands in the dry lowlands of Tasmania with those of deer in highland woodlands and forest with a cooler and wetter climate. Our grassy woodlands study area is typical of areas in which fallow deer have been well established in Tasmania since the nineteenth century (Bentley 1957) and represents habitat that has been generally considered to be preferred by the species (Chapman and Chapman 1975). The cooler highland study areas have more varied and dense vegetation with less grass and a higher representation and diversity of woody species. These study areas are typical of environments into which fallow deer have spread more recently (Wapstra 1973).
We expected that in the lowland grassy woodlands, deer would eat mostly grasses and forbs; our study was designed to test the extent to which the diet differed in contrasting high-elevation habitats, especially by including a higher diversity of plant species and increased browsing on woody vegetation.
We described diet by metagenomic analysis of faecal material. The metagenomic approach was chosen to maximise information on the taxonomic composition of the diet, identified to the finest possible level, and to identify taxa that might be strongly affected by herbivory in the highlands. Metagenomic analysis provides more highly resolved information on herbivore diets than traditional methods such as histological examination of faecal material (Pompanon et al. 2012), and has been used successfully in previous analysis of faecal material in deer (Czernik et al. 2013; Nakahara et al. 2015; Erickson et al. 2017).
Materials and methods
Study area
We investigated diets of fallow deer at four study areas in Tasmania, one (Beaufront) in the low-lying Midlands and three (Five Rivers, Silver Plains and Arthurs Lake) in highland areas in central Tasmania (Fig. 1). Beaufront is a private grazing property. Five Rivers and Silver Plains are reserves owned and managed by the Tasmanian Land Conservancy. Arthurs Lake is within the Great Lake Conservation Area and managed by the Tasmanian Parks and Wildlife Service. Beaufront is at an elevation of ~ 300 m ASL (above sea level), and its climate is characterised by low rainfall (mean annual 489 mm) and moderate temperatures (mean annual daily minima and maxima 5.6°C and 17.7°C respectively). The three highland study areas are 800–1000 m ASL, with climates that are wetter (mean annual rainfall 840–940 mm) and cooler (mean daily minima 1.6–3.4°C, maxima 10.3–13.9°C).
Location of study areas (black circles) used for faecal pellet collection. Black line depicts the Tasmanian Wilderness World Heritage Area. Red line depicts the distribution of fallow deer in central Tasmania from Cunningham et al. (2022). Satellite populations are not shown on map.
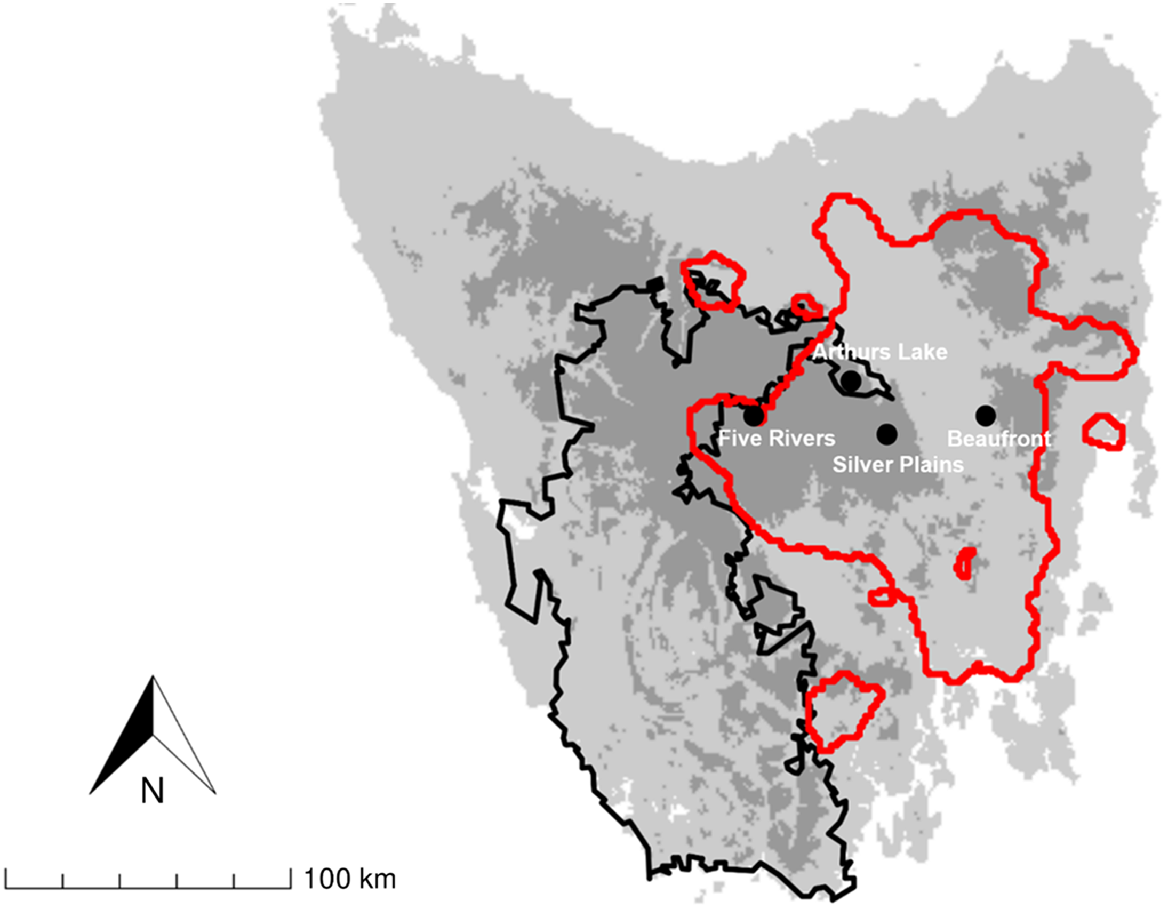
The vegetation on Beaufront is a mixture of open grasslands (used to graze sheep and cattle) and remnant open eucalypt woodland. We sampled in an area of the property that was predominantly open woodland over unimproved pasture dominated by native species. The canopy tree species were widely spaced white gum (Eucalyptus viminalis) and black peppermint (E. amygdalina) over a sparse and patchy mid-storey of silver wattle (Acacia dealbata), silver banksia (Banksia marginata) and black she-oak (Allocasuarina littoralis). The ground level vegetation was dominated by native grasses (kangaroo grass (Themeda triandra), tussock grasses (Poa spp.), spear grasses (Austrostipa spp.) and wallaby grasses (Rytidosperma spp.)), some introduced grasses and a high diversity of forbs, both native and introduced. Dense patches of sagg (Lomandra longifolia) and bracken fern (Pteridium esculentum) also occurred in places. The vegetation communities as defined by Kitchener and Harris (2013) were Eucalyptus viminalis grassy forest and woodland (DVG) within a mosaic of lowland grassland complex (GCL) and Lowland Poa labillardierei grassland (GPL).
The three high-elevation study areas consisted of forested areas dominated by gum-topped stringybark (E. delegatensis), cabbage gum (E. pauciflora) and swamp peppermint (E. rodwayi), growing over a dense mid-storey of shrubs dominated by small-fruit hakea (Hakea microcarpa). These forested areas were variously interspersed in some places by patches of alpine and sub-alpine heath, most commonly dominated by mountain pinkberry (Leptecophylla parvifolia), as well as coral heath (Epacris gunnii) and lowland richea (Richea procera), and in other places by tussock grasslands dominated by Poa and Rytidosperma species. These grasslands also contained a variety of both native (Cranesbill species (Geranium spp.), raspwort species (Gonocarpus spp.), button weeds (Leptinella spp.)) and introduced forbs (Hypochaeris radicata, Leontodon saxatilis). The vegetation communities represented in these high-elevation study areas were Eucalyptus delegatensis dry forest and woodland (DDE), Eucalyptus rodwayi forest and woodland (DRO), Eucalyptus pauciflora forest and woodland on dolerite (DPD) and Eucalyptus pauciflora forest and woodland not on dolerite (DPO), interspersed with Highland Poa grassland (GPH), Highland grassy sedgeland (MGH), Eastern alpine sedgeland (HSE) and Eastern alpine heathland (HHE) (Kitchener and Harris 2013).
Sample collection
Ten fallow deer faecal pellets were collected from each of the four study areas. Samples were taken during winter 2020 (August) except at Beaufront, which, due to limited access caused by heavy rains, was sampled during early spring (September) 2020. Each faecal pellet was collected from a fresh deposit, as judged by a dark brown shiny appearance. Samples were collected at a minimum of 1 km apart, to decrease the likelihood of collecting samples from the same animal. Samples were stored in 50-mL Falcon tubes on ice and transferred to a freezer at the end of each day.
Reference samples of plant material were collected for 30 plant species identified at the study areas but not represented in the nucleotide database NCBI (GenBank) (Supplementary Table S2). Plant species identifications were confirmed by the Tasmanian Herbarium. A single leaf, or leaf cutting approximately 20 mm in length, was taken from each of the plants. Samples were stored in the same manner as the faecal pellets. Faecal pellet and plant samples were sent frozen to eDNA Frontiers for metagenomic analysis.
Metagenomic analysis
Subsamples were taken from the middle of each scat. DNA was extracted from each subsample using a modified Qiagen DNeasy PowerSoil Pro IRT protocol (Qiagen, Germany) and an automated QIAcube extraction platform (Qiagen). DNA was also extracted manually from taxonomically identified plant specimens using a Qiagen DNeasy Plant Pro kit. All extractions were undertaken in a dedicated PCR-free laboratory, and extraction controls processed alongside samples. Extractions were eluted in a final volume of 100 μL AE buffer.
To sequence the dietary plant DNA, a new assay was selected (rbcL_uni_F: 5′-CGTTACAAAGGRMGATGC-3′; rbcL_uni_R – 5′-CATTCATAAACHGCTCTACC-3′). This assay (Tm – 49°C) amplifies a 341-bp region of the chloroplast rbcL gene and has been used successfully in house (eDNA Frontiers) to detect and identify a wide variety of terrestrial and marine plants. To determine the required dilution for optimal amplification of scat samples, PCR reactions were performed in duplicate on each extraction by adding DNA template directly to the PCR master mix (neat), then performing two serial dilutions (1 in 10, and 1 in 100). The PCRs were performed at a final volume of 25 μL, where each reaction consisted of: 1× PCR Gold Buffer (Applied Biosystems), 0.25 mM dNTP mix (Astral Scientific, Australia), 2 mM MgCl2 (Applied Biosystems), 1 U AmpliTaq Gold DNA polymerase (Applied Biosystems), 0.4 mg/mL bovine serum albumin (Fisher Biotec), 0.4 μM forward and reverse primers, 0.6 μL of a 1:10 000 solution of SYBR Green dye (Life Technologies), and 2 μL template DNA. PCRs were performed on StepOne Plus instruments (Applied Biosystems) with the following cycling conditions: 95°C for 5 min, followed by 50 cycles of: 95°C for 30 s, 49°C for 30 s, 72°C for 45 s, then a melt-curve analysis of: 95°C for 15 s, 60°C for 1 min, 95°C for 15 s, finishing with a final extension stage at 72°C for 10 min.
After selection of the optimal dilution (neat, 1 in 10, or 1 in 100), PCRs were repeated in duplicate as described above, with the addition of unique, single use combinations of 8 bp multiplex identifier-tagged (MID-tag) primers as described in Koziol et al. (2019) and van der Heyde et al. (2020). Master mixes were prepared using a QIAgility instrument (Qiagen) in an ultra-clean lab facility, with negative and positive PCR controls included on every plate to ensure the validity of results. A sequencing library was created by combining samples into mini-pools based on the PCR amplification results from each sample. The mini-pools were analysed using a QIAxcel (Qiagen) fragment analyser and combined in roughly equimolar concentrations to form a library. The library was then size selected (250–600 bp cut-off) using a Pippin Prep instrument (Sage Sciences) with 2% dye-free cassettes, cleaned using a QIAquick PCR purification kit, quantified on a Qubit (Thermo Fisher) and diluted to 2 nM. The library was sequenced on an Illumina MiSeq instrument using a 500-v2 cycle kit with custom sequencing primers.
DNA from plant specimens was amplified at neat concentration with PCRs performed at a final volume of 25 μL, where each reaction comprised of: 1× Platinum Green PCR MM (Invitrogen), 0.2 μM forward and reverse primers (rbcLa-F and rbcLa-R; (Kress and Erickson 2007)), and 2 μL template DNA. PCRs were performed on StepOne Plus instruments with the following cycling conditions: 94°C for 3 min, followed by 35 cycles of: 94°C for 1 min, 54°C for 1 min, 72°C for 1 min, finishing with a final extension stage at 72°C for 10 min. Each PCR reaction was checked on an agarose gel to confirm positive amplification, then sent to the Australian Genome Research Facility (AGRF) for dual-direction sequencing.
Raw metabarcoding sequence data were analysed using the eDNAFlow pipeline (Mousavi-Derazmahalleh et al. 2021) with custom filtering parameters applied (−minAlignLeng ‘12’, −minLen ‘150’, −minsize ‘2’, perc_identity ‘95’, −qcov ‘100’, −minMatch_lulu ‘97’). Generated ZOTUs were queried against the nucleotide database NCBI (GenBank), as well as a custom database generated from plant specimens, and assigned to the species level where possible or dropped back to the lowest common ancestor if multiple possible taxonomic assignments were given. Data were further curated by removing taxa that were unlikely to be a match (i.e. not found in the study area), allowing for additional resolution in taxonomic assignments. Taxa above family level were excluded from the analysis.
Statistical analysis
Metabarcoding matches that were at a coarser taxonomic level than family were excluded from the analysis. All other matches are reported to the lowest possible taxonomic level.
We used global non-metric multidimensional scaling (NMDS) to ordinate the presence/absence data for the 31 most abundant taxa (five or more faecal pellets). The ordination procedure followed the default options in DECODA. Vectors of maximum correlation for the species in Table 1 were fitted to the ordination space in DECODA. Probabilities were calculated using 10 000 iterations.
Study areas | ||||||||
---|---|---|---|---|---|---|---|---|
Variable | AL | FR | B | SP | Mean | χ2 value | Significance P | |
Gonocarpus tetragynus | 100 | 60 | 0 | 40 | 50 | 20.8 | 0.000 | |
Eucalyptus spp. | 90 | 60 | 10 | 80 | 60 | 15.83 | 0.001 | |
Cerastium spp. | 100 | 100 | 50 | 100 | 87.5 | 17.14 | 0.001 | |
Oxalis spp. | 0 | 0 | 40 | 0 | 10 | 13.33 | 0.004 | |
Asteraceae spp. | 30 | 20 | 80 | 20 | 37.5 | 10.56 | 0.014 |
Degrees of freedom = 3.
B, Beaufront; SP, Silver Plains Reserve; AL, Arthurs Lake; FR, Five Rivers Reserve.
Analysis of similarity (ANOSIM) was performed in DECODA to assess the strength and significance of dissimilarity of plant taxa eaten among the four study areas, the input being the presence/absence of taxa in each scat. The reported R-value reveals the degree of dissimilarity among study areas relative to dissimilarity in diets of individuals within study areas, with a value of 1 indicating that taxa consumed within each study area were completely distinct from one other, and a value of 0 indicating no dissimilarity among sites.
Chi-squared tests were performed to test the difference among study areas in the frequency of taxa with expected values for cells of five or more.
Results
Fallow deer consumed a wide range of plant species across all study areas. This included representatives of bryophytes (5% of samples), ferns (7.5%), orchids (2.5%) forbs (87.5%), grasses (30%), sedges (35%), rushes (17.5%), shrubs (50%) and trees (70%). Aquatic plant taxa were present in 10% of samples. Both introduced and native plant species were eaten. In all, 52 genera from 30 families were detected. Forty-four taxa were identified to species level (Supplementary Table S1). The most common taxa were Asteraceae at the family level (37.5% of all scats), Eucalyptus (60%) at the genus level and Gonocarpus tetragynus (50%) at the species level. The shrub small-fruit Hakea (Hakea microcarpa) was detected in 15% of faecal pellet samples (Supplementary Table S1).
The composition of the diet varied strongly between the low-elevation Midlands study area and the highland study areas, which were variable but overlapping with one another in the NMDS ordination space (Fig. 2). The only significant dissimilarity among study areas in ordination scores were between Beaufront and the other three study areas (ANOSIM, Beaufront versus Arthurs Lake R = 0.4404, P < 0.001; Beaufront versus Five Rivers R = 0.3300, P < 0.001; Beaufront versus Silver Plains, R = 0.3593, P < 0.001). The taxa most responsible for causing the differences in positions in the ordination space were the genera Oxalis, Cerastium and Eucalyptus, and the Family Asteraceae (Fig. 2). The first ordination axis was characterised by a shift from high representation of forbs (Asteraceae, Oxalis and Cerastium) at one end, to trees (Eucalyptus) and subshrubs (Gonocarpus) at the other (Fig. 2). The three Central Plateau study areas had significantly more scats with the tree genus Eucalyptus and the subshrub Gonocarpus tetragynus (Table 1).
Non-metric multidimensional scaling (NMDS) ordination plot of community dissimilarities based on the plant species found in deer faecal pellets (2D stress value = 0.214). The shorter the distance between two samples, the higher the similarity between the scats. Significant (P < 0.05) vectors of maximum correlation are shown.
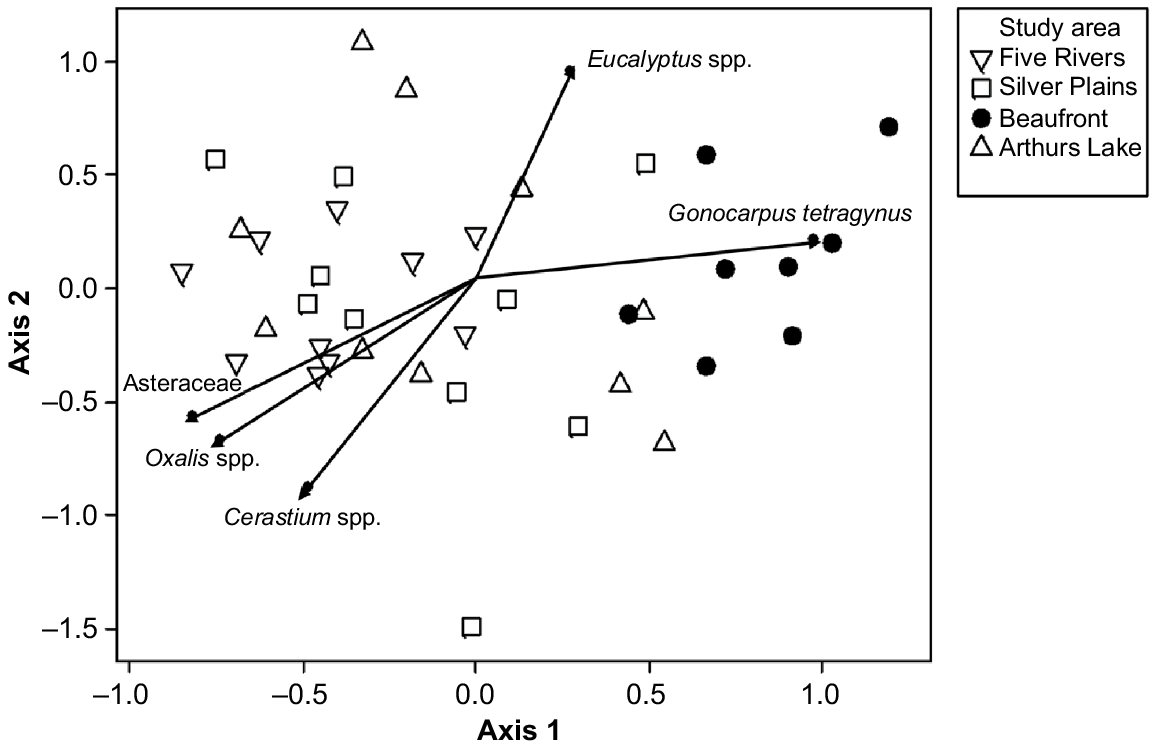
Fig. 3 summarises diet composition in the four study areas in terms of major plant life forms. This shows that at Beaufront, herbs were most commonly recorded in scats, and trees were relatively uncommon; trees and shrubs were more commonly recorded in scats at the highland sites, especially at Silver Plains and Arthurs Lake, and at one highland site (Arthurs Lake) grass was not detected (Fig. 3).
Discussion
Most studies of fallow deer diet have been conducted in Europe, followed by North and South America (Esattore et al. 2022). Our results provide replication at a finer taxonomic resolution for the small number of published Australian studies (Duncan 1992; Calleja 2001; Davis et al. 2023). We confirm that fallow deer has a wide dietary niche, consuming plants from a broad range of taxa and growth forms. We also show that there are large differences in diets of deer occupying contrasting habitats in Tasmania. This versatility of feeding ecology should mean that fallow deer will be able to occupy a broad range of vegetation types in Tasmania, shifting their diets locally in response to availability of different kinds of plants. Because of this versatility, deer appear capable of substantially extending their current distribution in Tasmania to large areas of the north and the south-west of the island (Cunningham et al. 2022). The broad dietary niche of fallow deer also means they could have significant impacts on vegetation communities in those regions.
The taxonomically wide diet of deer in Tasmania is consistent with a global literature that demonstrates that, although typically a grazer (Chapman and Chapman 1975; Hofmann 1989), fallow deer are versatile in their dietary choices (Chapman and Chapman 1975; Jackson 1977). The species can shift its diet in response to availability of different kinds of forage in different habitats or seasons (Spitzer et al. 2020; Esattore et al. 2022). For example, in the Czech Republic, under heavy snow cover, fallow deer switch from a diet of brambles and grasses to one of mostly low-quality spruce needles (Kamler and Homolka 2011). The differences in diet that we observed reflect availability according to vegetation structure. In the open grassy woodlands of the low-elevation woodlands, the scats of fallow deer contained predominantly forb and grass taxa, but in more densely wooded highland habitats with less grass and more heath or shrub vegetation in the understorey, the scats had a high proportion of shrub and tree taxa. We studied diet at the end of the winter, when plant growth (and therefore forage availability) was likely to be at an annual low point, especially for grasses and herbs. It is therefore likely that feeding on grasses would have increased during the warmer months, as has been observed in Italy (Bruno and Apollonio 1991).
Much of Tasmania is within the range of climate tolerance of fallow deer, with 56% of Tasmania estimated as highly suitable (Cunningham et al. 2022). However, it is possible that deer would have greater difficulty surviving in the oligotrophic environments of western Tasmania, where grazing animals are extremely sparse. Fallow deer in Tasmania thus have the potential to have negative effects on many plant species and distinctive vegetation communities. For example, several species of ancient endemic conifers, such as pencil pine (Athrotaxis cupressoides) occur in the Tasmanian highlands. Athrotaxis cupressoides is a slow growing species (Worth et al. 2017) and has been historically reduced by browsing by marsupial herbivores interacting with fire (Kirkpatrick and Bridle 2007; Holz et al. 2015). The grassy ecosystems of eastern and central Tasmania contain a high proportion of threatened taxa, including orchids, daisies, grasses and sedges (Quarmby et al. 2023). All of these types of plants were represented in the diet of Tasmanian deer.
The fact that Eucalyptus species were commonly eaten by fallow deer is concerning because Eucalypt woodlands are in decline across much of the lowland range of fallow deer. Eucalypt woodlands in the Tasmanian Midlands are already threatened (Kirkpatrick et al. 1995; Cowell et al. 2013) and in decline (Neyland 1996; Prior et al. 2013). Attempts to restore woodland patches in the Midlands (Davidson et al. 2021) are severely hampered by deer browsing. For example, Bailey et al. (2015) observed that 45% of Eucalyptus tenuiramis trees used for a restoration planting project in Tasmania were damaged by fallow deer after 3.25 years, and they cited damage from fallow deer as a significant impediment to cost-effective restoration.
In the higher elevation study areas of the Central Plateau, Eucalyptus species may experience even higher browsing pressure from fallow deer. Impacts on some species are potentially significant. For example, the Tasmanian endemic Eucalyptus gunnii has suffered increased dieback over the last decades, attributed to increased droughts associated with a warming climate (Calder and Kirkpatrick 2008). However, Calder and Kirkpatrick (2008) demonstrate that the most severe dieback is also related to heavy possum browsing pressure. The endangered E. gunnii subsp. divaricata is restricted to the eastern Central Plateau, in proximity to the Silver Plains and Arthurs Lake study areas used for sampling in the present paper, where our results show high prevalence of browsing on Eucalyptus species.
Of the taxa discovered in the diet, 14 were introduced species. Most of these species are common pasture weeds e.g. Alopecurus pratensis, Cirsium palustre, Leontodon saxatilis. The Midlands study area had the highest proportion of introduced species in the diet. This is most likely to be related to the higher level of disturbance in the landscape than in the higher elevation study areas. Plant germinants emerging from fallow deer scat are 57% greater per scat than those emerging from kangaroo scat (Claridge et al. 2016). Considering the wide dietary preferences demonstrated in this paper, it is likely that deer will act as weed vectors as they continue to colonise currently unoccupied environments.
Fallow deer have been shown to consume ferns, e.g. Dryopteris filix-mas and Pteridium sp. (Jackson 1977; Kerridge and Bullock 1991), although research that has quantified the proportions of plant species in the diet suggests that ferns are only consumed in low amounts, such as <1% (Davis et al. 2023), 5% (Jackson 1977) and 10% (Trabalza Marinucci et al. 2010). We report an even lower proportion, with each of the three species of fern detected being found in one deer scat each.
In New Zealand, at a similar latitude to temperate Australia, Nugent (1990) concluded that browsing by fallow deer in beech forests would inhibit regeneration of subcanopy tree species when densities of deer exceed 10 deer per km2. Fallow deer have a preference for young seedlings and coppiced trees in Australia (Hall and Gill 2005). Given the New Zealand and Australian observations, it is likely that browsing by fallow deer could limit recruitment of woody plants in some Tasmanian plant communities, especially given a potential for the populations to grow (Potts et al. 2015; Cunningham et al. 2022; Botterill-James et al. 2023). Fallow deer currently occur at a mean density thought to be 2.7 deer per km2 within their core range in Tasmania (Lethbridge et al. 2020), compared with levels on one site on the Australian Mainland of 39.3 fallow and red deer per km2 (Bengsen et al. 2023). In Budj Bim National Park, Victoria, the density of fallow deer has been estimated as 17.6 deer/km2 (Woodford et al. 2023).
Conclusions
Our study confirms that fallow deer in Tasmania have a varied diet. Therefore, it is unlikely that the distribution of certain vegetation types, or availability of a limited range of plant species, will constrain the distribution of fallow deer in Tasmania. The capacity of fallow deer to browse as well as graze indicates a need for further research to identify potential impacts on woody vegetation. At high densities of deer, these impacts could include decline or disappearance of Tasmanian endemic plant taxa, further decline of grassy woodlands and significant changes to the structure of other vegetation communities.
Data availability
The data that support this study will be shared upon reasonable request to the corresponding author.
Declaration of funding
Funding provided through Australian Research Council (ARC) Linkage Grant and the Department of Natural Resources and Environment LP170100301.
Acknowledgements
We respectfully acknowledge the traditional custodians of the lands on which this research was conducted, the Tasmanian Aboriginal people. Authority to take plants for scientific purposes: Authority FL20193. Landowners: Julian and Annabel Von Bibra and the Tasmanian Land Conservancy. Bioinformatics was completed by eDNA Frontiers. Study area map created with assistance from Thomas Botterill–James. Field assistance: Kerry Bridle, Harrison Talricho, Dakota Murdoch, Jarryd Bailey. Logistical support: David Green, Vince Scoleri. Plant sample confirmation: Miguel de Salas and Matthew Baker at the Tasmanian Herbarium.
References
Bailey TG, Gauli A, Tilyard P, Davidson NJ, Potts BM (2015) Feral deer damage in Tasmanian restoration plantings. Australasian Plant Conservation: Journal of the Australian Network for Plant Conservation 23, 10-12.
| Crossref | Google Scholar |
Bengsen AJ, Forsyth DM, Pople A, et al. (2023) Effectiveness and costs of helicopter-based shooting of deer. Wildlife Research 50, 617-631.
| Crossref | Google Scholar |
Bentley A (1957) A brief account of the deer in Australia. The Journal of Wildlife Management 21, 221-225.
| Crossref | Google Scholar |
Botterill-James T, Cunningham CX, Johnson CN, et al. (2023) Projecting the dynamics of invading deer with pattern-oriented modelling to support management decision-making. Journal of Applied Ecology 61, 1-13.
| Crossref | Google Scholar |
Bruno E, Apollonio M (1991) Seasonal variations in the diet of adult male fallow deer in a submediterranean coastal area. Revue d’Écologie (Terre et Vie) 46, 349-362.
| Google Scholar |
Burkepile DE, Parker JD (2017) Recent advances in plant-herbivore interactions. F1000Research 6, 119.
| Crossref | Google Scholar | PubMed |
Calder JA, Kirkpatrick JB (2008) Climate change and other factors influencing the decline of the Tasmanian cider gum (Eucalyptus gunnii). Australian Journal of Botany 56, 684-692.
| Crossref | Google Scholar |
Chakanya C, Dokora A-E-M, Muchenje V, Hoffman LC (2016) The fallow deer (Dama spp.); endangered or not? Der Zoologische Garten 85, 160-172.
| Crossref | Google Scholar |
Chapman NG, Chapman DI (1980) The distribution of fallow deer: a worldwide review. Mammal Review 10, 61-138.
| Crossref | Google Scholar |
Claridge AW, Hunt R, Thrall PH, Mills DJ (2016) Germination of native and introduced plants from scats of Fallow Deer (Dama dama) and Eastern Grey Kangaroo (Macropus giganteus) in a south-eastern Australian woodland landscape. Ecological Management & Restoration 17, 56-62.
| Crossref | Google Scholar |
Clavel J, Julliard R, Devictor V (2011) Worldwide decline of specialist species: toward a global functional homogenization? Frontiers in Ecology and the Environment 9, 222-228.
| Crossref | Google Scholar |
Cowell S, Cameron A, Sprod D, Appleby M (2013) Midlandscapes: matching actions to opportunities in landscape conservation in the Tasmanian Midlands. In ‘Linking Australia’s landscapes: lessons and opportunities from large-scale conservation networks’. (Eds J Fitzsimons, I Pulsford, G Wescott) pp. 85–94. (CSIRO: Melbourne, Vic., Australia)
Cunningham CX, Perry GLW, Bowman DMJS, et al. (2022) Dynamics and predicted distribution of an irrupting ‘sleeper’ population: fallow deer in Tasmania. Biological Invasions 24, 1131-1147.
| Crossref | Google Scholar |
Czernik M, Taberlet P, Świslocka M, Czajkowska M, Duda N, Ratkiewicz M (2013) Fast and efficient DNA-based method for winter diet analysis from stools of three cervids: moose, red deer, and roe deer. Acta Theriologica 58, 379-386.
| Crossref | Google Scholar | PubMed |
Davidson NJ, Bailey TG, Burgess S (2021) Restoring the Midlands of Tasmania: an introduction. Ecological Management & Restoration 22, 3-10.
| Crossref | Google Scholar |
Davis NE, Forsyth DM, Bengsen AJ (2023) Diet and impacts of non-native fallow deer (Dama dama) on pastoral properties during severe drought. Wildlife Research 50, 701-715.
| Crossref | Google Scholar |
Duncan A (1992) The winter diets of fallow deer (Dama dama) and forester kangaroos (Macropus giganteus tasmaniensis) in the midlands of Tasmania. The Tasmanian Naturalist 110, 1-6.
| Google Scholar |
Erickson DL, Reed E, Ramachandran P, Bourg NA, McShea WJ, Ottesen A (2017) Reconstructing a herbivore’s diet using a novel rbcL DNA mini-barcode for plants. AoB PLANTS 9, plx015.
| Crossref | Google Scholar |
Esattore B, Saggiomo L, Sensi M, Francia V, Cherin M (2022) Tell me what you eat and I’ll tell you…where you live: an updated review of the worldwide distribution and foraging ecology of the fallow deer (Dama dama). Mammalian Biology 102, 321-338.
| Crossref | Google Scholar |
Genovesi P (2005) Eradications of invasive alien species in Europe: a review. Biological Invasions 7, 127-133.
| Crossref | Google Scholar |
Hall GP, Gill KP (2005) Management of wild deer in Australia. Journal of Wildlife Management 69, 837-844.
| Crossref | Google Scholar |
Hofmann RR (1989) Evolutionary steps of ecophysiological adaptation and diversification of ruminants: a comparative view of their digestive system. Oecologia 78, 443-457.
| Crossref | Google Scholar | PubMed |
Holechek JL, Vavra M, Pieper RD (1982) Botanical composition determination of range herbivore diets: a review. Journal of Range Management 35, 309-315.
| Crossref | Google Scholar |
Holz A, Wood SW, Veblen TT, Bowman DMJS (2015) Effects of high-severity fire drove the population collapse of the subalpine Tasmanian endemic conifer Athrotaxis cupressoides. Global Change Biology 21, 445-458.
| Crossref | Google Scholar | PubMed |
Jackson J (1977) The annual diet of the Fallow deer (Dama dama) in the New Forest, Hampshire, as determined by rumen content analysis. Journal of Zoology 181, 465-473.
| Crossref | Google Scholar |
Kamler J, Homolka M (2011) Needles in faeces: an index of quality of wild ungulate winter diet. Folia Zoologica 60, 63-69.
| Crossref | Google Scholar |
Kerridge FJ, Bullock DJ (1991) Diet and dietary quality of red deer and fallow deer in late summer. Journal of Zoology 224, 333-337.
| Crossref | Google Scholar |
Koziol A, Stat M, Simpson T, et al. (2019) Environmental DNA metabarcoding studies are critically affected by substrate selection. Molecular Ecology Resources 19, 366-376.
| Crossref | Google Scholar | PubMed |
Kress WJ, Erickson DL (2007) A two-locus global DNA barcode for land plants: the coding rbcL gene complements the non-coding trnH-psbA spacer region. PLOS ONE 2, e508.
| Crossref | Google Scholar |
Lurgi M, Galiana N, López BC, Joppa LN, Montoya JM (2014) Network complexity and species traits mediate the effects of biological invasions on dynamic food webs. Frontiers in Ecology and Evolution 2, 36.
| Crossref | Google Scholar |
Masseti M, Pecchioli E, Vernesi C (2008) Phylogeography of the last surviving populations of Rhodian and Anatolian fallow deer (Dama dama dama L., 1758). Biological Journal of the Linnean Society 93, 835-844.
| Crossref | Google Scholar |
McHugh ML (2013) The Chi-square test of independence. Biochemia Medica 23, 143-149.
| Crossref | Google Scholar | PubMed |
Mousavi-Derazmahalleh M, Stott A, Lines R, et al. (2021) eDNAFlow, an automated, reproducible and scalable workflow for analysis of environmental DNA sequences exploiting Nextflow and Singularity. Molecular Ecology Resources 21, 1697-1704.
| Google Scholar | PubMed |
Nakahara F, Ando H, Ito H, et al. (2015) The applicability of DNA barcoding for dietary analysis of sika deer. DNA Barcodes 3, 200-206.
| Crossref | Google Scholar |
Nugent G (1990) Forage availability and the diet of fallow deer (Dama dama) in the Blue Mountains, Otago. The New Zealand Journal of Ecology 13, 83-95.
| Google Scholar |
Pompanon F, Deagle BE, Symondson WOC, Brown DS, Jarman SN, Taberlet P (2012) Who is eating what: diet assessment using next generation sequencing. Molecular Ecology 21, 1931-1950.
| Crossref | Google Scholar | PubMed |
Potts JM, Beeton NJ, Bowman DMJS, Williamson GJ, Lefroy EC, Johnson CN (2015) Predicting the future range and abundance of fallow deer in Tasmania, Australia. Wildlife Research 41, 633-640.
| Crossref | Google Scholar |
Powell M (2023) Hundreds of deer carcasses left to rot in Tasmania’s World Heritage Wilderness Area after cull. ABC News. Available at https://www.abc.net.au/news/2023-05-26/711-deer-killed-in-tasmanias-first-aerial-shooting-cull/102391844 [Accessed 8 August 2023]
Prior LD, Sanders GJ, Bridle KL, Nichols SC, Harris R, Bowman DMJS (2013) Land clearance not dieback continues to drive tree loss in a Tasmanian rural landscape. Regional Environmental Change 13, 955-967.
| Crossref | Google Scholar |
Quarmby J, Kutt AS, Dickson CR, Hamer R (2023) Evaluating extinction risk in Tasmania’s vascular flora using rapid IUCN Red List assessments. Pacific Conservation Biology 30, 1-13.
| Crossref | Google Scholar |
Slatyer RA, Hirst M, Sexton JP (2013) Niche breadth predicts geographical range size: a general ecological pattern. Ecology Letters 16, 1104-1114.
| Crossref | Google Scholar |
Spitzer R, Felton A, Landman M, Singh NJ, Widemo F, Cromsigt JPGM (2020) Fifty years of European ungulate dietary studies: a synthesis. Oikos 129, 1668-1680.
| Crossref | Google Scholar |
Trabalza Marinucci M, Capecci A, Riganelli N, Acuti G, Antonini C, Olivieri O (2010) Dietary preferences and ruminal protozoal populations in roe deer (Capreolus capreolus), fallow deer (Dama dama) and mouflon (Ovis musimon). Italian Journal of Animal Science 4, 401-403.
| Google Scholar |
van der Heyde M, Bunce M, Wardell-Johnson G, Fernandes K, White NE, Nevill P (2020) Testing multiple substrates for terrestrial biodiversity monitoring using environmental DNA metabarcoding. Molecular Ecology Resources 20, 732-745.
| Crossref | Google Scholar |
Worth JRP, Jordan GJ, Marthick JR, et al. (2017) Fire is a major driver of patterns of genetic diversity in two co-occurring Tasmanian palaeoendemic conifers. Journal of Biogeography 44, 1254-1267.
| Crossref | Google Scholar |