Habitat loss and degradation reduce the abundance of the glossy grass skink, Pseudemoia rawlinsoni
Jules E. Farquhar

A
Handling Editor: Adam Stow
Abstract
Habitat loss and degradation are major drivers of biodiversity loss worldwide. In particular, wetland environments are being removed and degraded faster than any other terrestrial habitat on earth. The loss and degradation of wetlands has been particularly pronounced in south-eastern Australia.
Here we investigated the impact of habitat loss and degradation on the Data Deficient glossy grass skink (Pseudemoia rawlinsoni), a species that predominantly favours wetland vegetation in south-eastern Australia.
We established artificial cover-object (roofing tiles) survey grids in paired remnant and disturbed sites at six locations across Victoria, Australia, and surveyed for skinks between November 2021 and April 2022.
Sites at which glossy grass skinks occur are characterised by tall dense vegetation, with a high cover of matted biomass. Thermal profiles within these complex vegetation structures remain much cooler during hot days, and warmer during cold nights, than external temperatures. Nearby disturbed sites (i.e. grazed or mowed areas within dispersal distance of remnant sites) are generally devoid of skinks, have very low and structurally simple (open) vegetation, and have thermal regimes that offer lizards no respite from high summer temperatures. We found that roofing tiles are an effective way to survey for glossy grass skinks; even on cool cloudy days, the temperature of tiles, and the lizards sheltering beneath them, are often much higher than ambient temperatures.
These findings implicate habitat loss and degradation as having a substantial negative impact on glossy grass skink presence and abundance; skinks largely avoid disturbed areas, even at sites immediately adjacent to remnant habitat. This may be driven not simply by the removal of tall and dense vegetation structures, but the consequent loss of the optimal thermal buffer afforded by such structures.
Our study emphasises the threat that habitat loss and degradation pose to wetland species in Australia, and throughout the world.
Keywords: agriculture, artificial cover object, Data Deficient, habitat loss, lizard, reptile, thermal biology, threatened, vegetation structure, wetland.
Introduction
The global human population has rapidly increased over recent decades, and is predicted to reach 10 billion by the middle of the century (United Nations 2022). The impacts of this rapid human proliferation to biodiversity have been profound, with current species extinction rates estimated to be 100–1000 times greater than background rates (Pimm et al. 2014; Ceballos et al. 2015). Humans have been modifying landscapes with disturbances such as fire (Pyne 1997) and agriculture (Smith 1995) for thousands of years, but the impact of these activities has worsened following the industrial revolution (Steffen et al. 2007). As a consequence of ongoing and major changes in land-use/cover (Lambin and Geist 2006), many species have undergone significant population declines and extinctions (Dirzo et al. 2014; Ceballos et al. 2017, 2020). Anthropogenic habitat loss currently affects over 85% of species on the International Union for Conservation of Nature (IUCN) Red List of Threatened Species, and is the dominant threat for over 70% of those species (Hogue and Breon 2022).
In reptiles, habitat loss is a leading cause of population declines globally (Gibbons et al. 2000; Böhm et al. 2013; Doherty et al. 2020), and Australia has one of the worst track records for threatened species (Ritchie et al. 2013). Of Australia’s most threatened reptile taxa, invasive species (exotic weeds and predators) and agriculture are leading threats to the persistence of these taxa (Geyle et al. 2021). The Australian state of Victoria is particularly decimated by habitat loss; since European colonisation, extensive land conversion for agriculture and livestock has left the state with approximately only 30% of the original vegetation remaining (Bradshaw 2012). Consequently, many of Victoria’s reptiles are subject to ongoing population declines and local extinctions (Clemann 2015). To curtail this extinction crisis, conservation managers require a sufficient understanding of how populations will respond to anthropogenic habitat modification.
In addition to the outright loss of natural ecosystems, vegetative habitats are modified and degraded by humans, resulting in changes to the composition (e.g. native vs exotic spp.) and structure (e.g. simple vs complex) of plant communities. Invasive exotic plants can reduce reptile richness and abundance (Martin and Murray 2011; Abom et al. 2015; Lindenmayer et al. 2017); for example, some species of habitat-specialised alpine skinks avoid modified ski slopes dominated by exotic grasses (Sato et al. 2014a). But despite the importance of floral composition, much research has emphasised the role of vegetation structure on fauna (Garden et al. 2007; Mizsei et al. 2020; Cornelis et al. 2023). For example, the critically endangered striped legless lizard (Delma impar) appears somewhat tolerant of exotic vegetation, provided that its structural requirements are met (Dorrough and Ash 1999) and herbivore grazing pressure is not excessive (Howland et al. 2016). Vegetation-dependent fauna often have strong preferences for specific structural attributes of plant biomass, and are thus highly responsive to changes in vegetation structures (Howland et al. 2016; Nugent et al. 2022; Cornelis et al. 2023). With respect to grass-dwelling lizard ecology, complex vegetative structures are beneficial because they serve multiple important functions, such as providing structures to bask on (House and Spellerberg 1983), a higher abundance of invertebrate prey (Morton and James 1988), thermal buffering (Bell et al. 2021) and protection from predators such as birds (Sato et al. 2014b). Thus, the manipulation of vegetation structures via weed invasion, agriculture, livestock grazing, infrastructural works and recreational activities is expected to negatively affect vegetation-specialised fauna.
South-eastern Australia has seen a significant loss and degradation of wetland habitat from drainage works to convert the land for pasture or cropping, infilling for agriculture, or conversion to evaporative salt ponds for salt extraction (Sinclair and Boon 2012; Boon et al. 2015). Such alterations to wetlands also affect adjacent swamp scrub and grassland communities that receive periodic flooding from wetlands (Yugovic 2011), and hence affect fauna dependant on such vegetation and hydrology (Gorissen et al. 2017). Victoria’s freshwater lakes and swamps are largely found in fragmented landscapes, and more than two-thirds have been cleared or degraded (Victorian Environmental Assessment Council 2011). Fortunately, many Victorian wetlands have been retained as public land, most of which support remnant native vegetation on their margins (Victorian Environmental Assessment Council 2011). Nevertheless, reptiles that are dependent on wetland, or wetland-adjacent vegetation may be of particular conservation concern given that much of the landscape between wetlands has been cleared of connecting habitat. For example, the endangered swamp skink (Lissolepis coventryi) has suffered declines in population size and genetic diversity associated with fragmentation of its preferred dense wetland vegetation (Senior et al. 2022). Small ectotherms such as lizards generally have poor dispersal abilities, and thus make excellent models for evaluating the effects of habitat loss on populations at fine scales (Olsson and Shine 2003; Dubey and Shine 2010; Ackley et al. 2015; Doherty et al. 2020).
Understanding population fragmentation and dispersal characteristics can assist with predicting the impact of habitat loss on vegetation-specialised fauna (Sato et al. 2014a; Doherty et al. 2020). The glossy grass skink (Pseudemoia rawlinsoni) is a small (adult snout–vent length up to ~65 mm) lizard that appears to specialise on densely vegetated wetland habitats, with a disjunct distribution across south-eastern Australia, including Tasmania and alpine regions of New South Wales and the Australian Capital Territory (Hutchinson and Donnellan 1988; Wilson and Swan 2021; Farquhar et al. 2023). The glossy grass skink is currently listed as Data Deficient on the IUCN Red List because of a lack of information on its threats, ecology and populations status (Gillespie et al. 2018). Recent studies have demonstrated that Data Deficient reptiles are more likely to be threatened than are assessed species (Chapple et al. 2021; Caetano et al. 2022; Meiri et al. 2023). Thus, because habitat loss and degradation are a major threat to wetland habitats in south-eastern Australia (Boon et al. 2015), it is likely that the glossy grass skink has experienced range contractions and a reduction in connectivity since European colonisation (Gillespie et al. 2018). Indeed, the glossy grass skink is listed as Endangered in Victoria (which comprises the bulk of its geographic range) because of the loss, degradation and drainage of swamp habitats (Department of Environment, Land, Water and Planning 2022).
Here we conducted field surveys to determine the impact of habitat loss and degradation on the abundance and microhabitat of the glossy grass skink at six locations across Victoria. At each location, we examined paired remnant and disturbed habitats, and predicted that the glossy grass skink would be strongly affected by habitat loss and degradation, being absent or occurring in low abundances at disturbed sites. We expected that disturbed sites would have structurally simple vegetation, and that lower abundances of glossy grass skinks at such sites may reflect the species’ requirement for structurally complex habitats that provide suitable thermal regimes and protection from predators (e.g. Sato et al. 2014b).
Materials and methods
Study region
Although the glossy grass skink has a patchy distribution from the Australian Capital Territory, New South Wales, across Victoria, and into South Australia and Tasmania, travel restrictions owing to the COVID-19 pandemic limited our fieldwork to Victoria. We established 12 sites at six different locations; each location had paired sampling grids in remnant and disturbed habitats in wetland locations across Victoria, Australia, including Port Fairy, Queenscliff, Swan Bay, Yering, Tooradin and Warringine (Table 1, Fig. 1). Each location comprised one site in remnant native vegetation, which contained known populations of the glossy grass skink, and one paired nearby disturbed site (total of 12 tile-sampling grid sites; Table 1). The remnant sites had not experienced major anthropogenic disturbance for at least 15 years, and were managed by Parks Victoria (n = 2), Melbourne Water (n = 1), Council (n = 1), a rail trail committee (n = 1), and a private landholder (n = 1). The vegetation structure at remnant sites typically contained tall, dense vegetation. The paired disturbed sites were subject to frequent mowing by the land manager (n = 5) or grazing by livestock (n = 1). Consequently, vegetation at the disturbed sites was typically low and structurally simple (i.e. not dense).
Location | Treatment | Site name | Latitude | Longitude | |
---|---|---|---|---|---|
Port Fairy | Remnant | McColls Road | −38.346409 | 142.242085 | |
Disturbed | Chris’s front paddock | −38.346880 | 142.242171 | ||
Queenscliff | Remnant | Crownland | −38.268226 | 144.628523 | |
Disturbed | Marine Centre | −38.270315 | 144.633454 | ||
Swan Bay | Remnant | Sara’s swamp | −38.201634 | 144.676130 | |
Disturbed | Sara’s paddock | −38.201556 | 144.675679 | ||
Yering | Remnant | Spadonis Nature Reserve | −37.681576 | 145.349988 | |
Disturbed | Spadonis Aeroclub | −37.681850 | 145.348554 | ||
Tooradin | Remnant | Tooradin Inlet | −38.211158 | 145.427389 | |
Disturbed | Harewood | −38.212377 | 145.428118 | ||
Warringine | Remnant | Warringine Park | −38.325837 | 145.191561 | |
Disturbed | Mick’s paddock | −38.326758 | 145.186623 |
Occurrence records for Pseudemoia rawlinsoni in south-eastern Australia from Farquhar et al. (2023). Study locations are denoted by blue arrows: (a) Port Fairy, (b) Queenscliff, (c) Swan Bay, (d) Yering, (e) Tooradin, and (f) Warringine.
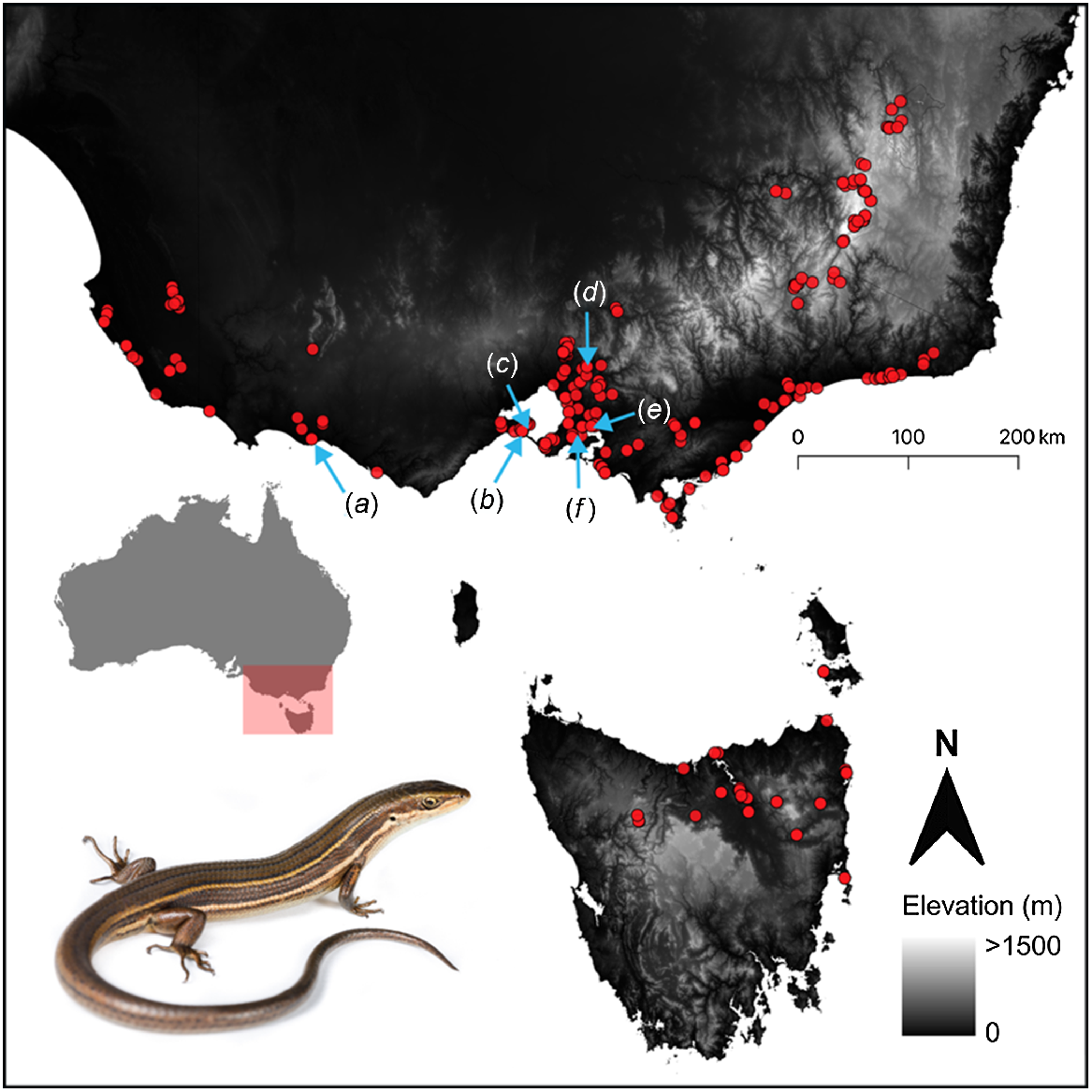
Quantifying habitat structure and microclimate
In October 2021, habitat-structure assessments were conducted at each site to quantify important aspects of the vegetation available to glossy grass skinks as habitat. At each site fifteen 1 m2 quadrats were randomly placed (by selecting random GPS coordinates within the site), and we visually estimated the percentage cover of rocks, logs, litter, bare ground and various plant life forms, including matts of vegetation, grass tussocks, sedges, rushes, reeds, shrubs, subshrubs (i.e. species with a woody base and soft, non-woody shoots), herbs, forbs and succulents. We also quantified structural aspects of the vegetation, including vegetation height and density, and shrub touches within three height classes (0–50 cm, 51–100 cm, 101–180 cm). Vegetation height was measured (in centimetres) at five standard positions within each quadrat by vertically inserting a 1.8 m measurement pole into the vegetation until it touched the soil surface, then recording the height of the tallest leaves in contact with the pole. At these same positions, density was measured as the percentage of the vegetation height that was visible to the observer (viewing the pole at a ~45° angle); hence, vegetation density was measured proportional to vegetation depth. The maximum litter depth found within the quadrat was recorded in centimetres. Canopy cover and canopy height were visually estimated as a percentage and in metres respectively.
To quantify the buffering capacity of vegetation, we attached two temperature data loggers (Thermochron iButton model DS1922L, Maxim Integrated Products, San Jose, CA, USA; ±0.5°C) to a wooden picket by using three layers of tan-coloured cloth tape. Pickets were inserted vertically into the soil, with one logger positioned in the air above the vegetation, and directly below this we placed the second logger within 0–1 cm of the soil surface. The difference in recorded temperature between these two logger positions is assumed to describe the thermal buffering capacity of vegetation biomass. Loggers were set to record hourly temperatures between 15 October 2021 and 2 March 2022 (138 days of data). The height of vegetation (in centimetres), and thus the distance separating the data loggers, was measured using our measurement-pole method described previously.
Lizard surveys
We deployed 600 terracotta roof tiles in mid-October 2021, then performed tile surveys at all sites once a month from November 2021 to April 2022 (excluding February, total of five survey sessions). Each site consisted of 50 tiles spaced 5 m apart, and in 10 rows of five tiles. The propensity for glossy grass skinks to use tiles as a shelter site, and therefore be detected, is likely to be weather dependent, as it is for other lizards (Hoare et al. 2009; Joppa et al. 2009; Andersson et al. 2010). Therefore, to mitigate against the potential for a single site visit to coincide with unfavourable weather, and to improve our capture rates during the survey session in each month, we checked the tiles three times within a 24 h period. Differences in lizard detection numbers within a 24 h period reflect the influence of weather and time of day, not seasonal shifts in activity and abundance (i.e. our focus). Thus, glossy grass skinks caught more than once within the 24 h session were noted, but not labelled as true recaptures, given the short period between tile checks; rather, we considered an individual a true recapture if it was captured again in subsequent survey sessions (i.e. months), given that this is the time scale at which we aimed to understand changes in abundance. To distinguish new individuals from specimens that we had previously detected, we maintained a record (a physical photo album) of the unique identity of each captured lizard based on a combination of features such as sex, size, location, scars, and missing limbs/digits. We avoided checking tiles during periods of heavy rain, and during summer we avoided checking tiles during the hottest part of the day when tiles would be too hot to be used by lizards. At the start of each survey, we recorded air temperature (°C), wind speed (km/h), relative humidity (%), cloud cover (0–8) and rain intensity (0–3).
Thermal ecology
Internal body temperature of each lizard was measured within 5–10 s of capture, by using a digital thermometer (Omega HH508 digital thermometer, Omega Engineering Inc.) with a K-type thermocouple probe inserted ~1 cm into the cloaca. The same digital thermometer was used to record the air temperature 5–10 cm above the surface where the lizard was caught. An infrared thermometer (°C, recorded with a Xueliee GM320) was used to measure the under-tile surface temperature, directly beneath the lizard. Wind speed (km/h) was then recorded using a hand-held anemometer (Kestrel 5000 weather meter, Nielsen–Kellerman) ~10 cm above the surface where the lizard was caught. Because most lizards were captured when inactive under roof tiles, these data were used to explore the thermal ecology of only inactive, sheltering lizards.
Statistical analysis
We conducted all statistical analyses in R (v4.1.2; R Core Team 2021). Analyses were not performed on abundance data. Given the clear contrast in abundance between disturbed and remnant sites (skinks are virtually absent from disturbed sites, see Results section), we produced bar plots of mean glossy grass skink abundance for each site to provide a visual comparison between treatments.
We performed a principal-component analysis (PCA; package FactoMineR; Lê et al. 2008) to examine whether vegetation structure and microclimate differ between remnant and disturbed sites. We first removed variables with few recorded values (e.g. canopy cover and canopy height were zero at all sites), then removed highly correlated variables by retaining the variable with a greater ecological relevance from each pairing of highly correlated variables (ρ ≥ 0.7). We then examined and retained all principal components (PCs) that explained more variation than that of their constituent variables (i.e. we retained eigenvalue of ≥1, as per the Kaiser–Guttman criterion; Jackson 1993). Student’s t-tests were then conducted on these PC scores to determine whether disturbed and remnant sites occupy significantly different positions along major axes (i.e. important PCs) of environmental variation.
We used linear regression to determine the effect that vegetation height and density have on microhabitat temperatures. For the first model, vegetation height was used as the independent variable and the difference between air and ground temperature in December (ΔTair-ground) as the dependent variable. For the second model, vegetation density was the independent variable, again with ΔTair-ground as the dependent variable. Finally, we used the December microclimate data to produce a line plot illustrating the median, minimum and maximum ΔTair-ground hourly differences between remnant and disturbed sites. Although we recorded iButton thermal data in all months, for analyses we subset the data to December because this is the hottest time of year, and, hence, a time when the thermal difference between inside and outside of vegetation should be most relevant to the persistence of cool-adapted lizards at these sites.
To explore the interaction between tiles and body temperature (Tb) of glossy grass skinks, we first tested the effect of under-tile temperature (Ttile) on Tb of sheltering lizards. Second, we tested whether the temperature differential (ΔT) between Ttile and air temperature (Tair) corresponds to an increased ΔT between Tb and Tair; our expectation with this second test is that tile-inactive lizards will be able to achieve a higher Tb than their surroundings by exploiting the ΔT afforded by tiles. Both tests were performed with linear mixed-effects models (function lmer) in the package lme4 (Bates et al. 2015), by using Tb as the dependent variable and Ttile as a fixed effect. Sex was also included as a fixed effect to account for possible differences in thermal physiology between sexes. Site was included as a random effect in both models. We did not use thermal data from the same individual twice.
Results
Lizard surveys
In total, 82 glossy grass skinks were captured during the study, 71 of which were unique individuals, and 11 were recaptures (November 2021: 19 individuals; December 2021: 22 individuals; January 2022: 16 individuals; March 2022: 13 individuals; and April 2022: 12 individuals). Of the 11 recaptures, seven were recaptured at the same tile as where they were previously captured at, and the remaining four individuals were all recaptured at short distances from their initial capture point (5, 6, 6.5 and 9 m). Whereas the species was detected at seven sites (six remnant sites, and one disturbed site), the total number of individuals captured varied considerably among sites (Fig. 2). Two sites located in high-quality coastal saltmarsh habitat on the Bellarine Peninsula provided the majority (62%) of the individuals in this study, with 28 lizards at the Queenscliff remnant site and 16 at the Swan Bay remnant site. Our key finding was the stark differences in abundance of glossy grass skinks at remnant sites from those of nearby disturbed sites (Fig. 2); the species was not caught at any of the disturbed sites, except at one, the disturbed Swan Bay site (Fig. 2; see Discussion). The other reptiles and amphibians observed under tiles during our surveys are shown in Table S1.
Factors associated with the presence of glossy grass skinks
PC1 alone explained a considerable amount (52.1%) of the variation in the habitat data (Fig. 3a, Table 2) and therefore describes an important axis of environmental variation at our study sites. PC2 (21.8% explained variation) and PC3 (15% explained variation) were retained because they each had eigenvalues of >1; however, neither PC2 (t = −0.06, d.f. = 9.99, P = 0.951) nor PC3 (t = 0.11, d.f. = 9.62, P = 0.916) differed significantly between site types. However, along the PC1 axis there is clear separation of disturbed versus remnant sites: remnant sites are characterised by increasing values (positive PC1 scores) of vegetation height, vegetation density, matted vegetation cover, and ΔTair-ground, whereas disturbed sites are characterised by increasing values (negative PC1 scores) of bare ground, herbs, and non-matted vegetation. The PC1 scores of remnant sites, where glossy grass skinks are abundant, are significantly different from those of disturbed sites, where glossy grass skinks are absent or very low in abundance (t = −12.50, d.f. = 8.70, P < 0.001). Thus, glossy grass skinks favour structurally complex habitat (tall, dense and matted vegetation) that provides high values of ΔTair-ground, and avoid structurally simple habitat (short, open vegetation with high bare-ground cover) that provides low values of ΔTair-ground (Fig. 3a).
(a) Principal-component analysis of vegetation-structure data obtained from remnant (blue) sites (favoured by Pseudemoia rawlinsoni) and disturbed (red) sites. Colour-shaded ellipses represent 90% confidence intervals. (b) Correlation circle of the seven habitat variables. The length and directionality of arrows (vectors) illustrate respectively, the strength of contribution (loading) and sign of correlation (positive, negative) of each variable on the two components (PC1: x-axis; PC2: y-axis). Variables closer to each other are more correlated on a given component.
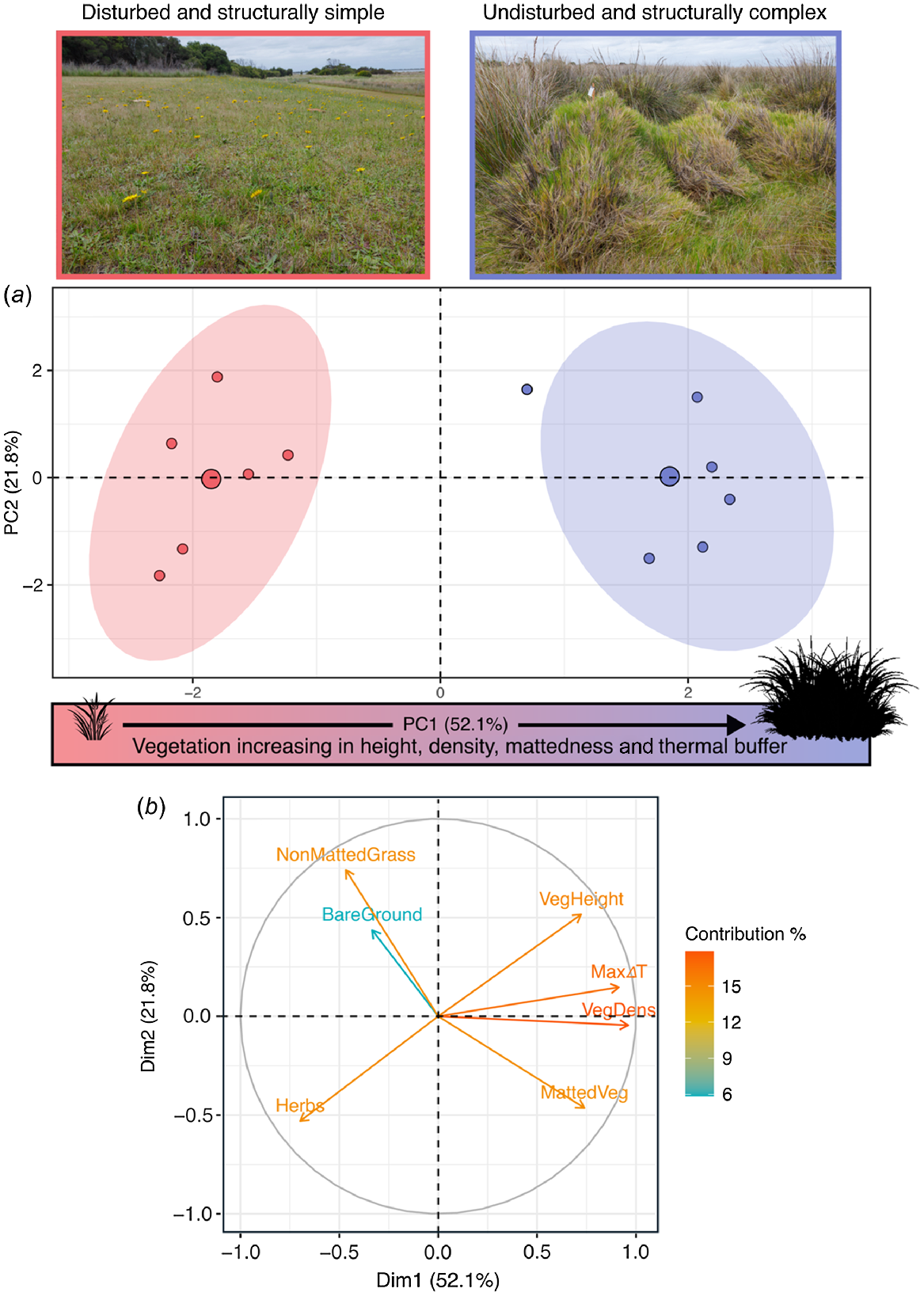
Item | PC1 | PC2 | PC3 | |
---|---|---|---|---|
Proportion of variance | 0.52 | 0.21 | 0.15 | |
Cumulative proportion | 0.52 | 0.73 | 0.89 | |
Eigenvalue | 3.64 | 1.52 | 1.06 | |
Loadings | ||||
Bare ground | −0.33 | 0.43 | 0.76 | |
Matted vegetation | 0.73 | −0.46 | 0.40 | |
Non-matted grass | −0.46 | 0.74 | −0.20 | |
Herbs | −0.69 | −0.53 | −0.37 | |
Vegetation height | 0.72 | 0.51 | −0.32 | |
Vegetation density | 0.96 | −0.04 | −0.02 | |
Maximum ΔT | 0.91 | 0.14 | −0.16 |
The first three PCs are shown because these each had an eigenvalue of >1.
The ΔTair-ground (°C) values at remnant sites in December were positive during daylight hours (i.e. the ground beneath vegetation was cooler than the external ambient air; Fig. 4a). The opposite trend was observed for the disturbed sites; at disturbed sites, low and open vegetation affords minimal thermal buffer, with daytime ground temperatures becoming much hotter than air temperatures (hence, a negative x-axis value for disturbed sites in Fig. 4a). Both remnant and disturbed sites had similarly low ΔTair-ground (°C) values at night, but were slightly negative at remnant sites and zero at disturbed sites. In other words, the night time ground temperatures beneath vegetation were slightly warmer than the external air at remnant (but not at disturbed) sites.
(a) iButton microhabitat temperature data, showing the median ΔTair-ground (i.e. degrees of difference between air and ground temperature under vegetation) at all remnant (blue) and disturbed (red) sites in December (the hottest time of year). The broad shaded zones indicate the maximum and minimum ΔTair-ground. Values of zero on the Y-axis indicate no temperature difference between air and under vegetation. Negative values indicate that that the temperature under vegetation is warmer than the air; positive values indicate the opposite. These thermal regimes are explained by vegetation structure: (b) linear regression of vegetation height and (c) density against the ΔTair-ground in December, with a shaded 95% confidence interval (grey), the line of best fit (blue), and R2 correlation coefficient. **Statistically significant correlation, P < 0.01; ***statistically significant correlation, P < 0.001.
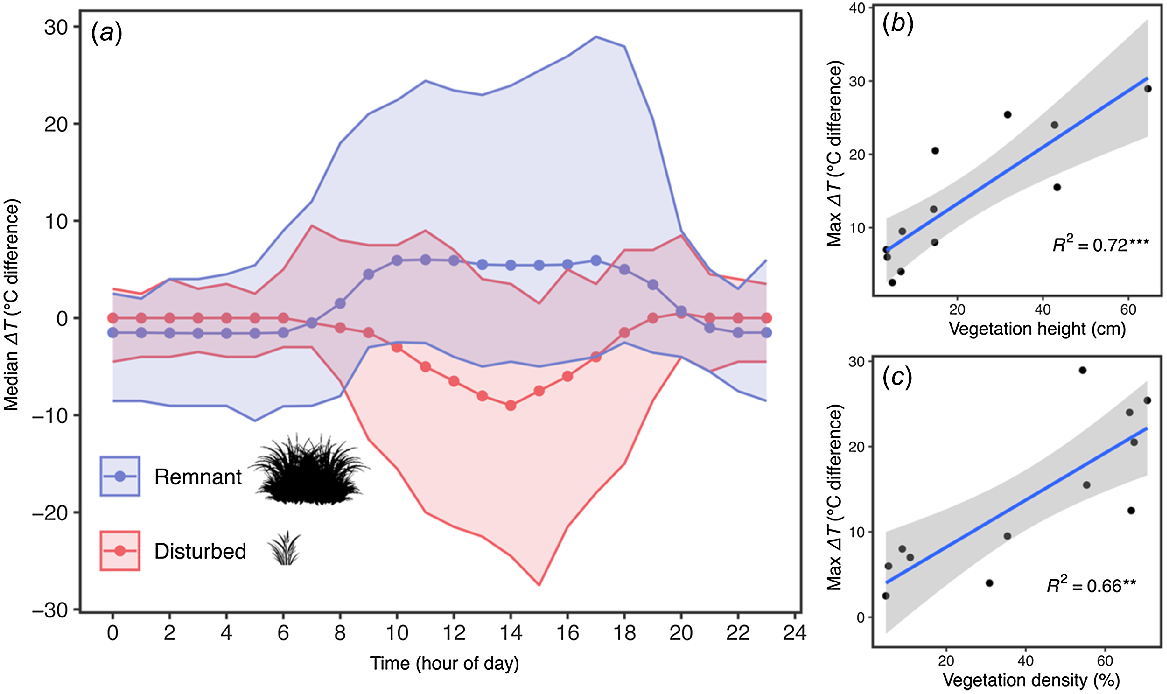
Linear regression showed that December ΔTair-ground (°C) was positively associated with vegetation height (R2 = 0.72, F (1,10) = 25.88, P < 0.001; Fig. 4b) and vegetation density (R2 = 0.66, F (1,10) = 19.79, P = 0.001; Fig. 4c), suggesting a causal relationship between complex vegetation and thermally buffered microclimates at wetland margins.
Thermal ecology
The body temperature of glossy grass skinks sheltering beneath tiles had a positive association with the under-tile surface temperature (d.f. = 43.78, t = 12.50, P < 0.001; Fig. 5a, Table S2). There was also a positive association between ΔTtile-air and ΔTlizard-air (d.f. = 38.20, t = 7.87, P < 0.001; Fig. 5b, Table S3), indicating that the degree of difference between tile-sheltering lizard Tbs and the external air temperature is dependent on the degree to which under-tile surface temperature differs from that of the external air. The median and range values for weather conditions under which glossy grass skink was detected are presented in Table S4.
(a) Linear regression of tile temperature and body temperature of Pseudemoia rawlinsoni, showing a strong positive correlation. (b) Linear regression of ΔTtile-air temperature and ΔTlizard-air temperature, showing a moderate positive correlation. The line of best fit and 95% confidence interval are denoted by the blue line and grey shaded zone respectively. The R2 correlation coefficient is provided. ***Statistically significant correlation, P < 0.001.
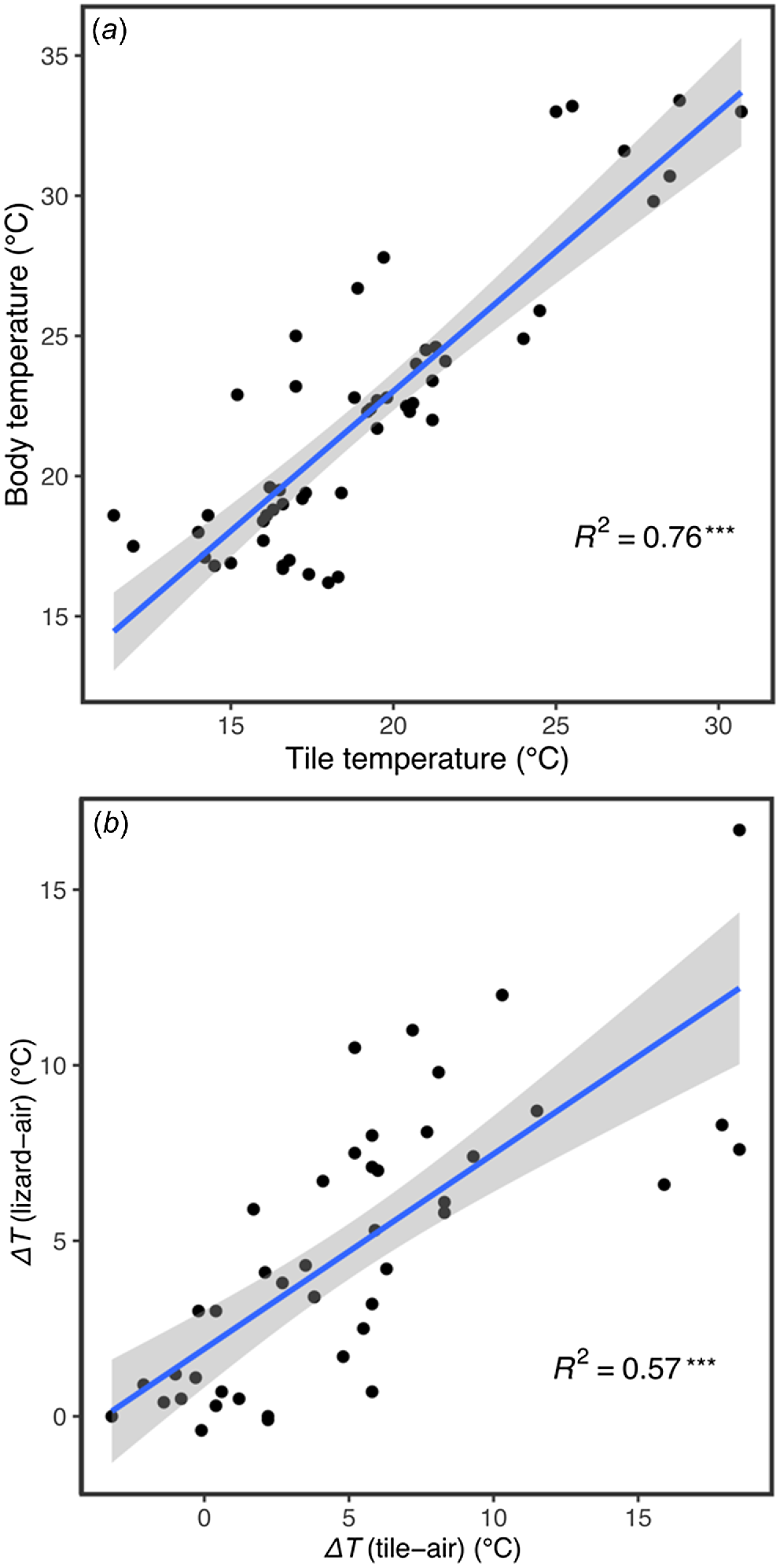
Discussion
Our results showed that habitat loss and degradation have an impact on the distribution and abundance of the glossy grass skink. The presence of glossy grass skinks is associated with vegetation complexity, described in this study by the presence of matted, tall and dense vegetation, which in turn creates a higher thermal-buffering effect than at structurally simple sites. At disturbed sites, the simple vegetation structure (short, open grass) affords no such thermal buffer, with daytime surface temperatures exceeding air temperatures, owing to the negligible cover of vegetation. Our study also demonstrated that roofing tiles are an effective survey and monitoring method for glossy grass skinks, because the surfaces beneath tiles offer temperatures that are often much warmer than external conditions. Below we discuss the implications of our results for the conservation of the glossy grass skink and for future survey and management efforts.
Impact of habitat loss and degradation on glossy grass skink
Farquhar et al. (2023) found that 38% of the glossy grass skink’s predicted distribution has been converted to agricultural land, with apparent population extirpations from many sites in agricultural areas (inferred from field surveys at known record sites). As a further testament to the impact of agricultural development on the species, the present study showed that glossy grass skinks were virtually absent from the disturbed, structurally simple vegetation that typifies farm paddocks, which are usually mowed with machinery and/or grazed by livestock. Thus, the removal of tall and dense native vegetation, and its replacement with frequently disturbed low pasture grasses, is likely a contributing driver of ongoing declines in glossy grass skink populations.
Our finding that glossy grass skinks are largely dependent on complex wetland vegetation emphasises the ecological value of these habitats for the conservation of the species, particularly for their thermal-buffering capacity. To avoid thermal extremes and optimise performance, reptiles seek thermally buffered retreat sites (Huey et al. 1989; Sato et al. 2014b; Moore et al. 2018). Species depending on vegetation for thermoregulation may be disadvantaged when biomass is removed or altered through mowing or grazing, which effectively eliminates the thermal buffer available for lizards (Sato et al. 2014b). Being exposed to higher ground surface temperatures at sites lacking vegetation cover, the efficiency of behavioural thermoregulation may be reduced (Kearney et al. 2009), because lizards contend with shorter thermally optimum activity-time windows (Huey et al. 2010). We suspect that the reduced thermal quality of matrix habitat is a leading ecological factor that limits glossy grass skinks predominantly to dense remnant vegetation. Our temperature-data loggers demonstrated a strong relationship between vegetation complexity and summertime thermal buffering; in structurally simple disturbed habitat, the lack of vegetative cover makes ground surface temperatures often hotter than the ambient temperature during thermally challenging periods (midday–mid-afternoon). Conversely, undisturbed sites with glossy grass skinks typically feature dense vegetation, beneath which lizards can access temperatures many degrees cooler than the ambient temperature. Additional research into the thermal adaptation of the glossy grass skink is required to put our findings into a robust ecophysiological context. Once the thermal-tolerance limits and thermal preference of the species are known, we can then model the sensitivity of the species to variation in the thermal landscape (Moore et al. 2018; Garcia and Clusella-Trullas 2019).
Dispersal capacity of glossy grass skink
If the glossy grass skink were a habitat generalist, we perhaps would have detected individuals at disturbed sites with some regularity. Indeed, other generalist lizard species were often detected at most disturbed sites (e.g. grass skink (Lampropholis guichenoti), southern grass skink (Pseudemoia entrecasteauxii); Table S1), indicating that such sites are not entirely unsuitable for lizards. Instead, the glossy grass skink exhibits a near complete avoidance of disturbed sites; by positioning our paired disturbed and remnant sites in close proximity to each other (i.e. within dispersal distance of small lizards), we could determine the degree to which they are confined to remnant swamp vegetation. The avoidance of disturbed habitat was also found in habitat-specialist skinks such as alpine she-oak skink (Cyclodomorphus praealtus) and the tussock skink (Pseudemoia pagenstecheri) that are rarely found in mowed, structurally open ski fields (Sato et al. 2014a). Similarly, Gorissen et al. (2017) showed that the Blue Mountains water skink (Eulamprus leuraensis) is restricted to densely vegetated and wet swamp habitat, and was not detected in any disturbed site. We recaptured glossy grass skinks either in the same location or at short distances (<10 m) from their initial capture points, suggesting that the species may have small home ranges with limited capacity for dispersal. However, because our recaptures were few, a more focused study on home-range size is needed to understand this species’ spatial ecology in greater detail (e.g. Olsson and Shine 2003).
The glossy grass skink was detected at only one disturbed site (Sara’s paddock, Swan Bay); however, it is noteworthy that this disturbed (regularly mowed) site was intentionally situated in very close proximity (~10 m) to undisturbed vegetation occupied by the species. This suggests that the species has some capacity for short-distance dispersal into the disturbed habitat matrix adjacent to high-quality remnant habitat. Given this, activities such as mowing and grazing can cause direct mortality of individuals and hinder dispersal outside of habitat patches (Sato et al. 2014b). Indeed, on vehicle tracks through swampy habitat, dead glossy grass skinks (with photographic evidence) have been found at Lake Connewarre (immediately following track resurfacing works; B. Lingham, pers. comm. 2021) and at a desalination plant near Wonthaggi (C. Boase, pers. comm. 2021).
Together these findings implicate glossy grass skink as a habitat specialist, largely confined to complex and mostly undisturbed swampy vegetation. However, it also has the capacity to disperse into adjacent disturbed and structurally simple vegetation communities, which, if left to regenerate, may become sufficiently complex and more habitable for the species. We caution that biomass disturbance within and adjacent to glossy grass skink habitat has the potential to adversely affect the species, via the loss of potential dispersal habitat and direct mortality of individuals. If mowing of vegetation in or near glossy grass skink habitat is unavoidable, such activities should be restricted to winter months (June–August), when lizard activity is lowest.
Managing vegetation for glossy grass skink conservation
Although this study emphasises the importance of intact native vegetation, we are careful not to discount the potential value of disturbed and exotic habitats for the glossy grass skink. There is a pervasive notion that disturbed and exotic vegetation communities are synonymous with ‘unsuitable’ habitat for threatened species (Davis et al. 2011). Whereas this is partially true for some disturbance-intolerant species (e.g. Sato et al. 2014a; Wong et al. 2021), the reality is that disturbed sites with exotic vegetation can fulfil the ecological needs of threatened species (Lampert et al. 2014; Howland et al. 2016), even if suboptimally and in the short term. For example, there are numerous observations of glossy grass skinks occupying sites containing, or dominated by, Kikuyu grass (Cenchrus clandestinus), a globally significant invasive weed (Herrero-Borgoñón et al. 1995). Kikuyu grass grows in dense and connected clump formations, and, in doing so, we suggest that its structure may be analogous to that of some native grasses, such as Australian salt-grass (Distichlis distichophylla), a plant commonly used by glossy grass skink in high-quality remnant habitat in coastal areas. Glossy grass skink has been observed at a site on the outskirts of Korumburra (South Gippsland) confined to a small patch of dense Kikuyu grass, surrounded by buildings and mowed lawn-grass on one side, and a grazed cattle paddock on the other, both with very low and open exotic biomass (R. Kidd, pers. comm. 2021). We highlight this example to demonstrate the difficulties that may emerge in the conservation management of glossy grass skink, particularly in urban areas were populations still persist in degraded and isolated habitat patches that are subject to mowing, slashing, invasive plants and infrastructural developments.
It is typically desirable to control weedy vegetation, owing to its impacts on native fauna persistence and ecosystem function (Valentine et al. 2007; Martin and Murray 2011; Sato et al. 2014a; Abom et al. 2015; Lindenmayer et al. 2017). But in highly modified landscapes lacking native vegetation, the structural complexity afforded by some exotic grasses is perhaps better (for the species’ persistence) than is a complete lack of vegetation (Garden et al. 2007; Howland et al. 2016; Cornelis et al. 2023). For example, Kikuyu-dominated wetlands in Perth were found to offer tiger snakes similar habitat quality (thermally and structurally) to that of native vegetation (Cornelis et al. 2023). This is of course no advocacy of exotic grass proliferation, but conservation practitioners responsible for biomass management must recognise the potential conservation value of these disturbed and exotic, but nonetheless complex and habitable, plant communities (Davis et al. 2011; Lampert et al. 2014).
Potential impact of tree and shrub planting on glossy grass skink
The present study focused on exploring structurally simple versus complex strata (grasses, herbs, low shrubs, etc.) at the ground level, rather than exploring differences between open versus closed canopy. This was by necessity, given that most known glossy grass skink occurrence localities tend to lack canopy cover such as tall trees; most record sites are situated in open swampy areas that naturally have little or no canopy (dataset in Farquhar et al. 2023). It is possible that high canopy cover, and hence reduced incident solar radiation, is detrimental to the species’ persistence, at least at some sites. There is some preliminary support for this; for instance, the species was routinely detected at an ecological monitoring site in seasonally swampy habitat in Bayswater North (in Melbourne’s east), but over the course of a decade, its apparent disappearance coincided with the overgrowth of densely planted trees, tall shrubs and tussocks (D. De Angelis, pers. comm. 2021). It is likely that most current revegetation projects are not sympathetic to the habitat structural requirements of glossy grass skink. Dense plantings or natural encroachments of trees and tall shrubs can increase shading of the ground strata, thereby reducing basking- and shelter-site suitability for heliothermic reptiles adapted to more open canopy habitats (House and Spellerberg 1983; Todd and Andrews 2008; Michael et al. 2011). Revegetation initiatives should aim to restore historic vegetation composition and structure for glossy grass skink, rather than aiming to meet arbitrary ‘number-of-trees-planted’ quotas that may result in overly shaded biomass.
Further research is needed to understand the glossy grass skink’s sensitivity to different revegetation schemes; only then can we confidently develop a well-informed framework for reinstating its habitat at degraded sites. In situations where habitat is degraded and significantly altered from its original state, the right answer to the question ‘How best to manage biomass for the glossy grass skink?’ is likely to be elusive and context dependent. This is not least because the species has a wide distribution, over which it occupies many different habitats, from coastal swamps to alpine bogs (Farquhar et al. 2023). Thus, conservation and land management efforts will need to consider the historical habitat state, its legacy of disturbance, the extent of invasive plant encroachment, fire and hydrological regimes, and the (sometimes conflicting) ecological needs of co-occurring priority species (Michael et al. 2011). Nonetheless, for existing habitat, the preservation of structurally complex and undisturbed native vegetation should be a general conservation priority for the glossy grass skink (Sato et al. 2014a).
Roofing tiles are an effective survey method for glossy grass skink
As a diurnal heliothermic ectotherm, the glossy grass skink controls its body temperature via behavioural thermoregulation, and will bask on grass, rocks and logs, as well as artificial cover objects (ACOs; e.g. roofing tiles), so as to achieve ideal body temperatures (Hoare et al. 2009; Andersson et al. 2010; Saleeba et al. 2020). The species makes frequent use of roofing tiles (when available) as a thermally favourable shelter site. Indeed, we found that glossy grass skink body temperature was strongly correlated with under-tile surface temperature, including on cool, cloudy days during which lizards sheltered beneath tiles to achieve Tbs much warmer than external air temperatures (Hoare et al. 2009; Joppa et al. 2009). Thus, roofing tiles have proved a useful survey tool for glossy grass skinks, and other reptile and frog species (see Table S1), because of their thermal properties (Saleeba et al. 2020).
Wetlands are fragile ecosystems (Tooth and McCarthy 2007), and digging into soil to install pitfall traps, and rolling logs and rocks can be damaging to the habitat. Although visual-encounter surveys are a low-impact survey method, this method is practical only for detecting active lizards, which may be difficult to observe and capture when habitat is complex (Hoare et al. 2009). Additionally, live-trapping, such as pitfall trapping and funnel trapping, increases the risk of predation, drowning and heat stress for the target species (Enge 2001). Artificial cover objects are a low-cost survey method that has very little negative environmental impact (Hoare et al. 2009). This method has also been shown to be successful at capturing other lizard species that co-occur with glossy grass skink in wetland habitats, such as the swamp skink (Humphrey et al. 2017; present study). The use of ACOs also increases the accuracy of surveys because it reduces the chance of misidentification of a species, because tile-sheltering lizards are easily hand-captured (Sewell et al. 2012). Weather conditions for detecting lizards beneath ACOs should be considered when using this sampling method, although their direct impacts are not clearly understood and may be specific to taxa (Hoare et al. 2009; Joppa et al. 2009). For glossy grass skinks, we found that the ideal weather conditions for capture beneath tiles were moderate temperatures, relative humidity and cloud cover, and low wind speeds (see Table S4).
Conclusions
We have demonstrated that habitat loss and degradation are a clear threat to the persistence of wetland reptiles, and may limit the dispersal and connectivity among isolated subpopulations. The glossy grass skink is more abundant in (and may require) sites with tall, dense, and matted vegetation. In general, grazed or mowed sites with very short and structurally simple vegetation appear to be avoided, and, as we have shown, this may be explained by the lack of thermally buffered microclimates in such sites. However, we emphasise that disturbed sites can still be valuable conservation assets that are used by dispersing individuals, and may become more habitable if left to regenerate. In general, conservation management actions should ensure that complex, native vegetation structure is preserved and incorporated in revegetation planning for this species. However, we suspect that the relationship among glossy grass skink abundance, site occupancy and habitat structure is likely to be more complex and context-dependent than that identified in the present study. Nonetheless, our study offers the first insight into the general habitat requirements of the glossy grass skink, and highlights its vulnerability to habitat loss and degradation.
Data availability
The data and code associated with this study is provided in the supplementary material.
Declaration of funding
This work was supported by a grant from the Australian Research Council (FT200100108) to D. G. Chapple.
Acknowledgements
We thank Amelia Carlesso, Bethany McLeod and Margaret Haines for assistance with fieldwork, and Nick Schultz and Wyn Russell for useful discussions regarding our habitat-structure assessment methods. We also thank the following institutions for providing access to their land: Parks Victoria, Melbourne Water, Mornington Peninsula Shire Council, Yarra Ranges Shire Council, Queenscliff Marine and Freshwater Discovery Centre, and Port Fairy to Warrnambool Rail Trail Committee of Management. Several landowners/managers generously accommodated our tile surveys on their property, including Chris Ward, Philip Du Guesclin, Sara Randell, Paul Slinger, Gary Leckie, Dan Reed, Pat Macwhirter, Michael Peck, Bill Koolstra, David Simms and Guy Mayer.
References
Abom R, Vogler W, Schwarzkopf L (2015) Mechanisms of the impact of a weed (grader grass, Themeda quadrivalvis) on reptile assemblage structure in a tropical savannah. Biological Conservation 191, 75-82.
| Crossref | Google Scholar |
Ackley JW, Wu J, Angilletta MJ, Jr., Myint SW, Sullivan B (2015) Rich lizards: how affluence and land cover influence the diversity and abundance of desert reptiles persisting in an urban landscape. Biological Conservation 182, 87-92.
| Crossref | Google Scholar |
Andersson M, Krockenberger A, Schwarzkopf L (2010) Experimental manipulation reveals the importance of refuge habitat temperature selected by lizards. Austral Ecology 35, 294-299.
| Crossref | Google Scholar |
Bates D, Mächler M, Bolker B, Walker S (2015) Fitting linear mixed-effects models using lme4. Journal of Statistical Software 67, 1-48.
| Crossref | Google Scholar |
Bell KJ, Doherty TS, Driscoll DA (2021) Predators, prey or temperature? Mechanisms driving niche use of a foundation plant species by specialist lizards. Proceedings of the Royal Society B: Biological Sciences 288, 20202633.
| Crossref | Google Scholar |
Boon PI, Allen T, Carr G, Frood D, Harty C, McMahon A, Mathews S, Rosengren N, Sinclair S, White M, Yugovic J (2015) Coastal wetlands of Victoria, south-eastern Australia: providing the inventory and condition information needed for their effective management and conservation. Aquatic Conservation: Marine and Freshwater Ecosystems 25, 454-479.
| Crossref | Google Scholar |
Bradshaw CJA (2012) Little left to lose: deforestation and forest degradation in Australia since European colonization. Journal of Plant Ecology 5, 109-120.
| Crossref | Google Scholar |
Böhm M, Collen B, Baillie JEM, et al. (2013) The conservation status of the world’s reptiles. Biological Conservation 157, 372-385.
| Crossref | Google Scholar |
Caetano GHdO, Chapple DG, Grenyer R, Raz T, Rosenblatt J, Tingley R, Böhm M, Meiri S, Roll U (2022) Automated assessment reveals that the extinction risk of reptiles is widely underestimated across space and phylogeny. PLoS Biology 20, e3001544.
| Crossref | Google Scholar |
Ceballos G, Ehrlich PR, Barnosky AD, García A, Pringle RM, Palmer TM (2015) Accelerated modern human–induced species losses: entering the sixth mass extinction. Science Advances 1, e1400253.
| Crossref | Google Scholar | PubMed |
Ceballos G, Ehrlich PR, Dirzo R (2017) Biological annihilation via the ongoing sixth mass extinction signaled by vertebrate population losses and declines. Proceedings of the National Academy of Sciences of the United States of America 114, E6089-E6096.
| Crossref | Google Scholar | PubMed |
Ceballos G, Ehrlich PR, Raven PH (2020) Vertebrates on the brink as indicators of biological annihilation and the sixth mass extinction. Proceedings of the National Academy of Sciences of the United States of America 117, 13596-13602.
| Crossref | Google Scholar | PubMed |
Chapple DG, Roll U, Böhm M, Aguilar R, Amey AP, Austin CC, Baling M, Barley AJ, Bates MF, Bauer AM, Blackburn DG, Bowles P, Brown RM, Chandramouli SR, Chirio L, Cogger H, Colli GR, Conradie W, Couper PJ, Cowan MA, Craig MD, Das I, Datta-Roy A, Dickman CR, Ellis RJ, Fenner AL, Ford S, Ganesh SR, Gardner MG, Geissler P, Gillespie GR, Glaw F, Greenlees MJ, Griffith OW, Grismer LL, Haines ML, Harris DJ, Hedges SB, Hitchmough RA, Hoskin CJ, Hutchinson MN, Ineich I, Janssen J, Johnston GR, Karin BR, Keogh JS, Kraus F, LeBreton M, Lymberakis P, Masroor R, McDonald PJ, Mecke S, Melville J, Melzer S, Michael DR, Miralles A, Mitchell NJ, Nelson NJ, Nguyen TQ, de Campos Nogueira C, Ota H, Pafilis P, Pauwels OSG, Perera A, Pincheira-Donoso D, Reed RN, Ribeiro-Júnior MA, Riley JL, Rocha S, Rutherford PL, Sadlier RA, Shacham B, Shea GM, Shine R, Slavenko A, Stow A, Sumner J, Tallowin OJS, Teale R, Torres-Carvajal O, Trape J-F, Uetz P, Ukuwela KDB, Valentine L, Van Dyke JU, van Winkel D, Vasconcelos R, Vences M, Wagner P, Wapstra E, While GM, Whiting MJ, Whittington CM, Wilson S, Ziegler T, Tingley R, Meiri S (2021) Conservation status of the world’s skinks (Scincidae): taxonomic and geographic patterns in extinction risk. Biological Conservation 257, 109101.
| Crossref | Google Scholar |
Clemann N (2015) Cold-blooded indifference: a case study of the worsening status of threatened reptiles from Victoria, Australia. Pacific Conservation Biology 21, 15-26.
| Crossref | Google Scholar |
Cornelis J, von Takach B, Cooper CE, Vos J, Bateman PW, Lettoof DC (2023) Quantifying the impacts of an invasive weed on habitat quality and prey availability for tiger snakes (Notechis scutatus) in urban wetlands. Wetlands Ecology and Management 31, 715-732.
| Crossref | Google Scholar |
Davis MA, Chew MK, Hobbs RJ, Lugo AE, Ewel JJ, Vermeij GJ, Brown JH, Rosenzweig ML, Gardener MR, Carroll SP, Thompson K, Pickett STA, Stromberg JC, Tredici PD, Suding KN, Ehrenfeld JG, Philip Grime J, Mascaro J, Briggs JC (2011) Don’t judge species on their origins. Nature 474, 153-154.
| Crossref | Google Scholar | PubMed |
Department of Environment, Land, Water and Planning (2022) Flora and Fauna Quarantee Act 1988 – threatened list. September 2022. The State of Victoria Department of Environment, Land, Water and Planning. Available at https://www.environment.vic.gov.au/__data/assets/pdf_file/0031/536089/FFG-Threatened-List-September-2022.pdf [accessed 13 March 2023]
Dirzo R, Young HS, Galetti M, Ceballos G, Isaac NJB, Collen B (2014) Defaunation in the Anthropocene. Science 345, 401-406.
| Crossref | Google Scholar | PubMed |
Doherty TS, Balouch S, Bell K, Burns TJ, Feldman A, Fist C, Garvey TF, Jessop TS, Meiri S, Driscoll DA (2020) Reptile responses to anthropogenic habitat modification: a global meta-analysis. Global Ecology and Biogeography 29, 1265-1279.
| Crossref | Google Scholar |
Dorrough J, Ash JE (1999) Using past and present habitat to predict the current distribution and abundance of a rare cryptic lizard, Delma impar (Pygopodidae). Australian Journal of Ecology 24, 614-624.
| Crossref | Google Scholar |
Dubey S, Shine R (2010) Restricted dispersal and genetic diversity in populations of an endangered montane lizard (Eulamprus leuraensis, Scincidae). Molecular Ecology 19, 886-897.
| Crossref | Google Scholar | PubMed |
Enge KM (2001) The pitfalls of pitfall traps. Journal of Herpetology 35, 467-478.
| Crossref | Google Scholar |
Farquhar JE, Carlesso A, Pili A, Gale N, Chapple DG (2023) Capturing uncatalogued distribution records to improve conservation assessments of data-deficient species: a case study using the glossy grass skink. Animal Conservation 27, 124-137.
| Crossref | Google Scholar |
Garcia RA, Clusella-Trullas S (2019) Thermal landscape change as a driver of ectotherm responses to plant invasions. Proceedings of the Royal Society B: Biological Sciences 286, 20191020.
| Crossref | Google Scholar |
Garden JG, Mcalpine CA, Possingham HP, Jones DN (2007) Habitat structure is more important than vegetation composition for local-level management of native terrestrial reptile and small mammal species living in urban remnants: a case study from Brisbane, Australia. Austral Ecology 32, 669-685.
| Crossref | Google Scholar | PubMed |
Geyle HM, Tingley R, Amey AP, Cogger H, Couper PJ, Cowan M, Craig MD, Doughty P, Driscoll DA, Ellis RJ, Emery J-P, Fenner A, Gardner MG, Garnett ST, Gillespie GR, Greenlees MJ, Hoskin CJ, Keogh JS, Lloyd R, Melville J, McDonald PJ, Michael DR, Mitchell NJ, Sanderson C, Shea GM, Sumner J, Wapstra E, Woinarski JCZ, Chapple DG (2021) Reptiles on the brink: identifying the Australian terrestrial snake and lizard species most at risk of extinction. Pacific Conservation Biology 27, 3-12.
| Crossref | Google Scholar |
Gibbons JW, Scott DE, Ryan TJ, Buhlmann KA, Tuberville TD, Metts BS, Greene JL, Mills T, Leiden Y, Poppy S, Winne CT (2000) The global decline of reptiles, déjà vu amphibians. BioScience 50, 653-666.
| Crossref | Google Scholar |
Gillespie G, Clemann N, Hutchinson M, Michael D, Melville J, Robertson P, Chapple DG (2018) Pseudemoia rawlinsoni, The IUCN Red List of Threatened Species 2018: e.T109480985A109480994. Available at https://dx.doi.org/10.2305/IUCN.UK.2018-1.RLTS.T109480985A109480994.en [accessed 4 August 2023]
Gorissen S, Greenlees M, Shine R (2017) A skink out of water: impacts of anthropogenic disturbance on an endangered reptile in Australian highland swamps. Oryx 51, 610-618.
| Crossref | Google Scholar |
Herrero-Borgoñón JJ, Cristóbal JC, Crespo B. M (1995) Pennisetum clandestinum Hochst. ex Chiov. (Poaceae), an African grass in Europe. Israel Journal of Plant Sciences 43, 159-162.
| Crossref | Google Scholar |
Hoare JM, O’Donnell CFJ, Westbrooke I, Hodapp D, Lettink M (2009) Optimising the sampling of skinks using artificial retreats based on weather conditions and time of day. Applied Herpetology 6, 379-390.
| Crossref | Google Scholar |
Hogue AS, Breon K (2022) The greatest threats to species. Conservation Science and Practice 4, e12670.
| Crossref | Google Scholar |
House SM, Spellerberg IF (1983) Ecology and conservation of the sand lizard (Lacerta agilis L.) habitat in southern England. Journal of Applied Ecology 20, 417-437.
| Crossref | Google Scholar |
Howland BWA, Stojanovic D, Gordon IJ, Fletcher D, Snape M, Stirnemann IA, Lindenmayer DB (2016) Habitat preference of the striped legless lizard: implications of grazing by native herbivores and livestock for conservation of grassland biota. Austral Ecology 41, 455-464.
| Crossref | Google Scholar |
Huey RB, Peterson CR, Arnold SJ, Porter WP (1989) Hot rocks and not-so-hot rocks: retreat-site selection by garter snakes and its thermal consequences. Ecology 70, 931-944.
| Crossref | Google Scholar |
Huey RB, Losos JB, Moritz C (2010) Are lizards toast? Science 328, 832-833.
| Crossref | Google Scholar | PubMed |
Humphrey JE, Robert KA, Leonard SWJ (2017) Elliott traps found to be ineffective for the survey of swamp skink (Lissolepis coventryi): a cautionary tale of outdated survey guidelines. Wildlife Research 44, 514-522.
| Crossref | Google Scholar |
Hutchinson MN, Donnellan SC (1988) A new species of scincid lizard related to Leiolopisma entrecasteauxii, from southeastern Australia. Royal Society of South Australia 112, 143-151.
| Google Scholar |
Jackson DA (1993) Stopping rules in principal components analysis: a comparison of heuristical and statistical approaches. Ecology 74, 2204-2214.
| Crossref | Google Scholar |
Joppa LN, Williams CK, Temple SA, Casper GS (2009) Environmental factors affecting sampling success of artificial cover objects. Herpetological Conservation and Biology 5, 143-148.
| Google Scholar |
Kearney M, Shine R, Porter WP (2009) The potential for behavioral thermoregulation to buffer ‘cold-blooded’ animals against climate warming. Proceedings of the National Academy of Sciences 106, 3835-3840.
| Crossref | Google Scholar |
Lambin EF, Geist HJ (Eds) (2006) ‘Land-use and land-cover change.’ (Springer: Berlin, Germany). doi:10.1007/3-540-32202-7_1
Lampert A, Hastings A, Grosholz ED, Jardine SL, Sanchirico JN (2014) Optimal approaches for balancing invasive species eradication and endangered species management. Science 344, 1028-1031.
| Crossref | Google Scholar | PubMed |
Lindenmayer DB, Wood J, MacGregor C, Hobbs RJ, Catford JA (2017) Non-target impacts of weed control on birds, mammals, and reptiles. Ecosphere 8, e01804.
| Crossref | Google Scholar |
Lê S, Josse J, Husson F (2008) FactoMineR: an R package for multivariate analysis. Journal of Statistical Software 25, 1-18.
| Crossref | Google Scholar |
Martin LJ, Murray BR (2011) A predictive framework and review of the ecological impacts of exotic plant invasions on reptiles and amphibians. Biological Reviews 86, 407-419.
| Crossref | Google Scholar | PubMed |
Meiri S, Chapple DG, Tolley KA, Mitchell N, Laniado T, Cox N, Bowles P, Young BE, Caetano G, Geschke J, Böhm M, Roll U (2023) Done but not dusted: reflections on the first global reptile assessment and priorities for the second. Biological Conservation 278, 109879.
| Crossref | Google Scholar |
Michael DR, Cunningham RB, Lindenmayer DB (2011) Regrowth and revegetation in temperate Australia presents a conservation challenge for reptile fauna in agricultural landscapes. Biological Conservation 144, 407-415.
| Crossref | Google Scholar |
Mizsei E, Fejes Z, Malatinszky Á, Lengyel S, Vadász C (2020) Reptile responses to vegetation structure in a grassland restored for an endangered snake. Community Ecology 21, 203-212.
| Crossref | Google Scholar |
Moore D, Stow A, Kearney MR (2018) Under the weather? The direct effects of climate warming on a threatened desert lizard are mediated by their activity phase and burrow system. Journal of Animal Ecology 87, 660-671.
| Crossref | Google Scholar | PubMed |
Morton SR, James CD (1988) The diversity and abundance of lizards in arid Australia: a new hypothesis. The American Naturalist 132, 237-256.
| Crossref | Google Scholar |
Nugent DT, Baker-Gabb DJ, Green P, Ostendorf B, Dawlings F, Clarke RH, Morgan JW (2022) Multi-scale habitat selection by a cryptic, critically endangered grassland bird – the Plains-wanderer (Pedionomus torquatus): implications for habitat management and conservation. Austral Ecology 47, 698-712.
| Crossref | Google Scholar |
Olsson M, Shine R (2003) Female-biased natal and breeding dispersal in an alpine lizard, Niveoscincus microlepidotus. Biological Journal of the Linnean Society 79, 277-283.
| Crossref | Google Scholar |
Pimm SL, Jenkins CN, Abell R, Brooks TM, Gittleman JL, Joppa LN, Raven PH, Roberts CM, Sexton JO (2014) The biodiversity of species and their rates of extinction, distribution, and protection. Science 344, 1246752.
| Crossref | Google Scholar |
Ritchie EG, Bradshaw CJA, Dickman CR, Hobbs R, Johnson CN, Johnston EL, Laurance WF, Lindenmayer D, McCarthy MA, Nimmo DG, Possingham HH, Pressey RL, Watson DM, Woinarski J (2013) Continental-scale governance and the hastening of loss of Australia’s biodiversity. Conservation Biology 27, 1133-1135.
| Crossref | Google Scholar | PubMed |
Saleeba K, Phillips BL, O’Shea M, Kearney MR (2020) Using biophysical models to improve survey efficiency for cryptic ectotherms. The Journal of Wildlife Management 84, 1185-1195.
| Crossref | Google Scholar |
Sato CF, Wood JT, Schroder M, Green K, Michael DR, Lindenmayer DB (2014a) The impacts of ski resorts on reptiles: a natural experiment. Animal Conservation 17, 313-322.
| Crossref | Google Scholar |
Sato CF, Wood JT, Schroder M, Green K, Osborne WS, Michael DR, Lindenmayer DB (2014b) An experiment to test key hypotheses of the drivers of reptile distribution in subalpine ski resorts. Journal of Applied Ecology 51, 13-22.
| Crossref | Google Scholar |
Senior AF, Clemann N, Gardner MG, Harrisson KA, While GM, Chapple DG (2022) Genetic structure, diversity and distribution of a threatened lizard affected by widespread habitat fragmentation. Conservation Genetics 23, 151-165.
| Crossref | Google Scholar |
Sewell D, Guillera-Arroita G, Griffiths RA, Beebee TJC (2012) When is a species declining? Optimizing survey effort to detect population changes in reptiles. PLoS ONE 7, e43387.
| Crossref | Google Scholar | PubMed |
Sinclair S, Boon P (2012) Changes in the area of coastal marsh in Victoria since the mid 19th century. Cunninghamia 12, 153-176.
| Google Scholar |
Steffen W, Crutzen PJ, McNeill JR (2007) The Anthropocene: are humans now overwhelming the great forces of nature? AMBIO: A Journal of the Human Environment 36, 614-621.
| Crossref | Google Scholar | PubMed |
Todd BD, Andrews KM (2008) Response of a reptile guild to forest harvesting. Conservation Biology 22, 753-761.
| Crossref | Google Scholar | PubMed |
Tooth S, McCarthy TS (2007) Wetlands in drylands: geomorphological and sedimentological characteristics, with emphasis on examples from southern Africa. Progress in Physical Geography: Earth and Environment 31, 3-41.
| Crossref | Google Scholar |
United Nations (2022) World population prospects 2022. United Nations, Department of Economic and Social Affairs, Population Division. Available at https://population.un.org/wpp/Graphs/Probabilistic/POP/TOT/900 [accessed 3 August 2023]
Valentine LE, Roberts B, Schwarzkopf L (2007) Mechanisms driving avoidance of non-native plants by lizards. Journal of Applied Ecology 44, 228-237.
| Crossref | Google Scholar |
Victorian Environmental Assessment Council (2011) Remnant native vegetation investigation – final report. Available at https://www.veac.vic.gov.au/investigations-assessments/previous-investigations/investigation/remnant-native-vegetation-investigation-veac-2011 [accessed 3 August 2023]
Wong DT, Gruber B, Sarre SD, Osborne WS (2021) Agricultural modification to vegetation drives presence and abundance of a threatened fossorial legless lizard. Austral Ecology 46, 437-448.
| Google Scholar |
Yugovic J (2011) Ecology of the Kooweerup Swamp and associated grasslands. Proceedings of the Royal Society of Victoria 123, 172-188.
| Google Scholar |