Spatial and temporal variation in the diet of introduced sambar deer (Cervus unicolor) in an alpine landscape
Matthew J. Quin
A
B
C
Abstract
In south-eastern Australia, the abundance and distribution of non-native sambar deer (Cervus unicolor) has increased dramatically in alpine environments. As a result, significant concern surrounds the potential for the species to impact rare plant species and vegetation communities through browsing.
We aimed to determine the diversity of the plant species eaten by sambar deer in the Alpine National Park and to understand any spatial and temporal variation in deer diets.
We collected 90 sambar deer faecal pellet samples over a 3-month flowering period across two contrasting study sites with differing elevation, vegetation and underlying geology. We performed DNA sequencing using the ITS2 gene region and assigned dietary items to the lowest taxonomic level possible. The frequency of occurrence and sequencing read depth of each dietary item was calculated to investigate the diet of sambar deer at spatial and temporal scales, and dietary preferencing was assessed by comparing the frequency of occurrence of dietary items to the observation records for each dietary item in the study area.
We detected a total of 369 unique plant Operational Taxonomic Units (OTUs) from sambar deer faecal samples, representing 35 families and 80 genera. Considerable variation in the diet was observed over small spatial scales, and evidence of temporal diet variation was noted in one of the study sites. We detected Silky Snow-daisy (Celmisia sericophylla), which is currently listed as critically endangered under the Victorian Flora and Fauna Guarantee Act 1988, and Hawkweed (Pilosella spp.), a highly invasive, non-native taxon that is sparingly established in Alpine ecosystems.
Sambar deer displayed an intermediate feeder behaviour in alpine environments, foraging on a variety of forbs and shrubs, but forbs were the dominant dietary items. The spatial variation observed in the diet of sambar deer suggests that individual deer are unlikely to be dispersing widely while foraging.
Our results emphasise the need for careful evaluation of sambar deer impacts within individual sites and at small spatial scales. The detection of species of conservation significance in the diet indicates that the presence of sambar deer should be considered a significant risk to biodiversity in areas of high conservation value.
Keywords: Alpine, DNA sequencing, faecal DNA, invasive species, native species, preference, spatial variation, temporal variation.
Introduction
Globally, non-native species present significant conservation concerns due to their potentially negative impacts on native biodiversity (Wilcove et al. 1998; Chapin et al. 2000; Ricciardi 2007; Spear and Chown 2009; Nuñez et al. 2010). Non-native ungulate species have been introduced globally for a variety of purposes, including sport, hunting and food provision for humans (Griffith et al. 1989; Forsyth and Duncan 2001). In some cases, these species have established invasive populations, affecting native ecosystems through both direct and indirect pathways (Forsyth and Duncan 2001; Moriarty 2004; Pavel 2004; Suominen and Danell 2006; Foster and Scheele 2019).
Introduced deer (family Cervidae) have undergone significant global expansion over the last century (Côté et al. 2004), and in many countries have become regarded as agricultural and environmental pests (Moriarty 2004) due to their detrimental impacts on native ecosystems and the ensuing costs associated with ongoing management (Figgins and Holland 2012). Consumption of vegetation by deer can affect the growth, reproduction and long-term survival of plant species (Watson 1983; Côté et al. 2004), and preferential selection by deer is likely to cause changes to the composition of plant communities, with less preferred or more browse-tolerant species becoming dominant, a process that may also alter overall ecosystem function (Augustine and McNaughton 1998; Putman and Moore 1998; Gill and Beardall 2001; Rooney and Waller 2003; Côté et al. 2004).
In south-eastern Australia, the impact of introduced deer in alpine environments is of significant concern because these rare ecosystems occupy only 0.15% of the continent and contain species and ecological communities of national and international significance (Good 1989; Williams and Costin 1994; Venn et al. 2017). For over a century, alpine areas were used for grazing by many introduced species, including cattle (Bos taurus), sheep (Ovis aries) and horses (Equus caballus) (McDougall and Walsh 2007). Grazing and trampling by these hard-hoofed animals resulted in considerable damage to vegetation and soils (Good and Johnston 2019), leading to the practices being discontinued from high-elevation sites in Victoria in the mid-to-late 1990s, and from the Alpine National Park entirely in 2005 (Williams et al. 2006; McDougall and Walsh 2007).
Over the last decade, there has been a significant increase in the range and abundance of sambar deer (Cervus unicolor) within the Alpine National Park, with potential for the species to impact vegetation by preferential browsing, trampling and wallowing (Brown et al. 2016; Davies et al. 2020; Watter et al. 2020). The effects of browsing by sambar deer remains poorly understood within this landscape; however, the past impacts of other introduced ungulates in alpine landscapes (i.e. cattle, sheep and horses) offers clear insight into the potential consequences of inaction (Wahren et al. 1994; Driscoll et al. 2019; Robertson et al. 2019). Therefore, an investigation of the dietary components and feeding behaviour of sambar deer in high-elevation ecosystems is considered critical for effective future management of alpine landscapes in south-eastern Australia.
We analysed sambar deer faecal pellets using DNA metabarcoding techniques to assess the dietary composition of the species in the alpine landscape. Faecal samples were collected across multiple months and within several different vegetation communities at two contrasting sites to detail the taxonomic and trait diversity of the plant species that comprise the diet of sambar deer in the Alpine National Park. We pursued the following aims:
Determine whether some plant genera are preferred by sambar deer, testing the hypothesis that plant genera in the sambar deer diet differ from their availability in the landscape;
Determine whether the diet of sambar deer varies across the main alpine growth period, testing the hypothesis that sambar deer will consume different plant species at different times during the study; and
Determine whether the composition of plant species in faecal pellets varies across alpine sites and vegetation communities, testing the hypothesis that the movement patterns of sambar deer will result in faecal pellet composition that is not dependent on the site and vegetation community that those faecal pellets are collected from.
Materials and methods
Study species
Sambar deer are a large-bodied ungulate species native to the tropical and temperate ecosystems of India, southern China and south-eastern Asia (Leslie 2011), and were first introduced into south-eastern Australia in the mid-to-late 1800s (Forsyth et al. 2015). A cautious and secretive species, sambar deer are generally solitary (Harrison 1998) and prefer densely forested ecosystems, with a tendency to emerge from these areas at night for foraging (Eisenberg and Lockhart 1972). In 1941, the first reports of sambar deer in the Victorian alpine bioregion were documented at Mount Howitt, and as of 2015, sambar deer were estimated to have established wild, self-sustaining breeding populations covering approximately 29% of Victoria’s land area (Forsyth et al. 2015). Sambar deer are listed under the Flora and Fauna Guarantee Act 1988 as a ‘potentially threatening process’ to biodiversity in the state of Victoria yet are also protected as wildlife under the Victorian Wildlife Act 1975, due to their value as game species (Davis et al. 2016).
Study area
The study was conducted on the Bogong High Plains (BHP), within the Alpine National Park, approximately 240 km north-east of Melbourne, Victoria, Australia (36°50′S, 147°20′E). The BHP occupy an area of approximately 120 km2 and comprise vegetation communities ranging from woodlands, open and closed-heathlands, tussock grasslands, wetlands and snowpatch herbfields (McDougall 1982). The area experiences mean annual minimum and maximum temperatures of 2.7°C and 9.5°C respectively, and a mean annual precipitation of 2430 mm, much of which falls as snow in winter (June–August) (Lawrence 1995; Giljohann et al. 2011). The BHP are snow-covered from June to September, and the potential for ground frost is highest during the months of April, May and October. Sambar deer are the only species of deer observed on the BHP, inhabiting the area following snowmelt in late spring and remaining until the beginning of winter, when they descend to lower elevations (Downes 1983; Watter et al. 2020; Comte et al. 2022).
Two ecologically distinct sites on the BHP were selected for sampling and are separated by <10 km. The alpine Mt Nelse study site (36°50′06″S, 147°20′21″E) is 1884 m above sea level (a.s.l) and experiences long periods of late-lying snow following winter (Wahren et al. 2001). The sub-alpine Basalt Hill study site (36°53′24″S, 147°18′42″E) is 1640 m a.s.l and has been described as discordant with the rest of the BHP due to differing underlying geomorphology and plant species diversity (McDougall 1982; Wahren et al. 2001). The Basalt Hill location has a pronounced heterogenous landscape, comprising a mixture of sub-alpine vegetation communities, all in close proximity. At each of the study sites, collection area for faecal pellet samples was <5 km2.
Within each site, we concentrated on collecting samples from three different vegetation types: snowpatch herbfields, grasslands and wetlands. Snowpatch herbfields are one of the rarest vegetation types in Australia (Wahren et al. 2001; Williams et al. 2015) and are typically dominated by low growing forbs, sedges and grasses due to the shortened growing season at high elevation (Burrows 1977; Wahren et al. 2001; Venn and Morgan 2007; Williams et al. 2015). Wetland ecosystems include the Alpine Sphagnum Bogs and Associated Fens ecological community, currently listed as endangered under the Commonwealth Environment Protection and Biodiversity Conservation Act 1999. These communities are of high importance hydrologically and are particularly sensitive to grazing and trampling by ungulate species (McDougall 1982; Williams and Costin 1994). Grasslands are widespread and diverse across the alpine landscape, but high levels of deterioration within these communities has been observed due to previous grazing practices, which has resulted in the establishment of a range of invasive plants (Carr and Turner 1959; McDougall et al. 2005).
Sample collection
To assess spatial and temporal variation in sambar deer diet, faecal pellets were collected monthly (January to March), from within each of the selected vegetation types at the two study sites. Because sambar deer expel many faecal pellets in one defecation, we define a faecal pellet group as a collection of pellets that varies in the total number of individual units yet is produced by only one sambar deer. In total, 90 faecal pellet group samples were collected (n = 45 at each of the study sites, comprising 15 samples from each month across the three vegetation communities). Grasslands have a higher percentage of cover compared with wetlands on the Bogong High Plains (McDougall 2003), and snowpatch habitats are much smaller in area, occupying less than 1% of the overall alpine landscape (Wahren et al. 2001). We therefore stratified the sample collection across each month and within each site, by collecting six groups of faecal pellets from grasslands, five groups from wetlands and four groups from snowpatch herbfields. We opportunistically surveyed the same vegetation types within each site monthly and only collected fresh samples, described as those that were still brown and exhibited a wet shine with no outer decay (Mayze and Moore 1990). Pellet groups that exhibited clear signs of contamination from non-food items, such as leaf or plant tissue, were ignored. Each faecal pellet group sample consisted of approximately 10–12 faecal pellets, which were stored in a plastic zip-lock bag and placed in −30°C freezers until processing.
Plant reference library
We collected tissue samples of plant species that could be consumed by sambar deer in each of the study sites yet lacked available reference sequences on the National Centre for Biotechnology Information (NCBI) GenBank nucleotide database. We used these tissue samples to generate a plant DNA reference library. Approximately 100 mg of tissue was collected from each of the selected plant species (n = 63), and a DNeasy Plant Mini Kit (Qiagen) was used to perform DNA extractions. Plant DNA extractions were undertaken in a Polymerase Chain Reaction (PCR)-free laboratory separate to the faecal pellet extractions to prevent contamination.
Faecal DNA extraction and PCR amplification
Each faecal pellet group sample was homogenised and subsampled three times, resulting in a total of 270 samples for DNA extraction. DNA extractions were performed with ~100 mg of faecal sample using a QIAmp Fast DNA Stool Mini Kit (Qiagen) following manufacturers’ protocols and were eluted in a final volume of 200 μL. Throughout the process, negative extractions containing no faecal material were performed to monitor potential contamination.
Each DNA extraction (faecal and plant) was amplified using the ITS2 gene region of nuclear ribosomal DNA, using the established primer set ITS2-S2F and ITS4 (White et al. 1990; Chen et al. 2010). The robustness of the ITS2 gene region for analysing plant material that has undergone digestion and excretion has been highlighted by Moorhouse-Gann et al. (2018). Amplification of the ITS2 region was carried out in a final volume of 15 μL, using 2 μL of DNA extract as a template, 6.25 μL of MyTaq™ Red Mix (Bioline), 0.5 μL of each forward and reverse primer and 5.75 μL of H2O. The mixture was denatured at 94°C for 5 min, followed by 35 cycles of 30 s at 94°C, 30 s at 50°C and 45 s at 72°C. A final extension of 72°C for 10 min completed the PCR amplification stage. In cases where samples failed to amplify, DNA samples were diluted at 1/10 and 1/100 rates with H2O until amplification was successful. PCRs included both negative extraction and H2O controls to test for sample contamination.
Illumina adaptors and indexing primers were attached following the first round of PCRs, and these reactions comprised 6 μL of H2O, 6.25 μL of MyTaq™ Red Mix (Bioline), 0.75 μL of the index primer pair (Illumina) and 2 μL of DNA PCR template for a 15 μL reaction. Conditions for this PCR consisted of an initial denaturation period of 95°C for 3 min, followed by 10 cycles of denaturation at 95°C for 20 s, annealing at 60°C for 15 s and elongation at 72°C for 30 s, with a final extension step at 72°C for 3 min. PCR products were cleaned using an SPRI magnetic bead mix to remove non-specific DNA fragments. Preparation for sequencing was undertaken following the Illumina MiSeq protocol, using a MiSeq Reagent v2 (2 × 250 bp) sequencing kit.
Sequence analysis
The sequence analysis tool USEARCH v11.0.667 (Edgar 2010) was used to generate a sequence list of Operational Taxonomic Units (OTUs), which were then analysed to investigate the diet of sambar deer. The fastx_truncate function was used to remove the primers, and sequences were then filtered based on length using the fastq_filter function, to remove sequences <200 bp. Contaminants and singleton sequences were removed using the fastx_uniques function, and the -unoise3 function was used to generate OTUs. Any OTUs with fewer than three reads in a sample were removed from the dataset for analysis, and any OTU present in negative extractions and H2O controls were also discarded. After the completion of filtering, OTUs that were derived from each of three extraction subsamples were merged back into one single sample.
The final list of OTUs were first compared with our plant species reference sequence database and, if no match was found, compared with the National Centre for Biotechnology Information (NCBI) GenBank nucleotide database, using BLAST (Basic Local Alignment Search Tool; Altschul et al. 1990) with default parameters. We assigned all sequences to the lowest taxonomic level possible and considered correct assignment to species when a ≥97% match of a reference sequence to the full length of the OTU sequence was observed, following the convention that ≥97% similarity corresponds to species level (Schloss and Handelsman 2005). Likewise, ≥95% similarity was required for assignment to genus level and ≥90% for family level (Elbrecht et al. 2017). The Atlas of Living Australia (www.ala.org.au) and Royal Botanic Gardens Victoria VicFlora (www.vicflora.rbg.vic.gov.au) databases were used to ensure that plant species, genera or families identified from the OTUs had been previously recorded within or surrounding the study sites.
Statistical analysis
To determine whether the diet of sambar deer on the BHP had been sufficiently sampled, the cumulative richness of all detected OTUs, genera and families in each study site was plotted against the number of faecal samples using the ‘vegan’ package in R (R Development Core Team 2010; Oksanen et al. 2013). Differences in the number of OTUs sequenced each month were compared using a non-parametric Kruskall-Wallis H Test (Kruskal 1952).
The lowest taxonomic resolution observed for a majority of the detected OTUs was at the genus level (≥95% similarity), so much of the dietary preferencing analysis was performed at this taxonomic rank. All identified OTUs were grouped at the genus level and the frequency of occurrence was plotted for each detected genus. In accordance with Nawaz et al. (2019), we considered any genera that were present in ≥50% of all samples overall to be frequent plant dietary items, any genera that were observed in ≥10% of samples were regular dietary items and those with <10% occurrence were rare dietary items. To investigate dietary preferences, we determined if the genera detected across all samples occurred in sambar diet more or less frequently relative to their presence in the BHP plant records. Observation data were obtained from the online Atlas of Living Australia database by extracting all plant observation records within a 10-km radius from a location at the midpoint between both study sites. This midpoint included all genera available across Mt Nelse and Basalt Hill. A genus was judged as ‘preferred’ if its proportional representation across faecal samples within the study site was greater than its proportional representation in the environment as judged by ALA records (total records for target genera/total records for all genera).
To assess the feeding behaviour of sambar deer, we labelled each identified plant OTU as one of three plant growth forms (Forb, Woody, Graminoid), using information from the online Royal Botanic Gardens Victoria VicFlora database. The combined sequencing read depth of each growth form (calculated by performing the sum of all sequence reads for each OTU and grouping by growth form) across the 3 months and within each site was compared using a multivariate analysis of variance (MANOVA; O’Brien and Kaiser 1985), calculated using a Pillai trace statistic in the ‘car’ package in R (Olson 1976; Fox et al. 2007). The number of sequence reads for multiple dietary items can indicate the relative amounts of each of these items contained within a sample; therefore, sequence read depth data is used here to provide a proxy measure of overall plant biomass from each of the three plant growth forms consumed by sambar deer (Kowalczyk et al. 2011; Elbrecht and Leese 2015; Thomas et al. 2016; Deagle et al. 2019). Although the use of sequence read depth data for dietary analysis can be considered controversial due to a number of potential biases (Deagle et al. 2019), we cross-validated the results with the same analysis using cumulative OTU richness in addition to sequence read depth.
To examine the plant species composition of faecal samples among both sites and vegetation communities across different months, we performed a non-metric multi-dimensional scaling (nMDS) ordination using Bray-Curtis distance measures on presence/absence data in ‘vegan’ (Bray and Curtis 1957) and generated the ordination plot using the ‘phyloseq’ package in R (McMurdie and Holmes 2013). Stress values were used to evaluate the goodness-of-fit of the nMDS ordination, with values >0.3 considered to signify unsatisfactory representation of the data (Clarke and Warwick 2001). Analysis of similarities (ANOSIM) using ‘vegan’ was performed to test for significant differences in faecal pellet composition between sites (Mt Nelse or Basalt Hill), months (January, February or March) and vegetation communities (Snowpatch herbfield, grassland or wetland). The ‘indicspecies’ package in R was used to identify plants that were significantly associated with month, site or vegetation community, using the ‘multipatt’ function (De Cáceres 2013). The statistical significance of these associations was determined using a permutation test, with 9999 permutations.
Results
In total, 369 unique OTUs were detected in 90 faecal samples, representing 35 families and 80 genera, although only 59 dietary items could be identified to species level (Supplementary Material). At each site, the cumulative observed OTU richness approached an asymptote at a sample size of approximately 40–45 faecal samples, suggesting that a representative sample of the diet at each of the two study sites had been obtained, and that a majority of the items in the sambar deer diet had been detected (Fig. 1).
Cumulative observed richness of sambar deer dietary items at the OTU, genus and family levels, with increasing number of faecal pellet samples collected from the two sites. The shaded areas represent one standard deviation from the mean.
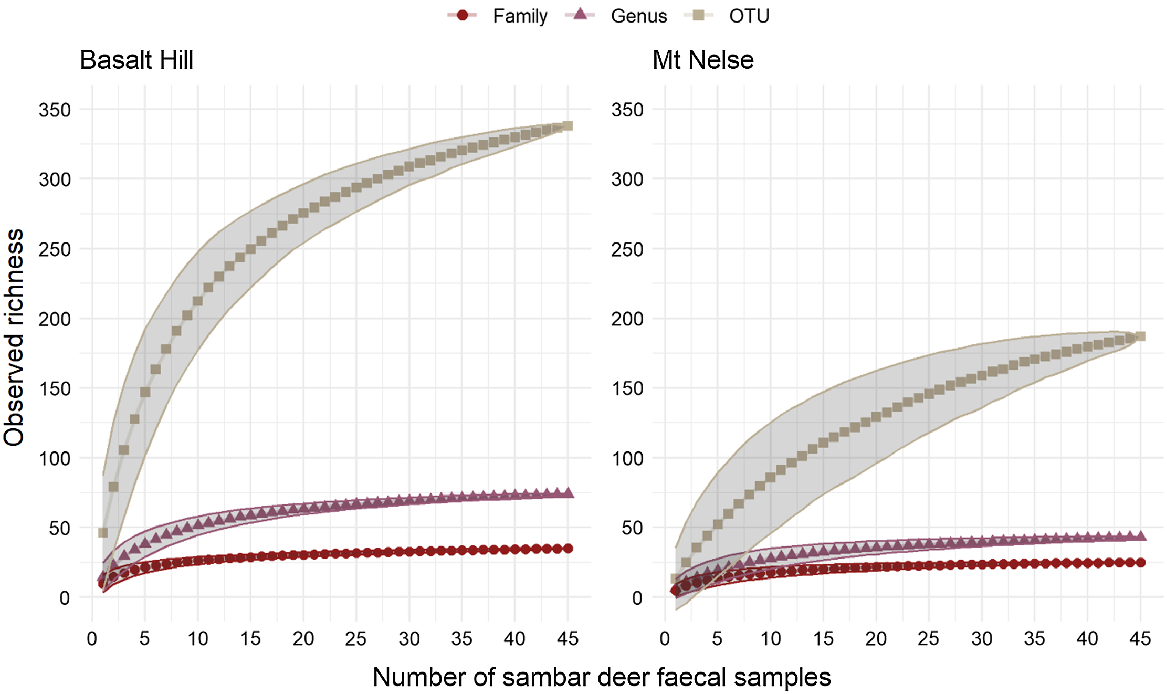
Diet and dietary preferences
The plant genera detected in the diet of sambar deer were not evenly represented – the frequency of occurrence across faecal samples ranged from 1 to 81%. Eleven plant genera were represented in only one faecal sample, and approximately 60% of all genera were found in 10 or fewer samples. The combined regular and frequent plant diet (≥10% occurrence) of sambar deer comprised 34 genera, of which 20 were forbs, 11 were shrubs and three were graminoids (Fig. 2). Forbs dominated the diet, both in terms of number of taxa detected and frequency of occurrence across faecal samples. Only three genera were noted as comprising the frequently consumed portion of the diet (≥50% occurrence): Acaena, Eucalyptus and the introduced Acetosella.
Frequency of occurrence for each genus detected in the diet of sambar deer across the two study sites on the BHP. The growth form for each genus is represented by one of three different colours. Dashed lines separate frequent, regular and rare dietary items.
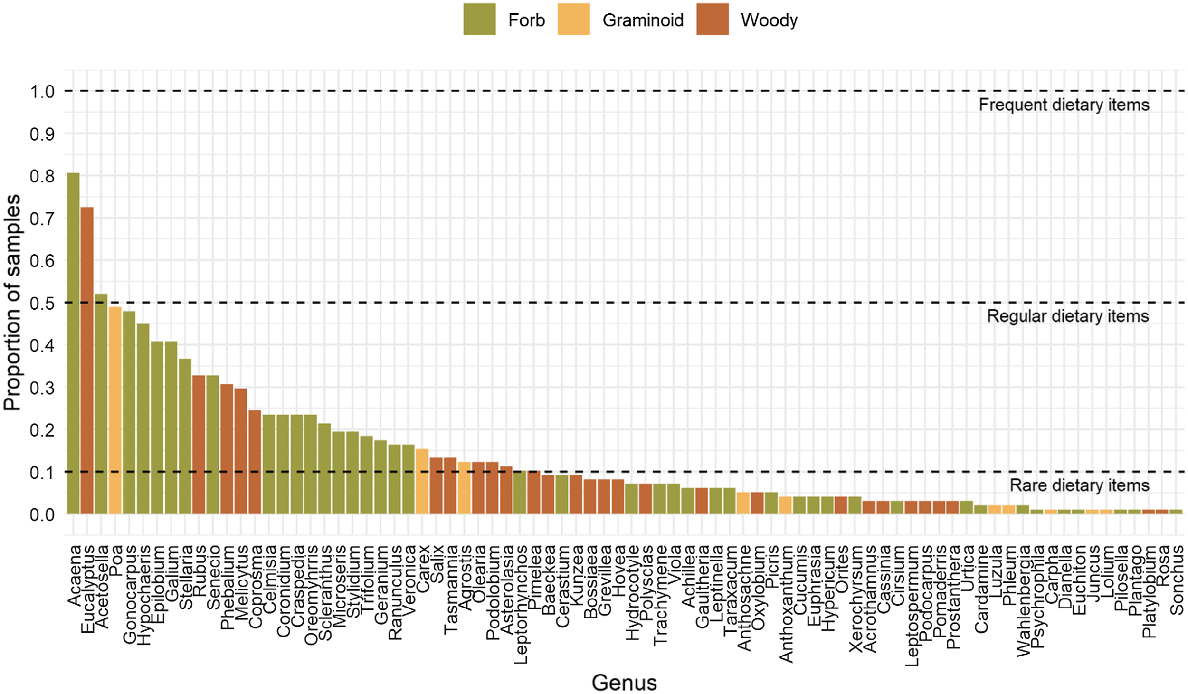
A number of genera were detected more often from faecal samples than would be expected based on plant observation records. These genera were therefore considered to be over-represented in the diet and included the native forbs Acaena, Gonocarpus, Galium and Epilobium, the native woody genus Eucalyptus and the introduced forbs Acetosella and Hypochaeris (Fig. 3). Conversely, several genera were under-represented in the diet, in comparison with their presence in plant observation records, including the most commonly observed plant genus within the entire study area (Poa) and two other graminoids (Carex, Luzula).
Relative occurrence of plant genera in 90 faecal samples and number of observations within the study area based on observations from the Atlas of Living Australia online database. The black dashed line indicates the equal detection of plants in faecal samples relative to observation counts in the landscape. Points above the line indicate higher occurrence of genera in the faecal samples than in the landscape, and points below the line indicate lower occurrence in the samples than would be predicted from recorded observations. Labels are included for the genera that were recorded in more than 400 observations and/or were detected in ≥37.5% of samples.
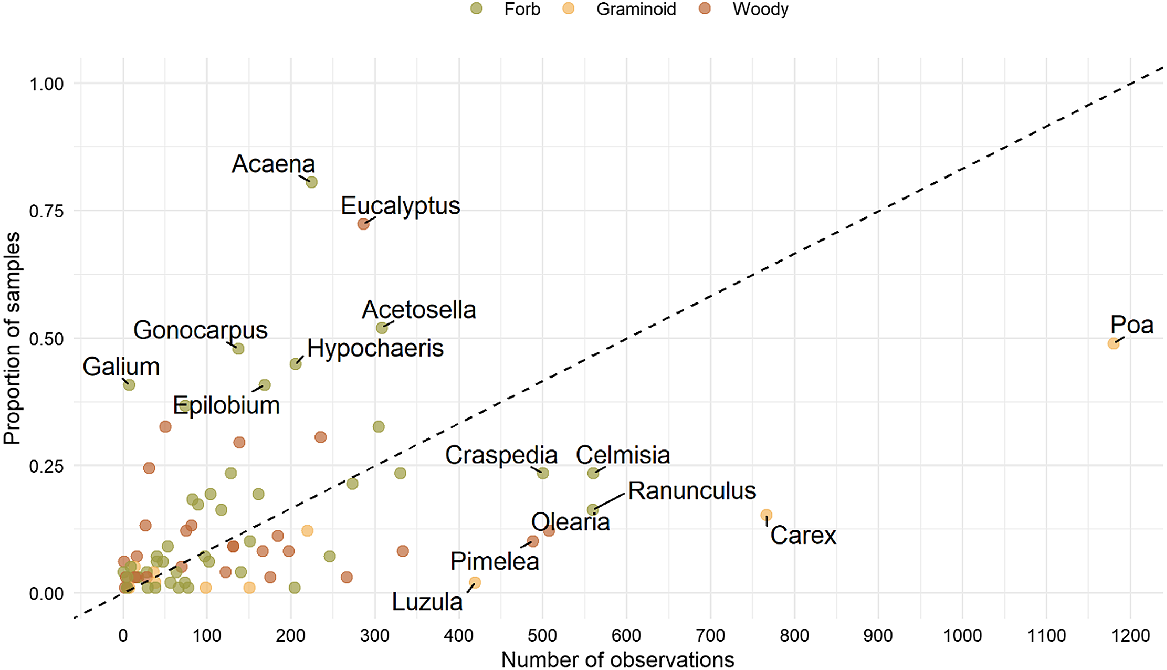
Of the OTUs that were identified to species level, the most frequently detected across all faecal samples were Acaena novae-zelandiae, Eucalyptus pauciflora, *Acetosella vulgaris, Poa costiniana, *Hypochaeris radicata and Gonocarpus montanus (asterisk here and throughout denotes non-native species). These species, as well as Coprosma hirtella, Oreomyhrris eriopoda, Scleranthus biflorus, Phebalium squamulosum subsp. ozothamnoides and *Salix cinerea, were also abundant in terms of overall sequencing read depth (each with a total read depth >5000).
Nine species that were detected in the diet are currently considered rare in the state of Victoria, (although all but one (Tasmannia vickeriana) are present in reasonable numbers in the study area). Four of these species were detected in few samples, or had low sequence read depths, suggesting that they comprise a minor component of the diet. These species were Tasmannia vickeriana (five samples, average read depth <70), Carpha nivicola (one sample, average read depth <40), Psychrophila introloba (one sample, average read depth <10) and Celmisia sericophylla (one samples, average read depth <5), which is currently listed as critically endangered under the Flora and Fauna Guarantee Act 1988. In comparison, five rare species were either detected in an increased number of samples or had high sequence read depths, suggesting that these species appear more regularly in the diet than would be expected. These species were Phebalium squamulosum subsp. ozothamnoides (29 samples, average read depth <300), Carex canescens (15 samples, average read depth <15), Ranunculus eichlerianus (10 samples, average read depth <15), Bossiaea foliosa (seven samples, average read depth <350) and Pimelea axiflora subsp. alpina (four samples, average read depth <200).
Temporal variation
There was no difference in the mean number of OTUs across the two study areas in each of the 3 months (χ2(2) = 0.828, d.f. = 2, P = 0.661), suggesting that the number of dietary items detected in the diet of sambar deer was similar throughout the study period. However, distinct monthly variation was observed in the sequence read depth of plant growth forms in Basalt Hill samples (Pillai’s trace = 0.411, F6,82 = 3.54, P < 0.01) but not in Mt Nelse (Pillai’s trace = 0.226, F6,82 = 1.743, P = 0.121; Fig. 4). These results were supported by similar analysis using cumulative OTU richness in addition to sequence read depth (Basalt Hill samples; Pillai’s trace = 0.541, F6,82 = 5.064, P < 0.01, and Mt Nelse samples; Pillai’s trace = 0.254, F6,82 = 1.993, P = 0.076). The sequence read depth of woody dietary items increased significantly in Basalt Hill samples in February (F2,42 = 5.62, P < 0.01), yet the contribution of forbs remained high across all months in the Mt Nelse samples. Additionally, we observed no difference in the sequence read depth of graminoids across the 3-month study period in Basalt Hill (F2,42 = 1.339, P = 0.271) or Mt Nelse (F2,42 = 2.685, P = 0.079).
Monthly variation in the diet of sambar deer in terms of sequence read depth of respective growth forms.
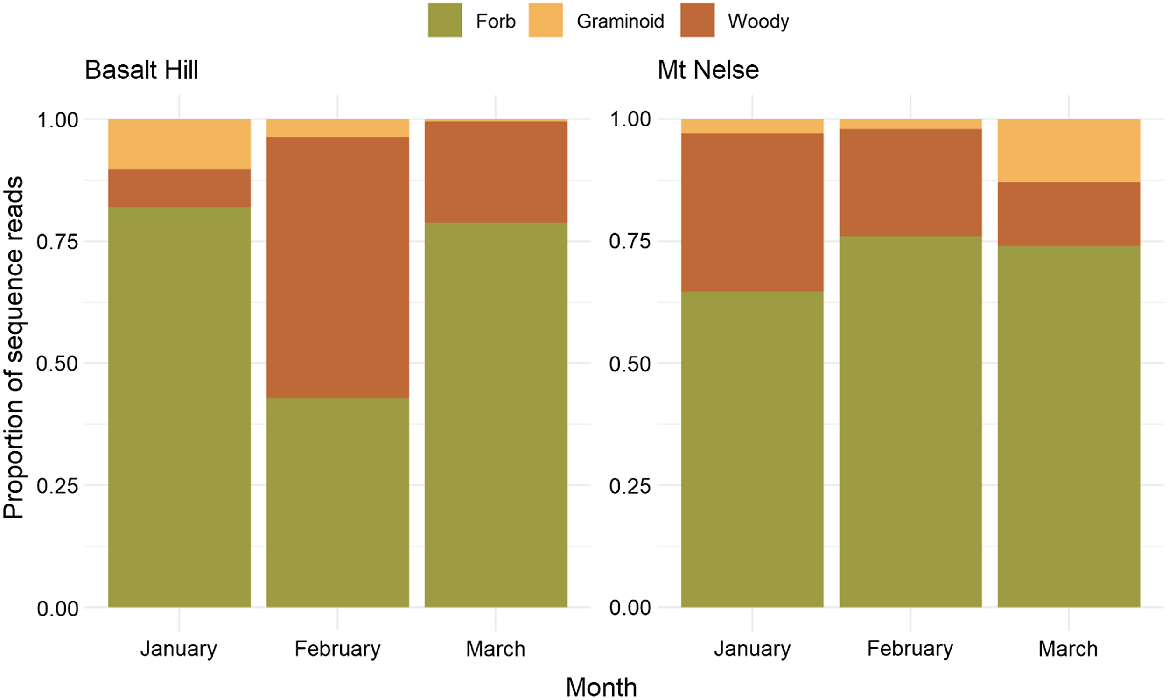
ANOSIM analysis comparing the plant species composition of faecal pellets across the 3-month sampling period resulted in no significant differences among the Mt Nelse samples (R = −0.015, P = 0.636); however, there was significant variation observed in Basalt Hill samples (R = 0.249, P = 0.001; Supplementary Material). From the indicator taxa analysis of Basalt Hill samples, the woody genus Asterolasia was more associated with February samples (statistic = 0.527, P = 0.033), and the forb genus Leptinella was more associated with March samples (statistic = 0.527, P = 0.035). The genera Eucalyptus (statistic = 0.858, P < 0.01), Gonocarpus (statistic = 0.778, P < 0.01), Phebalium (statistic = 0.761, P = 0.01) and Veronica (statistic = 0.632, P = 0.01) were significantly associated with the February and March samples, but not January. We also observed temporal variation in the plant species composition of faecal samples collected from wetland sites at Mt Nelse (R = 0.264, P = 0.022) and from grassland sites at Basalt Hill (R = 0.259, P = 0.012). Indicator taxa analysis suggests that the genera contributing to this temporal variation in wetlands at Mt Nelse were Oreomyhrris (statistic = 0.8, P = 0.049) and Scleranthus (statistic = 0.8, P = 0.049), and that the genera contributing to temporal variation in grasslands at Basalt Hill were Eucalyptus (statistic = 0.907, P = 0.019), Gonocarpus (statistic = 0.876, P < 0.01), Galium (statistic = 0.828, P = 0.023), Phebalium (statistic = 0.828, P = 0.025) and Veronica (statistic = 0.707, P = 0.044).
Spatial variation
A significant difference was observed between the plant species composition of samples from Mt Nelse and Basalt Hill (R = 0.242, P = 0.01; Fig. 5), suggesting differences in sambar deer diet between the two sites. Twelve genera were significantly more associated with samples collected from Basalt Hill: Rubus (statistic = 0.760, P < 0.01), Poa (statistic = 0.713, P < 0.01), Phebalium (statistic = 0.672, P < 0.01), Galium (statistic = 0.659, P < 0.01), Stellaria (statistic = 0.581, P < 0.01), Coprosma (statistic = 0.562, P < 0.01), Trifolium (statistic = 0.516, P < 0.01), Veronica (statistic = 0.516, P < 0.01), Agrostis (statistic = 0.494, P < 0.01), Geranium (statistic = 0.447, P < 0.01), Tasmannia (statistic = 0.447, P < 0.01) and Cerastium (statistic = 0.422, P < 0.01). Seven of the genera that were significantly associated with samples collected from Basalt Hill were only detected at this site (Agrostis, Cerastium, Geranium, Rubus, Tasmannia, Trifolium and Veronica). The remaining five genera (Coprosma, Galium, Phebalium, Poa and Stellaria) were also detected in samples from Mt Nelse, but in fewer samples compared with Basalt Hill. Although several genera were only detected in samples collected from Mt Nelse (Acrothamnus, Caltha and Lolium), each of these genera were detected in three or fewer samples, so were not significantly associated with the Mt Nelse site.
Non-metric multi-dimensional scaling (nMDS) comparison of plant species composition of sambar deer faecal pellet samples collected from Basalt Hill and Mt Nelse, using Bray–Curtis dissimilarity based on presence/absence data (stress = 0.19).
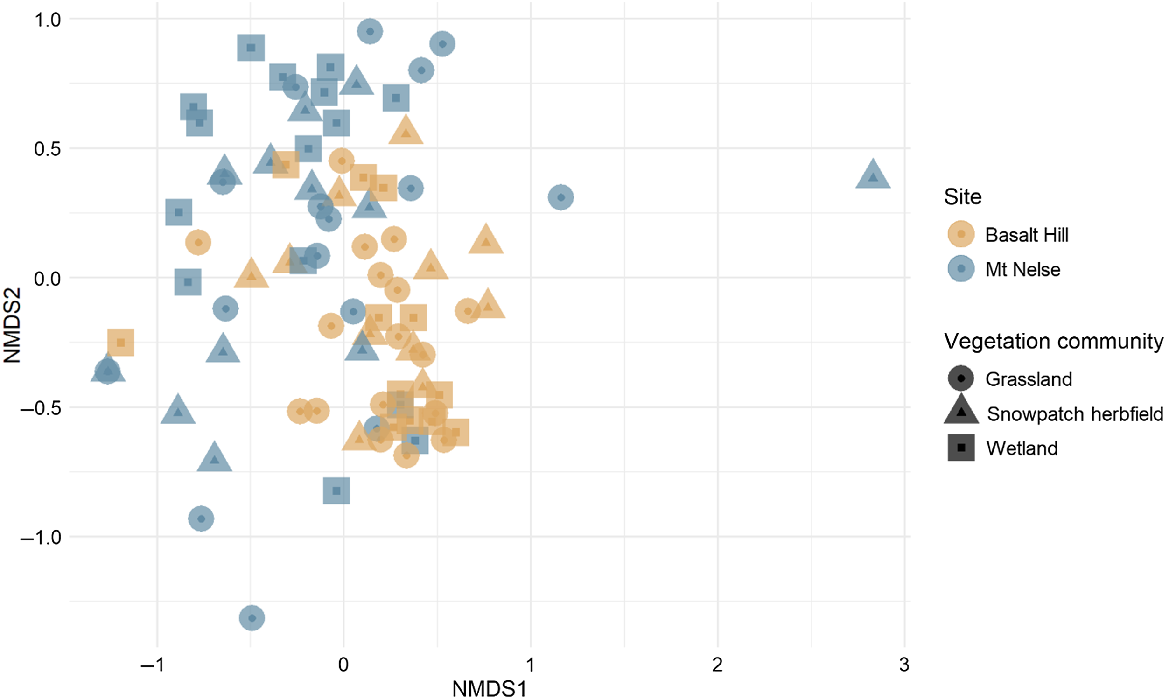
Differences were observed between sites for direct comparisons of snowpatch herbfields (R = 0.33, P = 0.001), grasslands (R = 0.237, P = 0.001) and wetlands (R = 0.212, P = 0.011). However, comparisons between vegetation communities within individual study sites resulted in non-significant results for Mt Nelse (R = 0.015, P = 0.619) and Basalt Hill (R = 0.052, P = 0.124). Additionally, comparisons among vegetation communities using all samples resulted in no difference (R = 0.009, P = 0.294), suggesting that within and among each study site, sambar deer diet contains a mixture of items from multiple vegetation communities.
Discussion
We investigated the diet of sambar deer within high-elevation sites in the Alpine National Park, where the foraging behaviours and subsequent impacts of the species remain poorly understood. Using DNA metabarcoding, the diet of sambar deer showed considerable diversity, with a total of 369 OTUs from 80 genera and 35 families identified over the 3-month sampling period. Forbs were most frequently observed in the sambar deer diet, although their importance appeared to fluctuate at one study site throughout the study period. Various plant species of conservation significance and invasive potential were detected, and preferencing of several dietary items was inferred. Notably, our results demonstrated significant spatial variation in the diet of sambar deer over a relatively small scale, which − despite the large home ranges suggested for sambar deer elsewhere − may highlight a localised feeding behaviour of the species on the BHP.
Overall diet
Although sambar deer have been described as grazers, browsers or intermediate feeders across their range (Varman and Sukumar 1993; Semiadi et al. 1995; Padmalal et al. 2003; Forsyth and Davis 2011), our study demonstrates that during the main flowering period in alpine environments, sambar deer exhibit an intermediate feeder diet mostly comprising forbs and shrubs, and they appear to adjust the composition of dietary items to adapt to food quality, quantity and availability. Forbs were the dominant dietary items, and several genera were frequently consumed by sambar deer (i.e. Acaena, Eucalyptus and *Acetosella). Other dietary studies of introduced deer in south-eastern Australia have reported similar dominance of forbs and shrubs in the diet of hog deer (Axis porcinus), red deer (Cervus elaphus) and sambar deer in a variety of environments ranging from coastal grassy woodlands to wet forests (Davis et al. 2008; Forsyth and Davis 2011; Roberts et al. 2015). In alpine environments, it is hypothesised that ungulates display opportunistic feeding behaviours due to the shortened growing season (Schaller and Gu 1994). Our results support this − the high contribution of forbs to the overall diet of sambar deer is likely a function of forb abundance in the alpine landscape (McDougall 1982). This may suggest that sambar deer are eating in response to what is locally available and therefore exhibiting feeding behaviours modified to the environments they inhabit (Hofmann and Stewart 1972). However, equally plausible is that the high contribution of forbs in the diet is also explained from a nutritional perspective, because forbs are of high nutritional quality (high metabolisable energy) and have the additional benefit of retaining their quality throughout the summer period while the quality of other plant growth forms decline (Holechek 1984; Shrestha et al. 2005; Goldberg et al. 2020).
Graminoids were not a main dietary item of sambar deer at either study site, or across the summer season. However, in Mt Nelse samples, graminoid abundance did increase towards the end of the summer season as the abundance of shrubs in diets declined. Stafford (1997) noted a dominance of grasses in the diet of sambar deer in New Zealand, a result that has been observed in other study systems (Kelton and Skipworth 1987; Padmalal et al. 2003). Although grasses, sedges and rushes represent an easily palatable and accessible supply of energy, (Jones and Wilson 1987; Frase and Armitage 1989), on the BHP, the intake of graminoids may be observed as only a minor component in the diet of sambar deer due to the availability of other, more nutritious growth forms (Goldberg et al. 2020). Therefore, it is likely that graminoids are only utilised by sambar deer to maintain energy intake and nutrient requirements when other growth forms are limited (Belovsky 1986; Frase and Armitage 1989). Further research on the diet of sambar deer outside of the summer growing season on the BHP could detail the prevalence of these growth forms during the colder months.
We detected several genera more often in faecal samples than would be expected based on their presence in the study area, including Acaena, Eucalyptus, *Acetosella, Gonocarpus, Galium, Epilobium and *Hypochaeris. The targeting of these particular plant genera suggests that sambar deer are not strictly opportunistic feeders in the Alpine National Park and are exhibiting some selective feeding behaviours towards certain plant genera. Selective feeding has the potential to alter the abundance of favoured dietary items within the landscape and is of particular concern when these items are rare or vulnerable (Kirby 2001; Keith and Pellow 2005). Currently, none of the genera detected as over-represented in the diet are considered threatened on the BHP, but their representation may help to understand movement patterns of sambar deer in alpine environments because a number of the over-represented dietary items are more common in the sub-alpine habitats, rather than the alpine sites sampled. In sub-alpine environments, sambar deer display strong crepuscular activity patterns because snow-gum woodlands and closed-heathland environments at lower elevations provide ideal habitat (i.e. cover) for the species during daylight hours (Leslie 2011; Davies et al. 2020). Considering these environments accommodate a majority of the genera observed as over-represented in the diet (McDougall 1982; Williams and Ashton 1987), it is probable that the high frequency of these dietary items is due to the movement of sambar between alpine and sub-alpine vegetation zones during active hours.
Considering the diverse range of plant material eaten by sambar deer in this study, the composition of individual faecal samples would be heavily dependent on the movement, gut passage retention times and foraging patterns of sambar deer prior to defecation (Mautz and Petrides 1971; Milne et al. 1978; Holand 1994; Gill and Beardall 2001; Pellerin et al. 2016). Therefore, as suggested by the presence of sub-alpine plant species in alpine feacal samples, the plant composition of faecal samples is unlikely to be a direct reflection of the specific vegetation community from where it was collected. Our results support the notion that sambar deer are using multiple vegetation communities for foraging, resting and defecating, and could have negative impacts through seed-dispersal of plant species into new communities and trampling of sensitive communities while browsing (Pakeman et al. 2002; Ismail and Jiwan 2015).
We did, however, observe differences between the plant species composition of faecal pellets collected from Mt Nelse and Basalt Hill, which not only suggests differences in the dietary items consumed by sambar deer that utilise these two sites, but may also suggest that sambar deer individuals are unlikely to be moving between these two areas. Mt Nelse and Basalt Hill are less than 10 km apart, which is well within home range estimates of sambar deer males and on the upper limit for females (Sankar 1994; Chundawat et al. 2007). However, despite this relatively short distance, the largely open, treeless nature of the alpine habitat between Mt Nelse and Basalt Hill may present too much of a risk for sambar deer; they are a naturally nervous and cautious species (Leslie 2011). The clear separation in dietary composition between sites suggests that localised feeding and movement behaviours are occurring on the Bogong High Plains. With the exception of sambar deer at high elevations descending to lower environments during winter, the species is typically considered non-migratory across much of its range and has a tendency to remain sedentary within locations (Schaller 1967; Leslie 2011). Further investigation utilising population level genetic techniques would provide better assessment of the movement and dispersal patterns of sambar deer within alpine landscapes and, most importantly, would aid in more effective management of current populations.
Species of conservation importance
Although many of the species detected in the diet of sambar deer are considered widespread in the environment, we detected several species of conservation significance. Rare wetland species such as Broad-leaf Flower rush (Carpha nivicola) and Alpine Marsh-marigold (Psychrophila introloba), and snowpatch species including Silky Snow-daisy (Celmisia sericophylla), which is currently listed as critically endangered under the Flora and Fauna Guarantee Act 1988, were each observed in a small number of samples. Although it appears that sambar deer are not selectively targeting these particular dietary items, the extent of browsing may only need to be minimal to have detrimental impacts on rare species (Davis et al. 2008). Therefore, it is recommended that routine monitoring of the abundance and distribution of rare plant species on the BHP is undertaken to ensure any negative impacts from sambar deer habitation is managed promptly.
We also detected Hawkweed (*Pilosella spp.) and Grey Sallow Willow (*Salix cinerea), both of which are invasive and pose serious threats to native vegetation, particularly snowpatch herbfields and wetlands (Morgan 2000; Karrenberg et al. 2002; Williams et al. 2008; Giljohann et al. 2011; Moore and Thomas 2011; Primrose et al. 2016). Although control programs exist to restrict and eradicate both species, the presence of each within the diet highlights that these species are both available in the landscape for consumption by sambar deer. Additionally, it remains possible that following consumption, sambar deer may facilitate the spread of these species through the processes of endo- or epizoochory (Albert et al. 2015).
It is possible that rare species could be widespread in the diet of sambar deer, but the DNA metabarcoding approach applied in this study may have failed to detect these dietary items due to low amounts of DNA compared with more common plant dietary item DNA (Deagle et al. 2019). Therefore, to better understand the extent of rare items in the diet, whether of conservation significance or invasive potential, a more refined method may be required. A targeted approach using species-specific primers in conjunction with real time quantitative PCR (qPCR) would be feasible for determining the presence of a single genus or species of interest in the diet of sambar deer (Harper et al. 2018) and, most importantly, could aid in tracking the distribution of rare dietary items through sambar deer faecal pellets.
Management implications
In the Alpine National Park, a long history of cattle grazing practices was discontinued in 2005 after their detrimental impacts on native vegetation, soils and water quality were highlighted (Carr 1977; Costin 1977; Van Rees 1982; Wahren et al. 1994; Williams et al. 2006). Since this time, the recovery of impacted plant species and communities has been slow (Driscoll et al. 2019). After cattle removal, sambar deer have become the primary large herbivore observed on the BHP, and our results highlight clear concerns for the management of the alpine plant species and vegetation communities in the presence of sambar deer. Although sambar deer displayed largely opportunistic feeding behaviours in this study, the preferencing of several genera highlights the ability of the species at times to be more selective with their dietary choices (Hanley 1982). The detection of rare, threatened native species and exotic weeds in the diet is of concern and should be carefully evaluated, with monitoring over a longer time period recommended where sambar deer coexist alongside plant species or communities of conservation significance.
The detection of distinct spatial and temporal variation in the diet of sambar deer within high elevation sites of the Alpine National Park provides evidence that individual deer have localised home ranges, which further emphasises the need for land managers to evaluate both the presence and potential impacts of sambar deer at small spatial scales. Further research on the population genetics of sambar deer individuals on the BHP is important, because the utilisation of advancing DNA analysis techniques for estimating population structure and dispersal characteristics would better detail the movement patterns of sambar deer within each of the study sites and, possibly, the broader Alpine National Park (Hampton et al. 2004; Brinkman and Hundertmark 2009; Davies et al. 2020).
Data availability
Data are available from the Dryad Digital Repository: https://doi.org/10.5061/dryad.rxwdbrvfz.
Acknowledgements
The authors thank Elaine Thomas, Mick Keenan, Alex Blackburn-Smith, Kurt Watter and Warren White for their help with obtaining faecal samples. A further thanks to Jude Hatley, James O’Dwyer and Erin Hill for their laboratory assistance and feedback on the manuscript. Comments from Peter Green, Ami Bennett and two anonymous reviewers greatly improved the manuscript.
References
Albert A, Auffret AG, Cosyns E, Cousins SAO, D’hondt B, Eichberg C, Eycott AE, Heinken T, Hoffmann M, Jaroszewicz B, Malo JE, Marell A, Mouissie M, Pakeman RJ, Picard M, Plue J, Poschlod P, Provoost S, Schulze KA, Baltzinger C (2015) Seed dispersal by ungulates as an ecological filter: a trait-based meta-analysis. Oikos 124(9), 1109-1120.
| Crossref | Google Scholar |
Altschul SF, Gish W, Miller W, Myers EW, Lipman DJ (1990) Basic local alignment search tool. Journal of Molecular Biology 215, 403-410.
| Crossref | Google Scholar | PubMed |
Augustine DJ, McNaughton SJ (1998) Ungulate effects on the functional species composition of plant communities: herbivore selectivity and plant tolerance. The Journal of Wildlife Management 62, 1165-1183.
| Crossref | Google Scholar |
Belovsky GE (1986) Generalist herbivore foraging and its role in competitive interactions. American Zoologist 26, 51-69.
| Crossref | Google Scholar |
Bray JR, Curtis JT (1957) An ordination of the upland forest communities of southern Wisconsin. Ecological Monographs 27(4), 325-349.
| Crossref | Google Scholar |
Brinkman TJ, Hundertmark KJ (2009) Sex identification of northern ungulates using low quality and quantity DNA. Conservation Genetics 10, 1189-1193.
| Crossref | Google Scholar |
Brown D, Thomas E, Herbert K, Primrose K (2016) Evaluating the effects of feral deer management on endangered alpine peatlands: the Alpine National Park deer control trial. Plant Protection Quarterly 31, 63-66.
| Google Scholar |
Burrows CJ (1977) Alpine grasslands and snow in the Arthur’s Pass and Lewis Pass regions, South Island, New Zealand. New Zealand Journal of Botany 15, 665-686.
| Crossref | Google Scholar |
Carr SGM, Turner JS (1959) The ecology of the Bogong High Plains. I. The environmental factors and the grassland communities. Australian Journal of Botany 7, 12-33.
| Crossref | Google Scholar |
Chapin FS, III, Zavaleta ES, Eviner VT, Naylor RL, Vitousek PM, Reynolds HL, Hooper DU, Lavorel S, Sala OE, Hobbie SE, Mack MC, Diaz S (2000) Consequences of changing biodiversity. Nature 405, 234-242.
| Crossref | Google Scholar | PubMed |
Chen S, Yao H, Han J, Liu C, Song J, Shi L, Zhu Y, Ma X, Gao T, Pang X, Luo K, Li Y, Li X, Jia X, Lin Y, Leon C (2010) Validation of the ITS2 region as a novel DNA barcode for identifying medicinal plant species. PLoS ONE 5, e8613.
| Crossref | Google Scholar | PubMed |
Comte S, Thomas E, Bengsen AJ, Bennett A, Davis NE, Freney S, Jackson SM, White M, Forsyth DM, Brown D (2022) Seasonal and daily activity of non-native sambar deer in and around high-elevation peatlands, south-eastern Australia. Wildlife Research 49, 659-672.
| Crossref | Google Scholar |
Côté SD, Rooney TP, Tremblay J-P, Dussault C, Waller DM (2004) Ecological impacts of deer overabundance. Annual Review of Ecology, Evolution, and Systematics 35, 113-147.
| Crossref | Google Scholar |
Davies C, Wright W, Hogan FE, Davies H (2020) Detectability and activity patterns of sambar deer (Rusa unicolor) in Baw Baw National Park, Victoria. Australian Mammalogy 42, 312-320.
| Crossref | Google Scholar |
Davis NE, Coulson G, Forsyth DM (2008) Diets of native and introduced mammalian herbivores in shrub-encroached grassy woodland, south-eastern Australia. Wildlife Research 35, 684-694.
| Crossref | Google Scholar |
Davis NE, Bennett A, Forsyth DM, Bowman DMJS, Lefroy EC, Wood SW, Woolnough AP, West P, Hampton JO, Johnson CN (2016) A systematic review of the impacts and management of introduced deer (family Cervidae) in Australia. Wildlife Research 43, 515-532.
| Crossref | Google Scholar |
De Cáceres M (2013) How to use the indicspecies package (ver. 1.7. 1). R Proj 29,.
| Google Scholar |
Deagle BE, Thomas AC, McInnes JC, Clarke LJ, Vesterinen EJ, Clare EL, Kartzinel TR, Eveson JP (2019) Counting with DNA in metabarcoding studies: how should we convert sequence reads to dietary data? Molecular Ecology 28, 391-406.
| Crossref | Google Scholar | PubMed |
Driscoll DA, Worboys GL, Allan H, Banks SC, Beeton NJ, Cherubin RC, Doherty TS, Finlayson CM, Green K, Hartley R, Hope G, Johnson CN, Lintermans M, Mackey B, Paull DJ, Pittock J, Porfirio LL, Ritchie EG, Sato CF, Scheele BC, Slattery DA, Venn S, Watson D, Watson M, Williams RM (2019) Impacts of feral horses in the Australian Alps and evidence-based solutions. Ecological Management & Restoration 20, 63-72.
| Crossref | Google Scholar |
Edgar RC (2010) Search and clustering orders of magnitude faster than BLAST. Bioinformatics 26, 2460-2461.
| Crossref | Google Scholar | PubMed |
Elbrecht V, Leese F (2015) Can DNA-based ecosystem assessments quantify species abundance? Testing primer bias and biomass – sequence relationships with an innovative metabarcoding protocol. PLos ONE 10, e0130324.
| Crossref | Google Scholar | PubMed |
Elbrecht V, Vamos EE, Meissner K, Aroviita J, Leese F (2017) Assessing strengths and weaknesses of DNA metabarcoding-based macroinvertebrate identification for routine stream monitoring. Methods in Ecology and Evolution 8, 1265-1275.
| Crossref | Google Scholar |
Figgins G, Holland P (2012) Red deer in New Zealand: game animal, economic resource or environmental pest? New Zealand Geographer 68, 36-48.
| Crossref | Google Scholar |
Forsyth DM, Davis NE (2011) Diets of non-native deer in Australia estimated by macroscopic versus microhistological rumen analysis. The Journal of Wildlife Management 75, 1488-1497.
| Crossref | Google Scholar |
Forsyth DM, Duncan RP (2001) Propagule size and the relative success of exotic ungulate and bird introductions to New Zealand. The American Naturalist 157, 583-595.
| Crossref | Google Scholar | PubMed |
Foster CN, Scheele BC (2019) Feral-horse impacts on corroboree frog habitat in the Australian Alps. Wildlife Research 46, 184-190.
| Crossref | Google Scholar |
Fox J, Friendly GG, Graves S, Heiberger R, Monette G, Nilsson H, Ripley B, Weisberg S, Fox MJ, Suggests MASS (2007) The car package. R Foundation for Statistical Computing 1109, 1431.
| Google Scholar |
Frase BA, Armitage KB (1989) Yellow-bellied marmots are generalist herbivores. Ethology Ecology & Evolution 1, 353-366.
| Crossref | Google Scholar |
Giljohann KM, Hauser CE, Williams NSG, Moore JL (2011) Optimizing invasive species control across space: willow invasion management in the Australian Alps. Journal of Applied Ecology 48, 1286-1294.
| Crossref | Google Scholar |
Gill RMA, Beardall V (2001) The impact of deer on woodlands: the effects of browsing and seed dispersal on vegetation structure and composition. Forestry: An International Journal of Forest Research 74, 209-218.
| Crossref | Google Scholar |
Goldberg AR, Conway CJ, Tank DC, Andrews KR, Gour DS, Waits LP (2020) Diet of a rare herbivore based on DNA metabarcoding of feces: selection, seasonality, and survival. Ecology and Evolution 10, 7627-7643.
| Crossref | Google Scholar | PubMed |
Good R, Johnston S (2019) Rehabilitation and revegetation of the Kosciuszko summit area, following the removal of grazing – an historic review. Ecological Management & Restoration 20, 13-20.
| Crossref | Google Scholar |
Griffith B, Scott JM, Carpenter JW, Reed C (1989) Translocation as a species conservation tool: status and strategy. Science 245, 477-480.
| Crossref | Google Scholar | PubMed |
Hampton JO, Spencer PBS, Alpers DL, Twigg LE, Woolnough AP, Doust J, Higgs T, Pluske J (2004) Molecular techniques, wildlife management and the importance of genetic population structure and dispersal: a case study with feral pigs. Journal of Applied Ecology 41, 735-743.
| Crossref | Google Scholar |
Hanley TA (1982) The nutritional basis for food selection by ungulates. Journal of Range Management 35, 146-151.
| Crossref | Google Scholar |
Harper LR, Lawson Handley L, Hahn C, Boonham N, Rees HC, Gough KC, Lewis E, Adams IP, Brotherton P, Phillips S, Hänfling B (2018) Needle in a haystack? A comparison of eDNA metabarcoding and targeted qPCR for detection of the great crested newt (Triturus cristatus). Ecology and Evolution 8, 6330-6341.
| Crossref | Google Scholar | PubMed |
Hofmann RR, Stewart DRM (1972) Grazer or browser: a classification based on the stomach-structure and feeding habits of East African ruminants. Mammalia 36, 226-240.
| Crossref | Google Scholar |
Holand Ø (1994) Seasonal dynamics of digestion in relation to diet quality and intake in European roe deer (Capreolus capreolus). Oecologia 98, 274-279.
| Crossref | Google Scholar | PubMed |
Holechek JL (1984) Comparative contribution of grasses, forbs, and shrubs to the nutrition of range ungulates. Rangelands Archives 6, 261-263.
| Google Scholar |
Ismail D, Jiwan D (2015) Browsing preference and ecological carrying capacity of sambar deer (Cervus unicolor brookei) on secondary vegetation in forest plantation. Animal Science Journal 86, 225-237.
| Crossref | Google Scholar | PubMed |
Karrenberg S, Kollmann J, Edwards PJ (2002) Pollen vectors and inflorescence morphology in four species of Salix. Plant Systematics and Evolution 235, 181-188.
| Crossref | Google Scholar |
Keith D, Pellow B (2005) Effects of Javan rusa deer (Cervus timorensis) on native plant species in the Jibbon-Bundeena area, Royal National Park, New South Wales. Proceedings of the Linnean Society of New South Wales 126, 99-110.
| Google Scholar |
Kelton SD, Skipworth JP (1987) Food of sambar deer (Cervus unicolor) in a Manawatu (New Zealand) flax swamp. New Zealand Journal of Ecology 10, 149-152.
| Google Scholar |
Kirby KJ (2001) The impact of deer on the ground flora of British broadleaved woodland. Forestry 74, 219-229.
| Crossref | Google Scholar |
Kowalczyk RI, Taberlet P, Coissac E, Valentini A, Miquel C, Kamiński T, Wójcik JM (2011) Influence of management practices on large herbivore diet – case of European bison in Białowieża Primeval Forest (Poland). Forest Ecology and Management 261, 821-828.
| Crossref | Google Scholar |
Kruskal WH (1952) A nonparametric test for the several sample problem. The Annals of Mathematical Statistics 23, 525-540.
| Crossref | Google Scholar |
Lawrence RE (1995) The effects of grazing activity on the hydrology of the Bogong High Plains, Australia. The Rangeland Journal 17, 138-153.
| Crossref | Google Scholar |
Leslie DM, Jr (2011) Rusa unicolor (Artiodactyla: Cervidae). Mammalian Species 43, 1-30.
| Crossref | Google Scholar |
Mautz WW, Petrides GA (1971) Food passage rate in the white-tailed deer. The Journal of Wildlife Management 35, 723-731.
| Crossref | Google Scholar |
McDougall KL (2003) Aerial photographic interpretation of vegetation changes on the Bogong High Plains, Victoria, between 1936 and 1980. Australian Journal of Botany 51, 251-256.
| Crossref | Google Scholar |
McDougall KL, Walsh NG (2007) Treeless vegetation of the Australian Alps. Cunninghamia 10, 1-57.
| Google Scholar |
McDougall KL, Morgan JW, Walsh NG, Williams RJ (2005) Plant invasions in treeless vegetation of the Australian Alps. Perspectives in Plant Ecology, Evolution and Systematics 7, 159-171.
| Crossref | Google Scholar |
McMurdie PJ, Holmes S (2013) phyloseq: an R package for reproducible interactive analysis and graphics of microbiome census data. PLoS ONE 8, e61217.
| Crossref | Google Scholar |
Milne JA, Macrae JC, Spence AM, Wilson S (1978) A comparison of the voluntary intake and digestion of a range of forages at different times of the year by the sheep and the red deer (Cervus elaphus). British Journal of Nutrition 40, 347-357.
| Crossref | Google Scholar | PubMed |
Moore J, Thomas E (2011) Fighting the willow menace on the Bogong High Plains. Park Watch 245, 8-9.
| Google Scholar |
Moorhouse-Gann RJ, Dunn JC, de Vere N, Goder M, Cole N, Hipperson H, Symondson WOC (2018) New universal ITS2 primers for high-resolution herbivory analyses using DNA metabarcoding in both tropical and temperate zones. Scientific Reports 8, 8542.
| Crossref | Google Scholar |
Morgan JW (2000) Orange Hawkweed Hieracium aurantiacum L.: a new naturalised species in alpine Australia. Victorian Naturalist 117, 50-51.
| Google Scholar |
Moriarty A (2004) The liberation, distribution, abundance and management of wild deer in Australia. Wildlife Research 31, 291-299.
| Crossref | Google Scholar |
Nawaz MA, Valentini A, Khan NK, Miquel C, Taberlet P, Swenson JE (2019) Diet of the brown bear in Himalaya: combining classical and molecular genetic techniques. PLoS ONE 14, e0225698.
| Crossref | Google Scholar | PubMed |
Nuñez MA, Bailey JK, Schweitzer JA (2010) Population, community and ecosystem effects of exotic herbivores: a growing global concern. Biological Invasions 12, 297-301.
| Crossref | Google Scholar |
Olson CL (1976) On choosing a test statistic in multivariate analysis of variance. Psychological Bulletin 83, 579-586.
| Crossref | Google Scholar |
O’Brien RG, Kaiser MK (1985) MANOVA method for analyzing repeated measures designs: an extensive primer. Psychological Bulletin 97, 316-333.
| Google Scholar | PubMed |
Padmalal UKGK, Takatsuki S, Jayasekara P (2003) Food habits of sambar Cervus unicolor at the Horton Plains National Park, Sri Lanka. Ecological Research 18, 775-782.
| Crossref | Google Scholar |
Pakeman RJ, Digneffe G, Small JL (2002) Ecological correlates of endozoochory by herbivores. Functional Ecology 16, 296-304.
| Crossref | Google Scholar |
Pavel V (2004) The impact of grazing animals on nesting success of grassland passerines in farmland and natural habitats: a field experiment. Folia Zoologica 53, 171-178.
| Google Scholar |
Pellerin M, Picard M, Saïd S, Baubet E, Baltzinger C (2016) Complementary endozoochorous long-distance seed dispersal by three native herbivorous ungulates in Europe. Basic and Applied Ecology 17, 321-332.
| Crossref | Google Scholar |
Primrose K, Constantine A, Smith N, Pascoe C (2016) Eradicating hawkweeds (Hieracium spp.) from the Victorian Alps: improving the efficiency and effectiveness of control whilst mitigating off-target impacts. Plant Protection Quarterly 31, 33-37.
| Google Scholar |
Putman RJ, Moore NP (1998) Impact of deer in lowland Britain on agriculture, forestry and conservation habitats. Mammal Review 28, 141-164.
| Crossref | Google Scholar |
R Development Core Team (2010) R: a language and environment for statistical computing. Computer Programme. Available at http://www.R-project.org/
Ricciardi A (2007) Are modern biological invasions an unprecedented form of global change? Conservation Biology 21, 329-336.
| Crossref | Google Scholar |
Roberts C, Westbrooke M, Florentine S, Cook S (2015) Winter diet of introduced red deer (Cervus elaphus) in woodland vegetation in Grampians National Park, western Victoria. Australian Mammalogy 37, 107-112.
| Crossref | Google Scholar |
Robertson G, Wright J, Brown D, Yuen K, Tongway D (2019) An assessment of feral horse impacts on treeless drainage lines in the Australian Alps. Ecological Management & Restoration 20, 21-30.
| Crossref | Google Scholar |
Rooney TP, Waller DM (2003) Direct and indirect effects of white-tailed deer in forest ecosystems. Forest Ecology and Management 181, 165-176.
| Crossref | Google Scholar |
Schaller GB, Gu B (1994) Comparative ecology of ungulates in the Aru basin of northwest Tibet. National Geographic Research and Exploration 10, 266-293.
| Google Scholar |
Schloss PD, Handelsman J (2005) Introducing DOTUR, a computer program for defining operational taxonomic units and estimating species richness. Applied and Environmental Microbiology 71, 1501-1506.
| Crossref | Google Scholar | PubMed |
Semiadi G, Barry TN, Muir PD, Hodgson J (1995) Dietary preferences of sambar (Cervus unicolor) and red deer (Cervus elaphus) offered browse, forage legume and grass species. The Journal of Agricultural Science 125, 99-107.
| Crossref | Google Scholar |
Shrestha R, Wegge P, Koirala RA (2005) Summer diets of wild and domestic ungulates in Nepal Himalaya. Journal of Zoology 266, 111-119.
| Crossref | Google Scholar |
Spear D, Chown SL (2009) Non-indigenous ungulates as a threat to biodiversity. Journal of Zoology 279, 1-17.
| Crossref | Google Scholar |
Stafford KJ (1997) The diet and trace element status of sambar deer (Cervus unicolor) in Manawatu district, New Zealand. New Zealand Journal of Zoology 24, 267-271.
| Crossref | Google Scholar |
Thomas AC, Deagle BE, Eveson JP, Harsch CH, Trites AW (2016) Quantitative DNA metabarcoding: improved estimates of species proportional biomass using correction factors derived from control material. Molecular Ecology Resources 16, 714-726.
| Crossref | Google Scholar | PubMed |
van Rees H (1982) The diet of free-ranging cattle on the Bogong High Plains, Victoria. The Rangeland Journal 4, 29-33.
| Crossref | Google Scholar |
Venn SE, Morgan JW (2007) Phytomass and phenology of three alpine snowpatch species across a natural snowmelt gradient. Australian Journal of Botany 55, 450-456.
| Crossref | Google Scholar |
Wahren CHA, Papst WA, Williams RJ (1994) Long-term vegetation change in relation to cattle grazing in sub-alpine grassland and heathland on the Bogong High-Plains: an analysis of vegetation records from 1945 to 1994. Australian Journal of Botany 42, 607-639.
| Crossref | Google Scholar |
Wahren CH, Williams RJ, Papst WA (2001) Alpine and subalpine snow patch vegetation on the Bogong High Plains, SE Australia. Journal of Vegetation Science 12, 779-790.
| Crossref | Google Scholar |
Watson A (1983) Eighteenth century deer numbers and pine regeneration near Braemar, Scotland. Biological Conservation 25, 289-305.
| Crossref | Google Scholar |
Watter K, Thomas E, White N, Finch N, Murray PJ (2020) Reproductive seasonality and rate of increase of wild sambar deer (Rusa unicolor) in a new environment, Victoria, Australia. Animal Reproduction Science 223, 106630.
| Crossref | Google Scholar | PubMed |
Wilcove DS, Rothstein D, Dubow J, Phillips A, Losos E (1998) Quantifying threats to imperiled species in the United States. BioScience 48, 607-615.
| Crossref | Google Scholar |
Williams RJ, Ashton DH (1987) Effects of disturbance and grazing by cattle on the dynamics of heathland and grassland communities on the Bogong High Plains, Victoria. Australian Journal of Botany 35, 413-431.
| Crossref | Google Scholar |
Williams RJ, Wahren CH, Bradstock RA, Müller WJ (2006) Does alpine grazing reduce blazing? A landscape test of a widely-held hypothesis. Austral Ecology 31, 925-936.
| Crossref | Google Scholar |
Williams SC, Ward JS, Ramakrishnan U (2008) Endozoochory by white-tailed deer (Odocoileus virginianus) across a suburban/woodland interface. Forest Ecology and Management 255, 940-947.
| Crossref | Google Scholar |
Williams RJ, Wahren CH, Stott KAJ, Camac JS, White M, Burns E, Harris S, Nash M, Morgan JW, Venn S, Papst WA, Hoffmann AA (2015) An International Union for the Conservation of Nature Red List ecosystems risk assessment for alpine snow patch herbfields, South-Eastern Australia. Austral Ecology 40, 433-443.
| Crossref | Google Scholar |