The toad less travelled: comparing life histories, ecological niches, and potential habitat of Asian black-spined toads and cane toads
C. L. Kelly

A Department of Agriculture and Fisheries, 203 Tor Street, Toowoomba, Qld 4350, Australia.
B College of Science and Engineering, James Cook University, Townsville, Qld 4814, Australia.
C Department of Primary Industries and Regional Development, 3 Baron-Hay Court, South Perth, WA 6151, Australia.
Abstract
Invasive vertebrates have significant negative impacts on biodiversity and agricultural production worldwide. Increased connectivity among countries, through trade and tourism, is escalating the rate of introductions of vertebrate species, particularly herpetofauna, across international borders. In Australia, Asian black-spined toads (ABSTs; Duttaphrynus melanostictus) are one of the species most intercepted at borders. They are considered a biosecurity risk because of the potential for negative environmental impacts, similar to those caused by cane toads (Rhinella marina).
We aimed to compare ABSTs with cane toads to investigate potential impacts and distribution of ABSTs in the Australian context. We also aimed to identify knowledge gaps regarding ABST biology and the potential role of cane toads in an ABST invasion in Australia.
We undertook a literature review to obtain published data to compare the life history characteristics of ABSTs and cane toads. We also modelled climatic niche overlap and compared suitable habitat for both species in Australia.
Our results show ABSTs and cane toads have broadly similar reproductive life histories and feeding niches. In particular, similarities include large clutch sizes, preferred oviposition sites, and diet at tadpole and adult life stages. In Australia, the species share suitable potential habitat, particularly in North Queensland, where the majority of ABST incursions have occurred. The species differ in size, call characteristics, clutch size relative to body size, and egg development rate, although the environment also influences these traits. We identify gaps in our knowledge of ABST spatial ecology, thermal tolerances, water reliance, and habitat.
ABSTs pose a significant biosecurity threat to Australia. Similarities in life history to cane toads means they may have similar impacts, but may have a more limited distribution in Australia. Invasion of Australia by ABSTs would likely result in interactions with cane toads, but it is not possible to accurately determine the outcomes of those interactions without further investigation.
Addressing knowledge gaps and quantitatively determining the potential for competition between ABSTs and cane toads will assist surveillance and response planning for ABST incursions in Australia.
Keywords: Asian black-spined toad, cane toad, competition, Duttaphrynus melanostictus, incursion, invasion, life history, niche overlap, species distribution modelling.
Introduction
Invasive vertebrates contribute significantly to degradation of global biodiversity and have negative impacts on agricultural production and social amenity (Forsyth et al. 2004; Gong et al. 2009; Roberts et al. 2013; Simberloff et al. 2013). Greater connectivity among countries through trade and tourism has increased the rate of new species incursions globally (Lockwood et al. 2019; Toomes et al. 2020). In Australia, invasive vertebrates are a key driver of extinctions (Woinarski et al. 2019), are among the most prevalent threat to native threatened vertebrates (Kearney et al. 2019), and have economic impacts of A$13.6B per year (Gong et al. 2009; Saunders et al. 2010; Hoffmann and Broadhurst 2016).
Various traits, including characteristics of the native range, history of establishment after introduction, diet, and habitat requirements have been used to infer the likely success of a novel species following introduction (Toomes et al. 2020). Species that share similar ecological traits or niches should respond similarly to environmental conditions (Thuiller et al. 2005; Yu et al. 2017). Consequently, examining the characteristics of related or ecologically analogous species has been proposed as one method of predicting an introduced species’ impact (Byers et al. 2002; Negussie et al. 2013). Although, in addition to biotic features of the invading species, successful invasion can be influenced by biotic (e.g. predators, competitors) and abiotic (e.g. resource availability) features of the new environment, propagule pressure, and human activity (e.g. alteration of environmental conditions) and their interactions (Catford et al. 2009).
Vertebrate incursions can occur along multiple pathways, including deliberate release, escape from the legal and illegal pet trade, and unintentional stowaways (Christy et al. 2007; Hulme 2015). In Australia, herpetofauna are the most frequently detected stowaways (Henderson and Bomford 2011; García-Díaz and Cassey 2014; Toomes et al. 2020). One species of particular concern is the Asian black-spined toad (ABST; Duttaphrynus melanostictus). ABSTs have been assessed as a ‘Serious’ establishment risk to Australia based on their habitat requirements, invasion history, and biotic traits (Bomford 2006). They are also the most commonly detected vertebrate stowaway at the Australian border, and detection rates are increasing (Tingley et al. 2018; Toomes et al. 2020).
Although ABSTs have yet not established in Australia, another Bufonid, the cane toad (Rhinella marina), has. Often, the potential impacts of ABSTs in Australia are considered likely to be similar to those of cane toads (Mo 2017). Cane toads provide a comparison to investigate potential impacts and spread of ABST in the Australian context and could be used as a proxy to facilitate development of surveillance and response tools for ABST incursions into Australia (Tingley et al. 2018; Christy 2020; Andersen et al. 2021). However, the life history of ABSTs is considerably less studied than that of cane toads, and there may be consequential differences between the two species. Further, the presence of cane toads in Australia could possibly influence the ability of ABST to establish and spread in Australian ecosystems through interspecific competition. Here we review the available literature to identify life history attributes of ABSTs and compare them with cane toads. We also quantify niche similarity and Australian habitat suitability for the two species, identify knowledge gaps about ABSTs, and consider the role of cane toads in a possible ABST invasion.
Methods
Species
Both ABSTs and cane toads have extensive native ranges (6.9 million km2 and 9.7 million km2 respectively), with widespread distributions on both continents and archipelagos (van Dijk et al. 2004; Solís et al. 2009). ABSTs are a species complex of three distinct genetic lineages (Wogan et al. 2016), and their native distribution extends throughout south Asia (Othman et al. 2020). Invasive populations established in Bali in approximately 1958 and invaded many of the islands of Wallacea, West Papua in 2007 and Madagascar in approximately 2010 (Church 1960; Trainor 2009; Reilly et al. 2017; Vences et al. 2017).
The native range of cane toads has been considered to extend from southern Texas through to Brazil (Solís et al. 2009). Outside of their native range, they have become established more broadly than ABST, including New Guinea, Jamaica, Puerto Rico, Bermuda, Hawai’i, Taiwan, Florida, and Fiji (Easteal 1981; Lever 2001). Cane toads were introduced to Australia in 1935 as biocontrol for two major pests, the greyback beetle (Dermolepida albohirtum) and the Frenchi beetle (Lepidiota frenchi) (Mungomery and Buzacott 1936). Following establishment, cane toads increased geographic range, causing significant environmental impacts (Boland 2004; Greenlees et al. 2006; Letnic et al. 2008; Crossland et al. 2009; Price-Rees et al. 2010; Bleach et al. 2015). Recent genetic and morphological evaluation of cane toads has identified two species (R. marina and R. horribilis) occurring within the range historically attributed to cane toads (Acevedo et al. 2016). Cane toads in Australia are R. marina (Slade and Moritz 1998). However, because the literature relating to cane toads does not distinguish between R. marina and R. horribilis prior to 2016, and providence of populations in all invaded areas globally is not known (Sales et al. 2021), here we consider R. marina and R. horribilis together (sensu Sales et al. 2021).
Literature review
The literature on the biology and ecology of ABSTs was reviewed by Christy (2020). We undertook a literature review of the biology of both cane toads and ABSTs using the online database Google Scholar to identify peer-reviewed publications and grey literature on the biology and ecology of both species. This online review was completed on 19 August 2021. In addition to online searches, we examined the reference lists of papers found in the search for additional works. For each species, we sought published data on morphology, life cycle, reproduction, habitat, diet, toxin, spatial ecology, thermal thresholds, and water requirements, and call auditory characteristics. Search terms included, but were not limited to, ‘Asian black-spined toad’ and ‘cane toad’, and their respective Latin names, followed by ‘ecology’, ‘invasion’, ‘morphology’, and ‘physiology’, etc. Where no, or limited, published data were available for ABSTs, we recorded these areas as not currently documented.
Niche overlap
Species presence data for ABSTs and cane toads were obtained from open access databases, and ABST records were supplemented with recent records from Madagascar (Licata et al. 2021; Licata and Crottini, unpubl. data). Native range and international occurrence records were collected from Global Biodiversity Information Facility (GBIF; https://www.gbif.org) and Australian occurrence records from Atlas of Living Australia (ALA; https://www.ala.org.au). We first filtered out records with an accuracy greater than 1 km2, to ensure we were using as precise a dataset as possible. We then removed multiples of records within 1-km2 cells. This produced 15 001 records for cane toads and 11 311 for ABSTs. To model climatic niche (i.e. the ecological niche using only bioclimatic variables), we obtained bioclimatic variables from WorldClim (https://www.worldclim.org).
To produce measures of climatic niche overlap between ABSTs and cane toads, we used methods and an R script developed by Di Cola et al. (2017) using the ecospat package (Broennimann et al. 2021). We produced two niche overlap plots: one comparing the native climatic niches of ABSTs and cane toads, and the other comparing their total bioclimatic niches (including invaded ranges). From there, we removed highly correlated bioclimatic variables (Pearson correlation coefficient greater than 0.75), leaving us with 10 bioclimatic variables (as indicated in Supplementary Table S1).
To determine climatic overlap in the ranges of the two species, we used the occurrence records and WorldClim layers, and calculated the contribution of each bioclimatic variable as a function of the density of the records. We then used a principal component analysis (PCA) to compare the pixel values of the bioclimatic variables between the species distributions, as outlined in Broennimann et al. (2012) and Di Cola et al. (2017). These PCA scores were projected onto a grid, whereon an occurrence density grid was projected for both species, in their respective native and total ranges.
The observed niche overlap scores were calculated using Schoener’s D, which varies from 0 (complete dissimilarity) to 1 (complete overlap). We created a null model for niche similarity between the two sets of compared ranges by randomising the occurrence records and calculating Schoener’s D 1000 times each, then compared the observed values with the null distribution of values. If the observed value fell within this range, we concluded the ranges were no more similar than would occur by chance alone, whereas if the value fell far from the mean of the null model, the ranges were similar. Thus, a significant P-value (P < 0.05) indicates there was significant similarity between the ranges of ABSTs and cane toads.
Species distribution models
Species distribution models were constructed using maximum entropy modelling (MaxEnt V3.4). MaxEnt uses maximum entropy (i.e. most spread out, or closest to uniform), subject to environmental features, to estimate the probability of presence of a species and generates an index of suitable habitat from 0 (lowest suitability) to 1 (highest suitability; Elith et al. 2011). For this, we used the same occurrence records we used for the niche modelling, as well as the filtered set of 10 bioclimatic variables that were obtained from WorldClim.
To set the threshold for discriminating suitable from unsuitable habitat, we applied the ‘minimum training presence’ threshold, as determined by the MaxEnt output. We used one of the lowest recommended thresholds, to avoid under-estimating potential range. To determine the importance of different variables to each species, we also calculated the relative contribution of each variable and the predicted index of habitat suitability for each occurrence record (Elith et al. 2011).
We generated a map to compare suitable habitat for ABSTs and cane toads by calculating the difference between cane toad and ABST habitat layers. We then squared the difference to provide an absolute value of similarity. Because this was calculated after the threshold had been applied, it only calculated the overlap in relation to the extent of the species with the smaller potential range (i.e. ABSTs).
Results
Life history
ABSTs and cane toads share similarities in morphology, life cycle, and habitat (Table 1a–b). Both ABSTs and cane toads mature in 1–2 years and produce clutches exceeding 30 000, depending on body size (Table 1b). Adults of both species prefer open environments and disturbed areas (Table 1c). They have similar diets as both tadpoles and adults, and as generalist predators, their diets include locally available prey (Table 1d). Adult ABSTs consume mostly ants and termites, whereas cane toads eat beetles, ants, and termites, and occasionally consume several species of vertebrate (Lever 2001; Beckmann and Shine 2012). Both ABSTs and cane toads can acclimate rapidly to new environments (Table 1e).
Habitus | Asian black-spined toad Duttaphrynus melanostictus | Cane toad Rhinella marina | |
---|---|---|---|
![]() | ![]() | ||
(a) Morphology | |||
Mean snout–vent length | Male: 72.9 mm Female: 85.3 mm | Male: 126.9 mm Female: 141.2 mm | |
Mean mass | Male: 46.7 g Female: 70.4 g1,2 | Male: 182.0 g Female: 262.9 g6,7,8 | |
Sexual dimorphism | Females are much larger than males. Highly variable colour pattern, usually grey to red–brown but can range from brick-red to almost black. The back is covered with warts of various sizes, which are topped with tiny dark spines.3,4 Possess prominent parotoid glands.5 | Females are larger than males. Males typically have yellow skin that feels like sandpaper to the touch, whereas females are smoother with dark marbled skin. Does not have the dark spines typical of ABST.9 Possess prominent parotoid glands.10 | |
(b) Life cycle | |||
Sexual reproduction | At 2 years females, males minimum 2 years.11 Breed once or twice a year.11 | At 1 or 2 years of age in most areas.17 Breed once a year.11,18 | |
Eggs | Prefers to lay eggs in shallow, still, or slow-flowing streams or pools of water.12 Large clutches (up to 40 000 eggs per clutch.5,13 in strings deposited in ponds or slow flowing streams.14 Eggs take 24–36 h to develop depending on temperature.3,15 | Prefers to lay eggs in shallow, still, or slow flowing water, limited vegetation.19,20 Large clutches (up to 30 000 eggs per clutch) in strings deposited in ponds or slow flowing streams.6,7,21,22 Eggs take 70–96 h to develop, dependent on temperature.7,17,18 | |
Tadpoles | Tadpoles metamorphose in 25–30 days (faster when in kin groups).15 | Tadpoles metamorphose in 14–28 days.21,23,24,25 | |
Length of life | 7–12 years in the wild depending on locality.3,16 | 10–15 years in the wild.6 | |
(c) Habitat | |||
Tadpole | Habitat not specifically documented but adapted to survive in shallow water – tail not well developed for swimming and has weak musculature.26 | Tadpoles school, usually within first 2 m of water’s edge.20 | |
Metamorph | Young toads are nocturnal and diurnal.27,28 Habitat selection not documented. | Diurnal, switching to nocturnal as they grow older.32,33 Metamorphs are restricted to margins of water bodies until they are old enough to disperse.22,34,35 | |
Adult | Ground-dwelling, preference for disturbed habitat.29,30 Typically detected in lowland habitats such as secondary forests, forest margins, riparian areas, and human-dominated agricultural and urban areas; uncommon in closed forest.31 | Ground-dwelling, preference for open and disturbed habitat.36,37,38 There is nowhere they are not found except if it is too cold. Prefer savannahs. Also common in coastal heath.39 | |
(d) Diet | |||
Tadpole | Primary food source algae, phytoplankton and detritus.27,29,40 Tadpoles can also feed on conspecific eggs and both conspecific and heterospecific adult and tadpole carrion (i.e. dead heterospecific tadpoles).41 | Primarily consume algae.21 Cannibalise eggs (but not tadpoles) of conspecifics. Rarely consume eggs of native anurans.21,46 Intraspecific predation restricted to pre-swimming embryo stages.21 | |
Metamorph | Not documented | Feed largely on invertebrates such as ants, beetles, hemipterans, and arachnids. May also consume smaller conspecifics.47 | |
Adult | Opportunistic feeder of ground-dwelling invertebrates, especially ants and termites.2,41,42,43,44 Adults are generalist predators whose diets most likely dependent on what prey is available.42 Only a single vertebrate has been recorded as ingested.45 | Opportunistic feeder of ground-dwelling invertebrates. Diverse diet with ants, termite and beetles forming primary food sources.6,7,48,49 Adults are generalist predators whose diets most likely dependent on what prey is available.6 A range of vertebrates have been recorded in the diet.7,50 | |
(e) Toxin concentration | Toxicity changes thought to be same as cane toad51 but are not documented. | Concentration highest in eggs. Decreases in the tadpole and is lowest in the late tadpole–early metamorph stages, then increases after metamorphosis.52 | |
(f) Spatial ecology | |||
Density | Can reach up to 1800 toads/hectare.53,54 | Can reach up to 2000–5000 toads/hectare.32,59 | |
Spatial use | Not documented | Occupy a large forage area (160 m2) but are not site specific.6 This area is smaller in the dry season, but in the wet season, toads are nomadic.8 | |
Daily movement | Not documented | Up to 1.8 km per night, but on average 264 m per night).60,61 | |
Rate of spread | Up to 3.3 km/year in Madagascar.55 | Up to 55–60 km/year at the Australian invasion front.25,62 | |
Thermal thresholds | Adults can tolerate exposure to 48°C for 8 days.56 Tolerance at other life stages not documented. Have the capacity for rapid thermal acclimation.57 | Critical thermal minimum of 10–12°C, and a critical thermal maximum of 41.5–42.5°C.6 Have the capacity for rapid thermal acclimation.6,63 | |
Water reliance | Tadpoles will metamorphose earlier in response to desiccation threat.58 Adult reliance on water and desiccation tolerance not documented. | Adults can last 5 days without water64 although toads in drier areas can develop cutaneous resistance to water loss.65,66 Adults can tolerate up to 50% body water loss,67 although behaviourally seek water after 10% body water loss.68 | |
(g) Call profile | Average call parameters: 26 s duration, 11.69 pulses/s, 1293 Hz, although these are highly variable among populations.30 | Average call parameters: 8 s duration, 15 pulses/s, 600 Hz.69,70 |
Data for ABST originally compiled in Christy (2020). Illustrations by CK.
References: (1) Alexander (1933); (2) Berry and Bullock (1962); (3) Bartlett et al. (2001); (4) Mo (2017); (5) Csurhes (2016); (6) Zug and Zug (1979); (7) Lever (2001); (8) Schwarzkopf and Alford (2002); (9) Narayan et al. (2008); (10) Cogger (2014); (11) Jørgensen et al. (1986); (12) Jayawardena et al. (2017); (13) Marshall et al. (2018); (14) Karraker and Dudgeon (2014); (15) Saidapur and Girish (2001); (16) Tyler (1989); (17) Alford et al. (1995); (18) Lampo and Medialdea (1996); (19) Semeniuk et al. (2007); (20) Lampo and de Leo (1998); (21) Hearnden (1991); (22) Shine et al. (2018); (23) Bayliss (1995); (24) Cabrera-Guzmán et al. (2011); (25) Rollins et al. (2015); (26) Asrafuzzaman et al. (2018); (27) Daniels (2005); (28) Sinha et al. (2001); (29) Wogan et al. (2016); (30) Ngo and Ngo (2013); (31) van Dijk et al. (2004); (32) Freeland and Kerin (1988); (33) Pizzatto et al. (2012); (34) Child et al. (2008a); (35) Brown et al. (2011); (36) Tingley et al. (2013); (37) Tingley et al. (2014); (38) Tingley et al. (2017); (39) Wijethunga et al. (2015); (40) Mahapatra et al. (2017); (41) Jamdar and Shinde (2013); (42) Norval et al. (2014); (43) Hui (2015); (44) Döring et al. (2017); (45) O’Shea et al. (2013); (46) Crossland (1998); (47) Pizzatto and Shine (2008); (48) Greenlees et al. (2006); (49) Kidera et al. (2008); (50) Beckmann and Shine (2012); (51) Marshall (2018); (52) Hayes et al. (2009); (53) McClelland et al. (2015); (54) Reardon et al. (2018); (55) Licata et al. (2019); (56) Deb et al. (1974); (57) Algiriyage et al. (2020); (58) Mogali et al. (2017); (59) Freeland (1986); (60) Phillips et al. (2006); (61) Phillips et al. (2007); (62) Urban et al. (2007); (63) McCann et al. (2014); (64) Gregg et al. (2019); (65) Brusch et al. (2019); (66) Kosmala et al. (2020); (67) Krakauer (1970); (68) Jørgensen (1991); (69) Bowcock et al. (2008); (70) Muller et al. (2016).
Despite their similarities, there are notable differences between the two species, including size. Average adult ABSTs are approximately 60% the snout–vent length and 25% the body mass of the average adult cane toad (Table 1a). The reported reproductive output of ABSTs is higher than that of cane toads (40 000 cf. 30 000 eggs per clutch), with reportedly more rapid egg development (36 h cf. 72 h, respectively), (Table 1b). The reported maximum density (1800 ha−1) and rate of spread (3.3 km/year) of ABSTs are both lower than for cane toads (5000 ha−1 and 60 km/year) (Table 1f). There are also differences in the call characteristics of the two species: ABST calls are longer and higher frequency than those of cane toads (Table 1g).
Niche overlap
The first two principal components selected by correlation analyses explained 64.46% of the variation in the bioclimatic variables (PC1 = 40.77%, PC2 = 23.69%) in the native range, and in the total range explained 63.18% of the variation in the bioclimatic variables (PC1 = 44.82%, PC2 = 18.36%) (Fig. 1a, c, respectively). There was a large climatic niche overlap of ABSTs and cane toads in native and total ranges (Fig. 2); in the native and total ranges, almost the entire cane toad distribution is captured within the ABST distribution, suggesting a high degree of similarity both in native and introduced environments. There were significant (P < 0.05) climatic niche similarities in the native (D = 0.180) and total ranges (D = 0.351) of ABSTs and cane toads (Fig. 1; individual variable overlap Fig. S1).
Comparison of climatic niches of ABSTs and cane toads in (a, b) native range only, and (c, d) total range – native and invaded. (a) and (c) represent the contribution of each climatic variable to the principal component axis for each species, and (b) and (d) represent the similarity test of the two species.
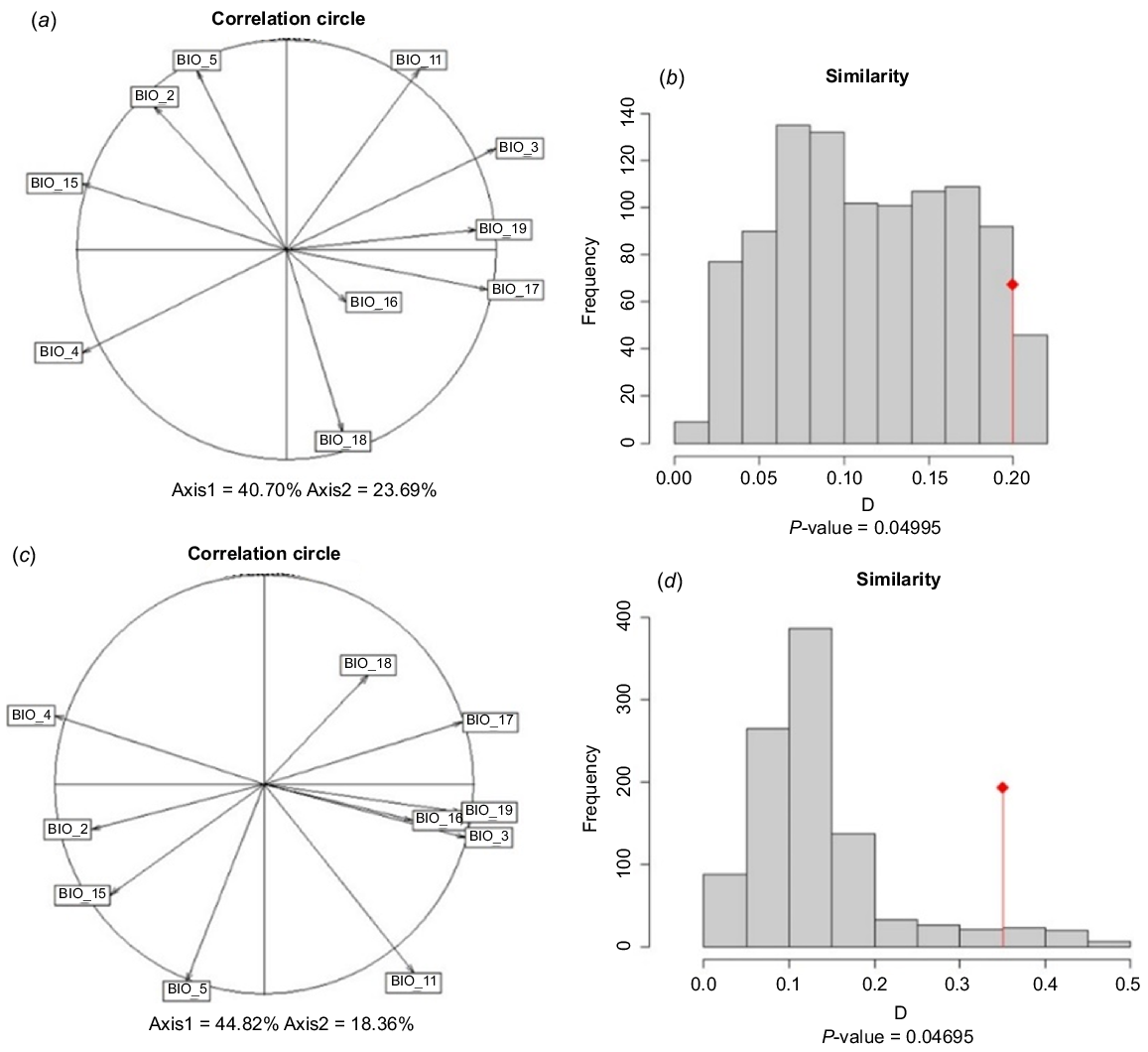
Species distribution models
The MaxEnt models had good predictive performance, producing AUC values of 0.881 for ABSTs and 0.794 for cane toads (Figs S2, S3). For ABSTs, precipitation in the wettest month (BIOL13) and the mean diurnal temperature range (BIOL2) were the two most significant factors affecting distribution, whereas low thermal variation (isothermality, BIOL3) and precipitation during the warmest quarter (BIOL18) were the two most significant factors for cane toads. ABST and cane toads tolerate similar precipitation in the wettest month. However, cane toads occupy habitat with a larger mean diurnal thermal range, higher isothermality, and less precipitation in the warmest quarter compared with ABST.
Potentially suitable habitat for ABSTs in Australia covers 1.94 million km2 (25.23% of the Australian landmass) compared with 7.68 million km2 (99.9% of the Australian landmass) for cane toads. Areas most vulnerable to invasion by ABSTs are the northern and eastern coastlines (Fig. 3). There is considerable overlap between ABSTs and cane toads in potentially highly suitable habitat, especially in the northern and eastern coastal regions of the Australian mainland (dark blue areas; Fig. 3c).
Maps of Australian MaxEnt-generated suitable habitat of (a) cane toad and (b) ABST. In these maps, the dark brown represents more suitable habitat and lighter colours represent low suitability. (c) Also presented is a map of habitat similarity between the two species. Dark purple represents habitat suitability that is highly similar (irrespective of quality) between cane toad and ABST moving to brown with increasing difference in suitability.
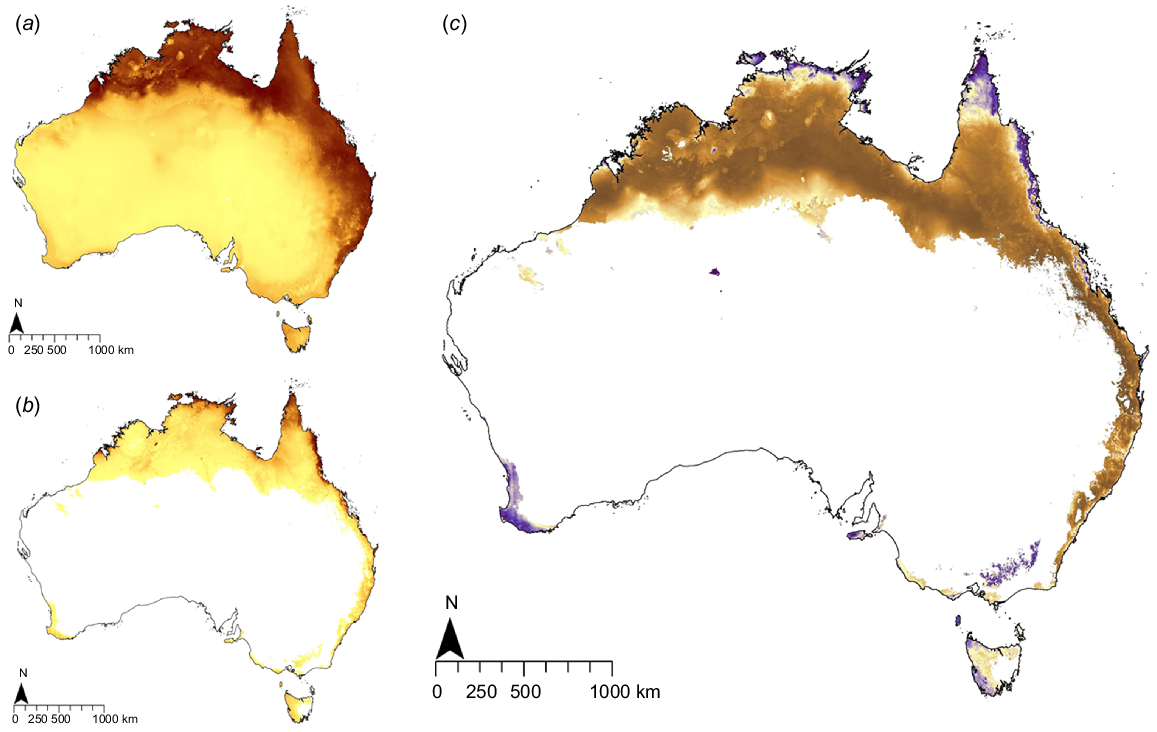
Discussion
Asian black-spined toads and cane toads share general similarities in morphology, large clutch sizes, preferred breeding sites, adult preference for disturbed land, and diet of both adults and tadpoles. In addition, they share similar climatic niches. However, there are key differences between the species: cane toads are larger, live longer, and have been recorded reaching higher densities and spreading more rapidly than ABSTs. Asian black-spined toads reportedly produce larger clutch sizes relative to body size and have faster egg development than cane toads. However, environmental conditions, body size, and invasion stage can be highly influential in anuran reproductive output (Hudson et al. 2015; Kelehear and Shine 2020), development (Kearney et al. 2008), density (Alford et al. 1995; Fan et al. 2013; Pikacha et al. 2015; Licata et al. 2019), rate of spread (Phillips et al. 2010), and call characteristics (Muller 2018), so reported figures are highly context dependent. Similarities between the species suggest ABSTs could pose similar threats to Australian ecosystems as cane toads (Bomford 2006). Similarities could also contribute to priority (i.e. order of invasion) effects in locations where ABSTs attempt to establish in the presence of cane toads.
Potential interactions between ABSTs and native species
Establishment of ABSTs in Australia would create novel interactions in Australian ecosystems. The impacts of ABSTs on ecosystems in their invaded range are poorly documented, although they competitively displace native Indonesian toads (Ingerophrynus biporcatus) (Church 1960; Iskander 2004). Given the similarities in life histories, ABSTs, like cane toads, may have both top-down and bottom-up trophic effects (Shine 2010) and compete with native species. For example, adult cane toads in Australia consume vast quantities of invertebrates, and in doing so can out-compete native anurans (Greenlees et al. 2006). Tadpoles of cane toads and native Australian frog species can also compete, reducing the number of native metamorphs (Crossland et al. 2009). Further, cane toads compete with native frogs for acoustic space (Hu et al. 2009; Brodie et al. 2021), leaving fewer gaps of silence for native frogs to exploit (Bleach et al. 2015). The dominant call frequency of ABSTs overlaps those of several native frogs in Australia (at least 28 species compared with approximately 12 species overlapping with cane toads (Allen-Ankins, pers. comm.; Allen-Ankins and Schwarzkopf 2022)), and their establishment in Australia could potentially reduce calling behaviour of native frogs (Taylor et al. 2017).
In terms of bottom-up effects, cane toads pose risks to some native species that prey on them, primarily because Australian predators have little resistance to bufotoxins (Phillips et al. 2003; Boland 2004; Shine 2010). Mortality from consumption of toads can significantly reduce predator populations at the invasion front, where species are naïve to cane toads (Letnic et al. 2008; Doody et al. 2009, 2015; Jolly et al. 2016). The similarities in life history combined with the difference in size between ABSTs and cane toads mean it is likely that ABSTs would have similar effects on native species to those of cane toads. The difference in adult size between the two species warrants investigation of the potential impacts on native predators of having a second, smaller toxic toad in Australia.
Potential interactions between ABST and cane toads
Given the species are related, share similarities in life history and climatic niches with potential habitat overlap, ABST establishment in Australia would mean they would likely interact with cane toads. When a species invades an ecosystem, it can be met with biotic resistance from resident species (Von Holle and Simberloff 2005). In some cases where an invasive species has already established, priority effects can impact the invasion success of later arriving species (Shulman et al. 1983; Wainwright et al. 2012). Priority effects can be more pronounced for closely related species (Tan et al. 2012). Cane toads are established across much of the potential ABST habitat in northern Australia. Although not universal, greater body size can provide a competitive advantage over similar species (Martin and Ghalambor 2014), and has been identified as an important factor in successful amphibian introductions (Tingley et al. 2010). Both priority effects and larger body size may convey a competitive advantage to cane toads. Moreover, Vidal-García and Keogh (2017) have proposed that the success of cane toads in Australia is due to their distinct morphology, coupled with a broad trophic niche breadth, allowing them to exploit an empty niche among Australian anurans. The morphological and dietary similarities between ABSTs and cane toads indicate scope for interspecific competition.
Interspecific competition between cane toads and ABST could result in constriction, divergence, or, conversely, expansion of the ABST or cane toad ecological niche (Dominguez Almela et al. 2021). An extreme, but unlikely, outcome could be competitive exclusion (Bøhn et al. 2008; Simha et al. 2022). Change of the ecological niche of ABSTs caused by interspecific competition could have flow-on effects for Australian ecosystems. Examination of a location where the two species co-occur as invasive species would be instructive. However, although the global introduced ranges of ABSTs and cane toads overlap in New Guinea (van Winkel and Lane 2012; Licata et al. 2019), they do not occur in sympatry there (Richards, pers. comm.; Menzies, pers. comm.). As such, it is not possible to determine the extent of interspecific competition between the species or any combined impacts of both species on native ecosystems using in situ observations.
An approach that may help estimate comparative impacts of the two species is to compare their functional and numerical responses. Comparative functional response (CFR) aims to quantify the resource consumption efficiency of functionally analogous species (Dick et al. 2014). Functional responses are assessed as a combination of the species attack rate and prey-handling time (Dick et al. 2013). CFR has been conducted between potentially invasive species and analogous native species to predict the impact of the invasive species (Dick et al. 2014; Laverty et al. 2017; Dickey et al. 2020). By combining functional responses with proxies for numerical responses, relative impact potential (RIP) further quantifies potential ecological impacts of invasive species and can be used to examine the potential role of competition in invasions (Dickey et al. 2020). Using an RIP approach to compare possible impacts of ABST and cane toads may shed light on potential competition; however, this analysis focuses entirely on competition for food. Cane toads and ABSTs may compete for other resources (e.g. breeding sites, acoustic space) as well.
A key difference in the invasion history of cane toads and ABST in Australia is propagule pressure. Thousands of captive-bred cane toad tadpoles were deliberately liberated in 1935 (Froggatt 1936; Lever 2001), and approximately 112 ABST (102 alive) have been intercepted in Australia since 1999 (Tingley et al. 2018). Although the difference in propagule pressure is marked, introductions of relatively small numbers of ABST may still pose a significant establishment threat due to the high reproductive capacity of ABST. In Madagascar, the ABST population was likely established from accidental introduction of a limited number of individuals released into highly suitable habitat surrounding ports (McClelland et al. 2015; Pearson 2015). This population spread over 10 800 ha and reached over 4 000 000 post-metamorphic toads in the 4–8 years following incursion (Reardon et al. 2018). This emphasises how rapidly a species can proliferate when the novel environment is highly suitable, even with relatively small founding populations.
Species distribution modelling
Our species distribution modelling shows marked differences in the area of suitable habitat for the two species in Australia. Our cane toad species distribution model for Australia is broadly similar to recent modelling (Kearney et al. 2008; Andersen et al. 2021; Sales et al. 2021) and indicates that much of northern and eastern Australia includes highly suitable habitat for the species. In comparison, our species distribution model for ABST suggests that suitable habitat is largely constrained to a narrow band of eastern northern Australia. This overlaps with the locations where border interceptions of ABST at ports have been most frequent (Tingley et al. 2018). Our modelling is consistent with models by Andersen et al. (2021) and Page et al. (2008), although these authors suggest a broader range of suitable habitat across northern Australia, with highest habitat suitability away from the coast. Our ABST species distribution modelling differs from that of Tingley et al. (2018), who suggest a more south-easterly potential distribution of ABST. Differences are likely due to different variable selection used in the analyses.
There is a notable difference between the climatic niche overlap, where cane toad climatic niche falls within that of ABSTs, and the spatial distribution modelling, where the potential Australian distribution of ABSTs falls within that of cane toads. There is significant overlap between the two species in terms of precipitation of the wettest month, although ABST occupy habitat that has comparatively more precipitation during the summer and areas with less precipitation in the dry season. It is possible that ABST habitat in Australia is more limited because cane toads can occupy habitat with a wider diurnal thermal range, higher isothermality, and lower precipitation in the warmest quarter of the year than ABST (Fig. S1). These differences may also occur because we lack ABST records within Australia (unlike cane toads), influencing the accuracy of distribution mapping and cropping the global habitat suitability to Australia.
According to our species distribution modelling, the area of potential habitat for ABST in Australia is almost entirely contained within the area of suitable habitat for cane toads. The overlap of highly suitable habitat occurs predominantly in coastal north-east Queensland, the north-east of the Northern Territory, and the western Kimberley. It is important to note, however, that cane toads have exhibited shifts in their realised climatic niche during their invasion of Australia, expanding their range well beyond that predicted from the native range (Tingley et al. 2014; Sales et al. 2021). When climatic niche shifts were examined for ABSTs alone, there was a significant similarity between their native and introduced ranges. A lack of an observed shift in their climatic niche may be due to their presently limited international distribution and, therefore, little opportunity to spread beyond their current climatic envelope. As such, ABST could potentially adapt to new climatic niches.
Knowledge gaps
ABSTs are not well studied across their range. In comparison, cane toads have been studied extensively, but with a particular focus on their invaded range in Australia (Shine 2010). The published literature we reviewed may not capture the full extent of variation in the life history of either species. We note that ABSTs comprise a species complex (Wogan et al. 2016), and that cane toads, as considered in this study, include R. marina and R. horribilis. Thus, variation in life history characteristics between species is expected, and also among populations of the same species in different locations. Where similarities occur there may be nuanced, consequential differences that have not been documented (Crossland et al. 2009).
In addition to a lack of information about potential competitive interactions between ABSTs and cane toads, there are several undocumented areas of ABST ecology, which would be useful to study to enhance response planning for incursions. These include details of spatial ecology, thermal and desiccation thresholds, and diet and habitat of tadpoles and metamorphs. Additionally, there are recorded differences in traits between ABSTs and cane toads identified in the literature, but these traits are strongly influenced by environmental conditions that could be further explored. This applies to clutch size, egg development rate, maximum densities, rates of spread, and call characteristics.
A detailed understanding of species ecology can inform response management. Knowledge of the extent of dependence of ABSTs on water sources could help predict ABST spread and suggest control measures. For example, cane toad activity during the dry season is restricted to permanent water bodies (Schwarzkopf and Alford 2002; Southwell et al. 2017). In arid Western Australia, cane toads can survive a maximum of 5 days without water and move up to 5.35 km during that period (Gregg et al. 2019). However, cane toads can alter their behaviour to reduce the effects of desiccation in water-limited environments, for example by finding underground water, burrowing, and remaining inactive (Schwarzkopf and Alford 2002; Brusch et al. 2019). In addition, cane toad amplexus can occur both close to, and distant from water bodies (Bowcock et al. 2009). It is unknown how ABSTs respond to arid or water-limited environments, which could affect their distribution in Australia. Understanding these traits could allow for targeted surveillance and response measures.
Thermal thresholds can be used to infer the success of a species in different environments. Cane toad tadpoles cannot develop in water below 16°C, and metamorphs have a lethal maximum temperature of 42°C, which may limit their distribution (Child et al. 2008b; Wijethunga et al. 2016). However, cane toads have increased cold tolerance following establishment and spread in both Australia and Florida (McCann et al. 2014; Mittan and Zamudio 2019). ABSTs occur at a range of elevations and can alter thermal sensitivity based on temperature (i.e. toads from lower elevations have higher thermal optimal temperatures, whereas toads from higher elevations have lower thermal optima (Algiriyage et al. 2020)), yet little information exists on the effect of temperature on ABST performance or their development. As such, the influence of exposure to extreme temperatures, such as those found in Australia, on ABST development, behaviour, or potential distribution are not known. The scope for ABSTs to increase cold tolerance in new environments, as cane toads have done, is also unknown. More detailed information on thermal tolerances would allow detailed modelling of survival of incipient populations and potential habitat.
Conclusion
ABSTs pose a significant biosecurity threat to Australia. Much of the northern coastline, including where border interceptions at ports have been most frequent (Tingley et al. 2018), is suitable habitat, and ABSTs potentially have impacts similar to those of cane toads. Should an ABST incursion occur, the invading population will likely encounter an established cane toad population. Because the two species share life history similarities and exhibit similar climatic niches, interspecific competition may affect establishment and impacts of ABSTs. Comparative investigation of the life histories and functional responses of these two species, undertaken within the native and invaded ranges of ABSTs, would help indicate the likely outcomes of species interactions in the context of an ABST invasion of Australia. Examination of key components of ABST life history, including thermal and desiccation thresholds and spatial and reproductive ecology, will assist in response planning for ABST incursions.
Acknowledgements
This manuscript benefited from discussions with Slade Allen-Ankins, Sheryn Brodie, Stewart McDonald, and Tony Pople. Matt Gentle and Tony Pople provided valuable comments on the manuscript. Unpublished records of ABST in Madagascar were provided by Fulvio Licata and Angelica Crottini.
References
Acevedo AA, Lampo M, Cipriani R (2016) The cane or marine toad, Rhinella marina (Anura, Bufonidae): two genetically and morphologically distinct species. Zootaxa 4103, 574-586.
| Crossref | Google Scholar |
Alexander G (1933) Secondary sexual characters of Bufo melanostictus Schneider. Copeia 1933, 204-207.
| Crossref | Google Scholar |
Alford RA, Cohen MP, Crossland MR, Hearnden MN, Schwarzkopf L (1995) Population biology of Bufo marinus in northern Australia. In ‘Wetland Research in the Wet–Dry Tropics of Australia.’ Supervising Scientist Report. (Ed. M Finlayson) pp. 173–181. (Office of the Supervising Scientist: Canberra, ACT, Australia)
Algiriyage DPH, Jayaweera H, Wijesinghe MR (2020) Inter-population variation in thermal sensitivity of the tropical toad Duttaphrynus melanostictus, across a small spatial scale in Sri Lanka. Journal of Thermal Biology 89, 102568.
| Crossref | Google Scholar |
Allen-Ankins S, Schwarzkopf L (2022) Using citizen science to test for acoustic niche partitioning in frogs. Scientific Reports 12, 1-7.
| Crossref | Google Scholar |
Andersen D, Borzée A, Jang Y (2021) Predicting global climatic suitability for the four most invasive anuran species using ecological niche factor analysis. Global Ecology and Conservation 25, e01433.
| Crossref | Google Scholar |
Asrafuzzaman S, Mahapatra S, Rout J, Sahoo G (2018) Dietary assessment of five species of anuran tadpoles from northern Odisha, India. Journal of Threatened Taxa 10, 12382-12388.
| Crossref | Google Scholar |
Beckmann C, Shine R (2012) How many of Australia’s ground-nesting birds are likely to be at risk from the invasive cane toad (Rhinella marina)? Emu – Austral Ornithology 112, 83-89.
| Crossref | Google Scholar |
Berry P, Bullock J (1962) The food of the common malayan toad, Bufo melanostictus Schneider. Copeia 1962, 736-741.
| Google Scholar |
Bleach IT, Beckmann C, Both C, Brown GP, Shine R (2015) Noisy neighbours at the frog pond: effects of invasive cane toads on the calling behaviour of native Australian frogs. Behavioral Ecology and Sociobiology 69, 675-683.
| Crossref | Google Scholar |
Boland CRJ (2004) Introduced cane toads Bufo marinus are active nest predators and competitors of rainbow bee-eaters Merops ornatus: observational and experimental evidence. Biological Conservation 120, 53-62.
| Crossref | Google Scholar |
Bowcock H, Brown GP, Shine R (2008) Sexual communication in cane toads, Chaunus marinus: what cues influence the duration of amplexus? Animal Behaviour 75, 1571-1579.
| Crossref | Google Scholar |
Bowcock H, Brown GP, Shine R (2009) Beastly bondage: the costs of amplexus in cane toads (Bufo marinus). Copeia 2009, 29-36.
| Crossref | Google Scholar |
Brodie S, Yasumiba K, Towsey M, Roe P, Schwarzkopf L (2021) Acoustic monitoring reveals year-round calling by invasive toads in tropical Australia. Bioacoustics 30, 125-141.
| Crossref | Google Scholar |
Broennimann O, Fitzpatrick MC, Pearman PB, Petitpierre B, Pellissier L, Yoccoz NG, Thuiller W, Fortin M-J, Randin C, Zimmermann NE, Graham CH, Guisan A (2012) Measuring ecological niche overlap from occurrence and spatial environmental data. Global Ecology and Biogeography 21, 481-497.
| Crossref | Google Scholar |
Broennimann O, Di Cola V, Guisan A (2021) Ecospat: spatial ecology miscellaneous methods. (R package version 3.2). Available at https://CRAN.R-project.org/package=ecospat.
Brown GP, Phillips BL, Shine R (2011) The ecological impact of invasive cane toads on tropical snakes: field data do not support laboratory-based predictions. Ecology 92, 422-431.
| Crossref | Google Scholar |
Brusch GA, Christian K, Brown GP, Shine R, DeNardo DF (2019) Cane toads (Rhinella marina) rely on water access, not drought tolerance, to invade xeric Australian environments. Oecologia 189, 307-316.
| Crossref | Google Scholar |
Bøhn T, Amundsen P-A, Sparrow A (2008) Competitive exclusion after invasion? Biological Invasions 10, 359-368.
| Crossref | Google Scholar |
Byers JE, Reichard S, Randall JM, Parker IM, Smith CS, Lonsdale WM, Atkinson IAE, Seastedt TR, Williamson M, Chornesky E (2002) Directing research to reduce the impacts of nonindigenous species. Conservation Biology 16, 630-640.
| Crossref | Google Scholar |
Cabrera-Guzmán E, Crossland M, Shine R (2011) Can we use the tadpoles of Australian frogs to reduce recruitment of invasive cane toads? Journal of Applied Ecology 48, 462-470.
| Crossref | Google Scholar |
Catford JA, Jansson R, Nilsson C (2009) Reducing redundancy in invasion ecology by integrating hypotheses into a single theoretical framework. Diversity and Distributions 15, 22-40.
| Crossref | Google Scholar |
Child T, Phillips BL, Shine R (2008a) Abiotic and biotic influences on the dispersal behavior of metamorph cane toads (Bufo marinus) in tropical Australia. Journal of Experimental Zoology Part A: Ecological Genetics and Physiology 309, 215-224.
| Crossref | Google Scholar |
Child T, Phillips BL, Brown GP, Shine R (2008b) The spatial ecology of cane toads (Bufo marinus) in tropical Australia: why do metamorph toads stay near the water? Austral Ecology 33, 630-640.
| Crossref | Google Scholar |
Christy MT, Savidge JA, Rodda GH (2007) Multiple pathways for invasion of anurans on a Pacific island. Diversity and Distributions 13, 598-607.
| Crossref | Google Scholar |
Church G (1960) The invasion of Bali by Bufo melanostictus. Herpetologica 16, 15-21.
| Google Scholar |
Crossland MR (1998) A comparison of cane toad and native tadpoles as predators of native anuran eggs, hatchlings and larvae. Wildlife Research 25, 373-381.
| Crossref | Google Scholar |
Crossland MR, Alford RA, Shine R (2009) Impact of the invasive cane toad (Bufo marinus) on an Australian frog (Opisthodon ornatus) depends on minor variation in reproductive timing. Oecologia 158, 625-632.
| Crossref | Google Scholar |
Deb C, Chatterjee S, Boral MC (1974) Body fluid and hematological changes in toads following heat exposure. American Journal of Physiology-Legacy Content 226, 408-410.
| Crossref | Google Scholar |
Di Cola V, Broennimann O, Petitpierre B, Breiner FT, d’Amen M, Randin C, Engler R, Pottier J, Pio D, Dubuis A, Pellissier L, Mateo RG, Hordijk W, Salamin N, Guisan A (2017) ecospat: an R package to support spatial analyses and modeling of species niches and distributions. Ecography 40, 774-787.
| Crossref | Google Scholar |
Dick JTA, Gallagher K, Avlijas S, Clarke HC, Lewis SE, Leung S, Minchin D, Caffrey J, Alexander ME, Maguire C, Harrod C, Reid N, Haddaway NR, Farnsworth KD, Penk M, Ricciardi A (2013) Ecological impacts of an invasive predator explained and predicted by comparative functional responses. Biological Invasions 15, 837-846.
| Crossref | Google Scholar |
Dick JTA, Alexander ME, Jeschke JM, Ricciardi A, MacIsaac HJ, Robinson TB, Kumschick S, Weyl OLF, Dunn AM, Hatcher MJ, Paterson RA, Farnsworth KD, Richardson DM (2014) Advancing impact prediction and hypothesis testing in invasion ecology using a comparative functional response approach. Biological Invasions 16, 735-753.
| Crossref | Google Scholar |
Dickey JWE, Cuthbert RN, South J, Britton JR, Caffrey J, Chang X, Crane K, Coughlan NE, Fadaei E, Farnsworth KD, Ismar-Rebitz SMH, Joyce PWS, Julius M, Laverty C, Lucy FE, MacIsaac HJ, McCard M, McGlade CLO, Reid N, Ricciardi A, Wasserman RJ, Weyl OLF, Dick JTA (2020) On the rip: using relative impact potential to assess the ecological impacts of invasive alien species. NeoBiota 55, 27-60.
| Crossref | Google Scholar |
Dominguez Almela V, South J, Britton JR (2021) Predicting the competitive interactions and trophic niche consequences of a globally invasive fish with threatened native species. Journal of Animal Ecology 90, 2651-2662.
| Crossref | Google Scholar |
Doody JS, Green B, Rhind D, Castellano CM, Sims R, Robinson T (2009) Population-level declines in Australian predators caused by an invasive species. Animal Conservation 12, 46-53.
| Crossref | Google Scholar |
Doody JS, Soanes R, Castellano CM, Rhind D, Green B, McHenry CR, Clulow S (2015) Invasive toads shift predator–prey densities in animal communities by removing top predators. Ecology 96, 2544-2554.
| Crossref | Google Scholar |
Döring B, Mecke S, Kieckbusch M, O’Shea M, Kaiser H (2017) Food spectrum analysis of the Asian toad, Duttaphrynus melanostictus (Schneider, 1799) (Anura: Bufonidae), from Timor Island, Wallacea. Journal of Natural History 51, 607-623.
| Crossref | Google Scholar |
Easteal S (1981) The history of introductions of Bufo marinus (Amphibia: Anura); a natural experiment in evolution. Biological Journal of the Linnean Society 16, 93-113.
| Crossref | Google Scholar |
Elith J, Phillips SJ, Hastie T, Dudik M, Chee YE, Yates CJ (2011) A statistical explanation of MaxEnt for ecologists. Diversity and Distributions 17, 43-57.
| Crossref | Google Scholar |
Fan X-L, Lin Z-H, Ji X (2013) Male size does not correlate with fertilization success in two bufonid toads that show size-assortative mating. Current Zoology 59, 740-746.
| Crossref | Google Scholar |
Forsyth DM, Duncan RP, Bomford M, Moore G (2004) Climatic suitability, life-history traits, introduction effort, and the establishment and spread of introduced mammals in Australia. Conservation Biology 18, 557-569.
| Crossref | Google Scholar |
Freeland WJ (1986) Populations of cane toad, Bufo marinus, in relation to time since colonization. Wildlife Research 13, 321-329.
| Crossref | Google Scholar |
Freeland WJ, Kerin SH (1988) Within-habitat relationships between invading Bufo marinus and Australian species of frog during the tropical dry season. Wildlife Research 15, 293-305.
| Crossref | Google Scholar |
Froggatt WW (1936) The introduction of the great mexican toad Bufo marinus into Australia. Australian Naturalist 9, 163-164.
| Google Scholar |
García-Díaz P, Cassey P (2014) Patterns of transport and introduction of exotic amphibians in Australia. Diversity and Distributions 20, 455-466.
| Google Scholar |
Greenlees MJ, Brown GP, Webb JK, Phillips BL, Shine R (2006) Effects of an invasive anuran [the cane toad (Bufo marinus)] on the invertebrate fauna of a tropical Australian floodplain. Animal Conservation 9, 431-438.
| Crossref | Google Scholar |
Gregg EA, Tingley R, Phillips BL (2019) The on-ground feasibility of a waterless barrier to stop the spread of invasive cane toads in western Australia. Conservation Science and Practice 1, e74.
| Crossref | Google Scholar |
Hayes RA, Crossland MR, Hagman M, Capon RJ, Shine R (2009) Ontogenetic variation in the chemical defenses of cane toads (Bufo marinus): toxin profiles and effects on predators. Journal of Chemical Ecology 35, 391-399.
| Crossref | Google Scholar |
Hoffmann BD, Broadhurst LM (2016) The economic cost of managing invasive species in Australia. NeoBiota 31, 1-18.
| Crossref | Google Scholar |
Hu W, Bulusu N, Chou CT, Jha S, Taylor A, Tran VN (2009) Design and evaluation of a hybrid sensor network for cane toad monitoring. ACM Transactions on Sensor Networks (TOSN) 5, 1-28.
| Crossref | Google Scholar |
Hudson CM, Phillips BL, Brown GP, Shine R (2015) Virgins in the vanguard: low reproductive frequency in invasion-front cane toads. Biological Journal of the Linnean Society 116, 743-747.
| Crossref | Google Scholar |
Hulme PE (2015) Invasion pathways at a crossroad: policy and research challenges for managing alien species introductions. Journal of Applied Ecology 1418-1424.
| Crossref | Google Scholar |
Iskander D (2004) Ingerophrynus biporcatus. IUCN red list of threatened species. Available at https://www.iucnredlist.org/species/54588/11156606
Jamdar S, Shinde K (2013) Gut content analysis of common Indian toad Duttaphrynus melanostictus (Schneider, 1799) Frost et al., 2006 (Anura: Bufonidae) from Aurangabad (Maharashtra) India. Indian Journal of Scientific Research and Technology 1, 23-26.
| Google Scholar |
Jayawardena UA, Rohr JR, Amerasinghe PH, Navaratne AN, Rajakaruna RS (2017) Effects of agrochemicals on disease severity of Acanthostomum burminis infections (Digenea: Trematoda) in the Asian common toad, Duttaphrynus melanostictus. BMC Zoology 2, 13.
| Crossref | Google Scholar |
Jolly CJ, Shine R, Greenlees MJ (2016) The impacts of a toxic invasive prey species (the cane toad, Rhinella marina) on a vulnerable predator (the lace monitor, Varanus varius). Biological Invasions 18, 1499-1509.
| Crossref | Google Scholar |
Jørgensen CB, Shakuntala K, Vijayakumar S (1986) Body size, reproduction and growth in a tropical toad, Bufo melanostictus, with a comparison of ovarian cycles in tropical and temperate zone anurans. Oikos 46, 379-389.
| Crossref | Google Scholar |
Karraker NE, Dudgeon D (2014) Invasive apple snails (Pomacea canaliculata) are predators of amphibians in south China. Biological Invasions 16, 1785-1789.
| Crossref | Google Scholar |
Kearney M, Phillips BL, Tracy CR, Christian KA, Betts G, Porter WP (2008) Modelling species distributions without using species distributions: the cane toad in Australia under current and future climates. Ecography 31, 423-434.
| Crossref | Google Scholar |
Kearney SG, Carwardine J, Reside AE, Fisher DO, Maron M, Doherty TS, Legge S, Silcock J, Woinarski JCZ, Garnett ST, Wintle BA, Watson JEM (2019) The threats to Australia’s imperilled species and implications for a national conservation response. Pacific Conservation Biology 25, 328.
| Crossref | Google Scholar |
Kelehear C, Shine R (2020) Tradeoffs between dispersal and reproduction at an invasion front of cane toads in tropical Australia. Scientific Reports 10, 486.
| Crossref | Google Scholar |
Kidera N, Tandavanitj N, Oh D, Nakanishi N, Satoh A, Denda T, Izawa M, Ota H (2008) Dietary habits of the introduced cane toad Bufo marinus (Amphibia: Bufonidae) on Ishigakijima, southern Ryukyus, Japan. Pacific Science 62, 423-430.
| Crossref | Google Scholar |
Kosmala GK, Brown GP, Shine R, Christian K (2020) Skin resistance to water gain and loss has changed in cane toads (Rhinella marina) during their Australian invasion. Ecology and Evolution 10, 13071-13079.
| Crossref | Google Scholar |
Krakauer T (1970) Tolerance limits of the toad, Bufo marinus, in south Florida. Comparative Biochemistry and Physiology 33, 15-26.
| Crossref | Google Scholar |
Lampo M, de Leo GA (1998) The invasion ecology of the toad Bufo marinus: from South America to Australia. Ecological Applications 8, 388-396.
| Crossref | Google Scholar |
Lampo M, Medialdea V (1996) Energy allocation patterns in Bufo marinus from two habitats in Venezuela. Journal of Tropical Ecology 12, 321-331.
| Crossref | Google Scholar |
Laverty C, Green KD, Dick JTA, Barrios-O’Neill D, Mensink PJ, Médoc V, Spataro T, Caffrey JM, Lucy FE, Boets P, Britton JR, Pegg J, Gallagher C (2017) Assessing the ecological impacts of invasive species based on their functional responses and abundances. Biological Invasions 19, 1653-1665.
| Crossref | Google Scholar |
Letnic M, Webb JK, Shine R (2008) Invasive cane toads (Bufo marinus) cause mass mortality of freshwater crocodiles (Crocodylus johnstoni) in tropical Australia. Biological Conservation 141, 1773-1782.
| Crossref | Google Scholar |
Licata F, Ficetola GF, Freeman K, Mahasoa RH, Ravololonarivo V, Solofo Niaina Fidy JF, Koto-Jean AB, Nahavitatsara ER, Andreone F, Crottini A (2019) Abundance, distribution and spread of the invasive Asian toad Duttaphrynus melanostictus in eastern Madagascar. Biological Invasions 21, 1615-1626.
| Crossref | Google Scholar |
Licata F, Andreone F, Crottini A, Harison RF, Ficetola GF (2021) Does spatial sorting occur in the invasive Asian toad in Madagascar? Insights into the invasion unveiled by morphological analyses. Journal of Zoological Systematics and Evolutionary Research 59, 2161-2169.
| Crossref | Google Scholar |
Lockwood JL, Welbourne DJ, Romagosa CM, Cassey P, Mandrak NE, Strecker A, Leung B, Stringham OC, Udell B, Episcopio-Sturgeon DJ, Tlusty MF, Sinclair J, Springborn MR, Pienaar EF, Rhyne AL, Keller R (2019) When pets become pests: the role of the exotic pet trade in producing invasive vertebrate animals. Frontiers in Ecology and the Environment 17, 323-330.
| Crossref | Google Scholar |
Mahapatra S, Dutta SK, Sahoo G (2017) Opportunistic predatory behaviour in Duttaphrynus melanostictus (Schneider, 1799) tadpoles. Current Science 112, 1755-1759.
| Crossref | Google Scholar |
Marshall BM, Casewell NR, Vences M, Glaw F, Andreone F, Rakotoarison A, Zancolli G, Woog F, Wüster W (2018) Widespread vulnerability of Malagasy predators to the toxins of an introduced toad. Current Biology 28, R654-R655.
| Crossref | Google Scholar |
Martin PR, Ghalambor CK (2014) When David beats Goliath: the advantage of large size in interspecific aggressive contests declines over evolutionary time. PLoS ONE 9, e108741.
| Crossref | Google Scholar |
McCann S, Greenlees MJ, Newell D, Shine R (2014) Rapid acclimation to cold allows the cane toad to invade montane areas within its Australian range. Functional Ecology 28, 1166-1174.
| Crossref | Google Scholar |
Mittan CS, Zamudio KR (2019) Rapid adaptation to cold in the invasive cane toad Rhinella marina. Conservation Physiology 7, coy075.
| Google Scholar |
Mo M (2017) Asian black-spined toads (Duttaphrynus melanostictus) in Australia: an invasion worth avoiding. Reptiles & Amphibians 24, 155-161.
| Crossref | Google Scholar |
Mogali S, Saidapur S, Shanbhag B (2017) Influence of desiccation threat on the metamorphic traits of the Asian common toad, Duttaphrynus melanostictus (Anura). Acta Herpetologica 12, 175-180.
| Crossref | Google Scholar |
Muller BJ, Pike DA, Schwarzkopf L (2016) Defining the active space of cane toad (Rhinella marina) advertisement calls: males respond from further than females. Behaviour 153, 1951-1969.
| Crossref | Google Scholar |
Mungomery R, Buzacott J (1936) A preliminary study in trapping “greyback” cane beetles. In ‘Proceedings of the International Society of Sugar Cane Technologists. Fifth Congress, Brisbane, 27 August–3 September 1935’. pp. 464–469. (Australian Society of Sugar Cane Technologists: Mackay, Qld, Australia)
Narayan E, Christi K, Morley C, Trevenen P (2008) Sexual dimorphism in the cane toad Bufo marinus: a quantitative comparison of visual inspection methods for sexing individuals. The Herpetological Journal 18, 63-65.
| Google Scholar |
Negussie A, Achten WMJ, Norgrove L, Hermy M, Muys B (2013) Invasiveness risk of biofuel crops using Jatropha curcas L. as a model species. Biofuels, Bioproducts and Biorefining 7, 485-498.
| Crossref | Google Scholar |
Ngo BV, Ngo CD (2013) Reproductive activity and advertisement calls of the Asian common toad Duttaphrynus melanostictus (Amphibia, Anura, Bufonidae) from Bach Ma National Park, Vietnam. Zoological Studies 52, 12.
| Crossref | Google Scholar |
Norval G, Huang S-C, Mao J-J, Goldberg SR, Yang Y-J (2014) Notes on the diets of five amphibian species from southwestern Taiwan. Alytes 30, 69.
| Google Scholar |
Othman SN, Chen Y-H, Chuang M-F, Andersen D, Jang Y, Borzée A (2020) Impact of the mid-pleistocene revolution and anthropogenic factors on the dispersion of Asian black-spined toads (Duttaphrynus melanostictus). Animals 10, 1157.
| Crossref | Google Scholar |
O’Shea M, Kathriner A, Mecke S, Sanchez C, Kaiser H (2013) ‘Fantastic voyage’: a live blindsnake (Ramphotyphlops braminus) journeys through the gastrointestinal system of a toad (Duttaphrynus melanostictus). Herpetology Notes 6, 467-470.
| Google Scholar |
Pearson RG (2015) Asian common toads in Madagascar: an urgent effort to inform surveys and eradication efforts. Global Change Biology 21, 9.
| Crossref | Google Scholar |
Phillips BL, Brown GP, Shine R (2003) Assessing the potential impact of cane toads on Australian snakes. Conservation Biology 17, 1738-1747.
| Crossref | Google Scholar |
Phillips BL, Brown GP, Webb JK, Shine R (2006) Invasion and the evolution of speed in toads. Nature 439, 803.
| Crossref | Google Scholar |
Phillips BL, Brown GP, Greenlees M, Webb JK, Shine R (2007) Rapid expansion of the cane toad (Bufo marinus) invasion front in tropical Australia. Austral Ecology 32, 169-176.
| Crossref | Google Scholar |
Phillips BL, Brown GP, Shine R (2010) Evolutionarily accelerated invasions: the rate of dispersal evolves upwards during the range advance of cane toads. Journal of Evolutionary Biology 23, 2595-2601.
| Crossref | Google Scholar |
Pikacha P, Lavery T, Leung LK-P (2015) What factors affect the density of cane toads (Rhinella marina) in the Solomon Islands? Pacific Conservation Biology 21, 200-207.
| Crossref | Google Scholar |
Pizzatto L, Shine R (2008) The behavioral ecology of cannibalism in cane toads (Bufo marinus). Behavioral Ecology and Sociobiology 63, 123-133.
| Crossref | Google Scholar |
Pizzatto L, Kelehear C, Dubey S, Barton D, Shine R (2012) Host–parasite relationships during a biologic invasion: 75 years postinvasion, cane toads and sympatric Australian frogs retain separate lungworm faunas. Journal of Wildlife Diseases 48, 951-961.
| Crossref | Google Scholar |
Price-Rees SJ, Brown GP, Shine R (2010) Predation on toxic cane toads (Bufo marinus) may imperil bluetongue lizards (Tiliqua scincoides intermedia, Scincidae) in tropical Australia. Wildlife Research 37, 166-173.
| Crossref | Google Scholar |
Reardon JT, Kraus F, Moore M, Rabenantenaina L, Rabinivo A, Rakotoarisoa NH, Randrianasolo HH (2018) Testing tools for eradicating the invasive toad Duttaphrynus melanostictus in Madagascar. Conservation Evidence 15, 12-19.
| Google Scholar |
Reilly SB, Wogan GOU, Stubbs AL, Arida E, Iskandar DT, McGuire JA (2017) Toxic toad invasion of Wallacea: a biodiversity hotspot characterized by extraordinary endemism. Global Change Biology 23, 5029-5031.
| Crossref | Google Scholar |
Roberts PD, Diaz-Soltero H, Hemming DJ, Parr MJ, Wakefield NH, Wright HJ (2013) What is the evidence that invasive species are a significant contributor to the decline or loss of threatened species? A systematic review map. Environmental Evidence 2, 5-7.
| Crossref | Google Scholar |
Rollins LA, Richardson MF, Shine R (2015) A genetic perspective on rapid evolution in cane toads (Rhinella marina). Molecular Ecology 24, 2264-2276.
| Crossref | Google Scholar |
Saidapur SK, Girish S (2001) Growth and metamorphosis of Bufo melanostictus tadpoles: effects of kinship and density. Journal of Herpetology 249-254.
| Crossref | Google Scholar |
Sales LP, Reboucas R, Toledo LF (2021) Native range climate is insufficient to predict anuran invasive potential. Biological Invasions 23, 2635-2647.
| Crossref | Google Scholar |
Saunders G, Cooke B, McColl K, Shine R, Peacock T (2010) Modern approaches for the biological control of vertebrate pests: an Australian perspective. Biological Control 52, 288-295.
| Crossref | Google Scholar |
Schwarzkopf L, Alford RA (2002) Nomadic movement in tropical toads. Oikos 96, 492-506.
| Crossref | Google Scholar |
Semeniuk M, Lemckert F, Shine R (2007) Breeding-site selection by cane toads (Bufo marinus) and native frogs in northern new south wales, Australia. Wildlife Research 34, 59-66.
| Crossref | Google Scholar |
Shine R (2010) The ecological impact of invasive cane toads (Bufo marinus) in Australia. The Quarterly Review of Biology 85, 253-291.
| Crossref | Google Scholar |
Shine R, Everitt C, Woods D, Pearson DJ (2018) An evaluation of methods used to cull invasive cane toads in tropical Australia. Journal of Pest Science 91, 1081-1091.
| Crossref | Google Scholar |
Shulman MJ, Ogden JC, Ebersole JP, McFarland WN, Miller SL, Wolf NG (1983) Priority effects in the recruitment of juvenile coral reef fishes. Ecology 64, 1508-1513.
| Crossref | Google Scholar |
Simberloff D, Martin J-L, Genovesi P, Maris V, Wardle DA, Aronson J, Courchamp F, Galil B, García-Berthou E, Pascal M, Pyšek P, Sousa R, Tabacchi E, Vilà M (2013) Impacts of biological invasions: what’s what and the way forward. Trends in Ecology & Evolution 28, 58-66.
| Crossref | Google Scholar |
Simha A, Pardo-De la Hoz CJ, Carley L (2022) Moving beyond the “diversity paradox”: the limitations of competition-based frameworks in understanding species diversity. The American Naturalist 200, 89-100.
| Crossref | Google Scholar |
Sinha B, Chakravorty P, Borah MM, Bordoloi S (2001) Qualitative analysis of food spectrum of five species of anuran tadpoles from arunachal pradesh, India. Zoos’ Print Journal 16, 514-515.
| Crossref | Google Scholar |
Slade RW, Moritz C (1998) Phylogeography of Bufo marinus from its natural and introduced ranges. Proceedings of the Royal Society of London. Series B: Biological Sciences 265, 769-777.
| Crossref | Google Scholar |
Solís F, Ibáñez R, Hammerson G, Hedges B, Diesmos A, Matsui M (2009) Rhinella marina. The IUCN red list of threatened species. Available at https://www.iucnredlist.org/species/41065/10382424
Southwell D, Tingley R, Bode M, Nicholson E, Phillips BL (2017) Cost and feasibility of a barrier to halt the spread of invasive cane toads in arid Australia: incorporating expert knowledge into model-based decision-making. Journal of Applied Ecology 54, 216-224.
| Crossref | Google Scholar |
Tan J, Pu Z, Ryberg WA, Jiang L (2012) Species phylogenetic relatedness, priority effects, and ecosystem functioning. Ecology 93, 1164-1172.
| Crossref | Google Scholar |
Taylor A, McCallum HI, Watson G, Grigg GC (2017) Impact of cane toads on a community of Australian native frogs, determined by 10 years of automated identification and logging of calling behaviour. Journal of Applied Ecology 54, 2000-2010.
| Crossref | Google Scholar |
Thuiller W, Lavorel S, Araújo MB (2005) Niche properties and geographical extent as predictors of species sensitivity to climate change. Global Ecology and Biogeography 14, 347-357.
| Crossref | Google Scholar |
Tingley R, Romagosa CM, Kraus F, Bickford D, Phillips BL, Shine R (2010) The frog filter: amphibian introduction bias driven by taxonomy, body size and biogeography. Global Ecology and Biogeography 19, 496-503.
| Crossref | Google Scholar |
Tingley R, Phillips BL, Letnic M, Brown GP, Shine R, Baird SJE (2013) Identifying optimal barriers to halt the invasion of cane toads Rhinella marina in arid Australia. Journal of Applied Ecology 50, 129-137.
| Crossref | Google Scholar |
Tingley R, Vallinoto M, Sequeira F, Kearney MR (2014) Realized niche shift during a global biological invasion. Proceedings of the National Academy of Sciences 111, 10233-10238.
| Crossref | Google Scholar |
Tingley R, Ward-Fear G, Schwarzkopf L, Greenlees MJ, Phillips BL, Brown G, Clulow S, Webb J, Capon R, Sheppard A, Strive T, Tizard M, Shine R (2017) New weapons in the toad toolkit: a review of methods to control and mitigate the biodiversity impacts of invasive cane toads (Rhinella marina). The Quarterly Review of Biology 92, 123-149.
| Crossref | Google Scholar |
Tingley R, García-Díaz P, Arantes CRR, Cassey P (2018) Integrating transport pressure data and species distribution models to estimate invasion risk for alien stowaways. Ecography 41, 635-646.
| Crossref | Google Scholar |
Toomes A, García-Díaz P, Wittmann TA, Virtue J, Cassey P (2020) New aliens in Australia: 18 years of vertebrate interceptions. Wildlife Research 47, 55-67.
| Crossref | Google Scholar |
Urban MC, Phillips BL, Skelly DK, Shine R (2007) The cane toad’s (Chaunus [Bufo] marinus) increasing ability to invade Australia is revealed by a dynamically updated range model. Proceedings of the Royal Society B: Biological Sciences 274, 1413-1419.
| Crossref | Google Scholar |
van Dijk PP, Iskandar D, Lau MWN, Huiqing G, Baorong G, Kuangyang L, Wenhao C, Zhigang Y, Chan B, Dutta S (2004) Duttaphrynus melanostictus IUCN red list of threatened species. Available at https://www.iucnredlist.org/species/54707/86445591
van Winkel D, Lane J (2012) The invasive cane toad (Bufo marinus) in West New Britain, Papua New Guinea: observations and potential impacts on native wildlife. Biological Invasions 14, 1985-1990.
| Crossref | Google Scholar |
Vences M, Brown JL, Lathrop A, Rosa GM, Cameron A, Crottini A, Dolch R, Edmonds D, Freeman KLM, Glaw F, Grismer LL, Litvinchuk S, Milne MG, Moore M, Solofo JF, Noël J, Nguyen TQ, Ohler A, Randrianantoandro C, Raselimanana AP, van Leeuwen P, Wogan GOU, Ziegler T, Andreone F, Murphy RW (2017) Tracing a toad invasion: lack of mitochondrial DNA variation, haplotype origins, and potential distribution of introduced Duttaphrynus melanostictus in Madagascar. Amphibia-Reptilia 38, 197-207.
| Crossref | Google Scholar |
Vidal-García M, Keogh JS (2017) Invasive cane toads are unique in shape but overlap in ecological niche compared to Australian native frogs. Ecology and Evolution 7, 7609-7619.
| Crossref | Google Scholar |
Von Holle B, Simberloff D (2005) Ecological resistance to biological invasion overwhelmed by propagule pressure. Ecology 86, 3212-3218.
| Crossref | Google Scholar |
Wainwright CE, Wolkovich EM, Cleland EE (2012) Seasonal priority effects: implications for invasion and restoration in a semi-arid system. Journal of Applied Ecology 49, 234-241.
| Crossref | Google Scholar |
Wijethunga U, Greenlees M, Shine R (2015) The acid test: pH tolerance of the eggs and larvae of the invasive cane toad (Rhinella marina) in southeastern Australia. Physiological and Biochemical Zoology 88, 433-443.
| Crossref | Google Scholar |
Wijethunga U, Greenlees M, Shine R (2016) Moving south: effects of water temperatures on the larval development of invasive cane toads (Rhinella marina) in cool-temperate Australia. Ecology and Evolution 6, 6993-7003.
| Crossref | Google Scholar |
Wogan GOU, Stuart BL, Iskandar DT, McGuire JA (2016) Deep genetic structure and ecological divergence in a widespread human commensal toad. Biology Letters 12, 20150807.
| Crossref | Google Scholar |
Woinarski JCZ, Braby MF, Burbidge AA, Coates D, Garnett ST, Fensham RJ, Legge SM, McKenzie NL, Silcock JL, Murphy BP (2019) Reading the black book: the number, timing, distribution and causes of listed extinctions in Australia. Biological Conservation 239, 108261.
| Crossref | Google Scholar |
Yu F, Groen TA, Wang T, Skidmore AK, Huang J, Ma K (2017) Climatic niche breadth can explain variation in geographical range size of alpine and subalpine plants. International Journal of Geographical Information Science 31, 190-212.
| Crossref | Google Scholar |
Zug GR, Zug PB (1979) The marine toad, Bufo marinus: a natural history resume of native populations. Smithsonian Contributions to Zoology 284, 1-58.
| Crossref | Google Scholar |