Can simple, on-ground vegetation and soil measures reliably indicate the health of rangelands? An application in Australia’s semi-arid woodlands
John A. Ludwig A D * , David J. Tongway B and Norman Hindley CA Retired.
B Retired.
C Deceased.
D Present address:
Abstract
Sustainably managing grazing lands is aided by monitoring and responding to simple and reliable indicators of how well the vegetation and soils of these landscapes are functioning to capture scarce resources such as water and nutrients. Indicators are needed because direct measurement of resource capture is time consuming and costly. Our aim was to assess how simple measures of vegetation patch cover and size, and soil surface condition, would apply to patchy (run-on/run-off) semi-arid landscapes being grazed at different intensities. We used the grazing gradient design where distance from water serves as a surrogate for grazing intensity, which is a combination of herbage consumption and trampling. From 0.5 to 8.9 km distance from water, we measured vegetation and soil indicators of landscape function on 12 sites, six along a grazing gradient in a mulga (Acacia aneura) woodland and six in a gidgee (Acacia cambagei) woodland. We found the size of tree groves, at both mulga and gidgee sites, declined near water, indicating a loss in the capacity of these groves to capture mobile resources in run-off. Enhancing this capacity at sites in ‘good’ rangeland condition was the presence of a thick band of grass upslope of tree groves. The number of soil erosion features (rills) was also a reliable indicator of landscape function at both gidgee and mulga sites. Soil surface condition indices of stability, infiltration and nutrient cycling had no detectable trends with distance from water at gidgee and mulga sites, but these three indicator values were always significantly higher within groves than inter-groves, confirming the important role of maintaining healthy groves of trees and upslope bands of grass within these semi-arid rangelands.
Keywords: Acacia aneura, Acacia cambagei, gidgee woodlands, grazing gradients, landscape function, mulga woodlands, rangeland health, soil erosion.
Introduction
As human populations increase the demand for ecosystem services from grazed drylands, there is a growing global recognition of the need to maintain healthy vegetation and soils in rangelands; see, for example, Adhikari and Hartemink (2016) (a global review), Damdinsuren et al. (2008) (Mongolia), Duff et al. (2009) (northern Australia), and Printz et al. (2014) (Great Plains, USA). Effectively managing the health of rangelands requires monitoring vegetation and soils of grazed landscapes to assess how well they function to conserve and use limited resources such as water, nutrients and organic matter (Tongway and Ludwig 1997a, 1997b), and provide habitats (food and shelter) for valuable plants and animals (Morton 1993; James et al. 1995). Although directly and repeatedly measuring how rangelands function to conserve limited resources can be achieved by scientific study (e.g. Bartley et al. 2014), such monitoring is time consuming and costly. Simple measures are needed to reliably indicate rangeland health in terms of how well grazed landscapes function to conserve water and nutrients.
Identifying simple yet reliable indicators of rangeland health and function has proven challenging globally (Eldridge et al. 2020) and locally, for example, in northern Australia (Tongway and Hindley 1995), in the USA Southwest and Great Basin (Whitford et al. 1998; de Soyza et al. 2000), and in central Iran (Safaei et al. 2019). The health of rangelands within the semi-arid woodlands of eastern Australia has proven especially difficult to assess because of their patchy, run-on/run-off vegetation structure where trees form groves and bands across an open landscape (Ludwig and Tongway 1995; Tongway and Ludwig 2001). These rangelands have erratic rainfall, highly weathered soils, and are susceptible to woody encroachment (Harrington et al. 1984). Forage and animal production decline if shrubs increase (Noble 1997), and if the condition of the soil surface decreases (Tongway and Smith 1989).
Although remotely sensed indicators of landscape function have been developed, applied and evaluated for monitoring rangeland health at large scales (e.g. Bastin et al. 2023; Safaei et al. 2023), there remains a need for simple, yet reliable, indicators of changes in vegetation and soil for on-ground monitoring at fine, management-oriented scales (Ludwig et al. 2007), such as around paddock water-points. Distance from water effectively serves as a surrogate for grazing intensity where herbage consumption and trampling impacts on vegetation and soils decline as distance increases (i.e. grazing gradients; Bastin et al. 1993).
In Australia, simple measures of the density, cover, and sizes of perennial vegetation patches and of the condition of soil surfaces have proven useful for reliably indicating how well landscapes function to conserve resources, such as, for example, in arid lands (Sparrow et al. 2003), in semi-arid woodlands (Tongway and Ludwig 1997a, 1997b) and in tropical savannas (Ludwig et al. 1999). These landscapes were characterised by having relatively gentle topography. However, a question remains of whether simple indicators of landscape function can also reliably assess rangeland health in semi-arid woodlands with more rugged landscapes featuring undulating plains with rock outcrops and incised ephemeral drainages?
In this paper, we assess whether simple measures of vegetation patch number, cover and size, and soil surface condition, reliably indicate rangeland health in the rugged semi-arid woodlands of south-western Queensland. We specifically looked for simple measures that would indicate a loss of landscape function near water points where livestock grazing intensities are highest. Simple and reliable indicators would provide pastoralists with useful tools for monitoring and managing the health of their grazing lands.
Study areas and methods
Two areas were studied in October 1995 within the rugged, patchy semi-arid woodlands of south-western Queensland in eastern Australia (Fig. 1), one characterised by groves of mulga (Acacia aneura F.Muell. ex Benth.), the other by groves of gidgee (Acacia cambagei R.T.Baker). These two study areas have been previously described in detail by Landsberg et al. (1999, 2003). Briefly, this semi-arid region has a mean annual rainfall of about 265 mm (Clewett et al. 1994). Rainfall is highly erratic, but, on average, more rainfall is recorded in the 6 months from November to April. Soils in the area have been described as hard-setting, massive red earths of low fertility (Webb et al. 1980). The mulga study area at 25.9°S, 143.3–143.4°E is within a paddock developed for grazing sheep in the late 1800s. Vegetation is characterised by open bare or grassy areas between groves of mulga, and soils are gravelly red earths on rough terrain with undulating plains and incised ephemeral drainages. The gidgee study area is within a very large paddock located at 26.7°S–26.8°S, 143.4°E, about 130 km south of the mulga study area. The paddock was fenced in the late 1800s for grazing by cattle and later by sheep. The vegetation has groves and discontinuous bands of gidgee separated by open areas of herbs, grasses and low shrubs. Soils are mostly red earths, but with brown cracking clays in and around depressions and sink holes (gilgai). Landscape consists of rough, dissected, and undulating pediments.
Top graph shows the location of mulga and gidgee study areas within the semi-arid woodlands of eastern Australia (after Harrington et al. 1984). Bottom graph illustrates a grazing-gradient design, with sites located at increasing distances from a water-point. Distances shown approximate those applied in this study.
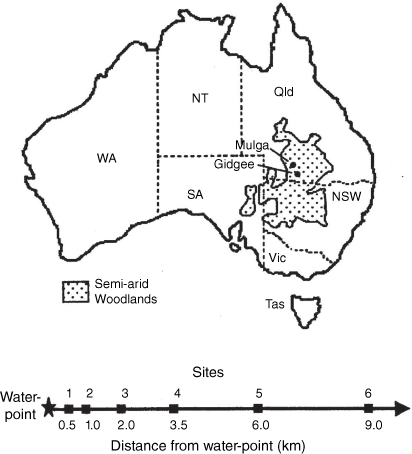
In each study area, six sites were located at an increasing distance from a water-point (up to ~9 km; Fig. 1). More sites were located closer to water where changes due to increased grazing intensity can occur rapidly. The grazing gradient design assumes that in arid and semi-arid environments, cattle and sheep are drinking water from a single location provided by an installed water-point. Hence, grazing and trampling impacts will be highest near water due to livestock social behaviour, and impacts significantly diminish away from water because animals fan out, creating what has been termed the piosphere effect (Lange 1969; Graetz and Ludwig 1978).
Five tree groves were observed at each site. Groves were classified according to their health relative to tree dieback and rangeland condition (Table 1). Trees within a grove were visually assessed for the degree of leafiness and branch death. For example, gidgee groves close to water were reduced in size to having a few trees with stems of low leafiness and mostly dead branches (Fig. 2).
Condition | Description | |
---|---|---|
Good | Healthy tree groves, with tree regeneration and no signs of tree dieback. Herbaceous ground cover of >70%, with good cover of 3P (i.e. perennial, palatable, productive) grasses, few weedy forbs, ephemeral grasses and subshrubs, no shrub thickening, no signs of excessive runoff and soil erosion. | |
Fair | Similar to Good, but with generally healthy tree groves with some regeneration and with dieback of <10%. Ground cover of 40–70%, less cover of 3P grasses, more ephemeral grasses, forbs and subshrubs, some shrub thickening, some signs of erosion (e.g. rills). | |
Poor | Similar to Fair, but with unhealthy tree groves with little or no regeneration and with tree dieback of >10%. Ground cover of <40%, low cover of 3P grasses, mostly ephemeral grasses, weedy forbs and subshrubs, clear signs of shrub thickening, obvious signs of excessive runoff and soil erosion. | |
Very poor | Tree groves with no regeneration and total or near-total dieback. Mostly bare ground, with lack of perennial grasses, forbs, and subshrubs except for possible thickening of unpalatable shrubs, signs of severe erosion (gullies, rills). |
Five open inter-grove sites, located between the five groves, and off the end of either the first or fifth grove, were also classified in terms their rangeland condition (Table 1). Within each of the five groves and five inter-groves at each site, 11 soil surface-condition attributes (Appendix 1) were assessed within five quadrats (0.25 m2) by using landscape function analysis (LFA) procedures (Tongway and Hindley 2004; Eldridge et al. 2020). From these quadrat assessments, three soil surface-condition indices (stability, infiltration, nutrient cycling) were calculated as described in Appendix 1. To confirm nutrient assessments, and evaluate general site fertility, soil samples were collected from 0–1 and 0–10 cm within each grove and inter-grove. The fertility of these soils, in terms of Kjeldahl nitrogen (KN) and organic carbon (OC), was measured in the laboratory by standard procedures.
At each of the six mulga and six gidgee sites, a line transect was oriented down-slope (Tongway and Ludwig 1997b), from a high point (e.g. ridge line, rock outcrop) to a low point (e.g. ephemeral drainage line). For every tree grove intercepted by a line transect, we estimated its potential to obstruct downslope surface flows (run-off) by measuring its maximum width perpendicular to the slope. We also measured the intercept cover (% of total line) of run-on features, including tree groves, clumps of shrub, and patches and bands of perennial grasses as well as smaller surface features such as piles of litter, rocks, log mounds formed from fallen trees, and soil mounds formed by ants and termites. If a band of perennial grasses occurred upslope of a tree grove intercepted by the line transect, its % cover was also measured. To test the statistical significance of linear regressions and group mean differences, we used Systat software (SigmaPlot: exact graphics for exact science, ver. 6, https://systatsoftware.com/sigmaplot/).
Soil-erosion features, such as rills, were also recorded along line transects at sites. The roughness of the soil surfaces was measured by surveying surface levels at half-metre intervals along the 12 line transects. Surface roughness was calculated as the sum of differences between the level at a point relative to that for the two points on either side of it, which we termed local surface roughness.
Results
The health of tree groves, as assessed by dieback and rangeland-condition criteria for five groves at each of the six mulga and six gidgee study areas, was classified as ‘very poor’ or ‘poor’ close to water-points and ‘good’ at about 9 km from water (Table 2). The health of open inter-grove areas had a similar trend from ‘very poor’ or ‘poor’ to ‘good’ with an increasing distance from water. Soil-surface condition indices for stability, infiltration and nutrients did not have any significant linear regression trends with distance from water, but mean condition values within tree groves were always significantly greater (P < 0.05) than were mean values within open inter-grove areas.
Woodland and attribute | Patch type | Study site | ||||||
---|---|---|---|---|---|---|---|---|
1 | 2 | 3 | 4 | 5 | 6 | |||
Mulga | ||||||||
Distance from water-point (km) | 0.5 | 1.1 | 2.0 | 3.7 | 6.0 | 8.9 | ||
Vegetation health | Tree groves | Poor | Poor | Fair | Fair | Fair | Good | |
Inter-groves | Poor | Poor | Poor | Fair | Fair | Good | ||
Stability indexA | Tree groves | 55.5 | 72.2 | 65.0 | 62.5 | 55.5 | 60.0 | |
Inter-groves | 40.0 | 47.2 | 56.2 | 42.5 | 40.0 | 41.2 | ||
Infiltration indexA | Tree groves | 40.7 | 48.1 | 40.7 | 48.1 | 48.1 | 50.0 | |
Inter-groves | 25.9 | 31.4 | 31.4 | 29.6 | 29.6 | 29.6 | ||
Nutrient indexA | Tree groves | 31.9 | 30.2 | 29.6 | 41.8 | 46.5 | 35.4 | |
Inter-groves | 11.6 | 12.7 | 12.7 | 13.9 | 11.6 | 12.7 | ||
Gidgee | ||||||||
Distance from water-point (km) | 0.7 | 1.2 | 1.8 | 3.7 | 5.5 | 8.9 | ||
Vegetation health | Tree groves | Very poor | Poor | Poor | Fair | Fair | Good | |
Inter-groves | Very poor | Very poor | Poor | Fair | Fair | Good | ||
Stability indexA | Tree groves | 50.0 | 62.5 | 60.0 | 60.0 | 57.5 | 47.5 | |
Inter-groves | 47.5 | 45.0 | 47.6 | 43.7 | 47.5 | 37.5 | ||
Infiltration indexA | Tree groves | 37.0 | 37.0 | 44.4 | 33.3 | 44.4 | 40.7 | |
Inter-groves | 29.6 | 25.9 | 31.4 | 25.9 | 29.6 | 29.6 | ||
Nutrient indexA | Tree groves | 18.6 | 24.4 | 41.8 | 20.9 | 25.5 | 22.0 | |
Inter-groves | 12.7 | 13.9 | 13.9 | 12.7 | 16.2 | 13.9 |
The length of line transect surveyed at the 12 sites varied from 129 to 581 m (Table 3), and depended on the distance from a high point in the landscape (ridge, outcrop) downslope to a low point (drainage). The steepness of slope at sites was <1.5% except for gidgee Site 4 (3.7%). The local surface roughness of mulga and gidgee sites was <10 mm except for gidgee Sites 1 and 4 at 10.4 and 13.0 mm respectively. At each site, we estimated the linear density of tree groves, that is, the number of groves at a site intercepted by the line transect, which was ~2/100 m, but varied from 1.1/100 to 2.7/100 m.
Woodland and attribute | Patch type | Study site | ||||||
---|---|---|---|---|---|---|---|---|
1 | 2 | 3 | 4 | 5 | 6 | |||
Mulga | ||||||||
Distance from water-point (km) | 0.5 | 1.1 | 2.0 | 3.7 | 6.0 | 8.9 | ||
Length of transect surveyed (m) | 450 | 581 | 397 | 352 | 407 | 283 | ||
Slope along line-transect (%) | 1.0 | 0.7 | 1.2 | 0.4 | 0.4 | 1.4 | ||
Local surface roughness (mm) | 6.1 | 8.1 | 8.4 | 7.6 | 9.5 | 8.7 | ||
Grove linear-density (#/100 m) | 2.0 | 1.5 | 2.3 | 2.3 | 2.5 | 1.8 | ||
Cover (%) | Tree groves | 24.9 | 14.0 | 15.6 | 30.9 | 32.5 | 27.5 | |
Open slopes | 46.5 | 65.9 | 67.8 | 45.5 | 46.7 | 58.3 | ||
Grass bands | 5.4 | 4.2 | 5.0 | 2.4 | 6.7 | 5.6 | ||
Soil mounds | 1.8 | 4.4 | 4.2 | 2.5 | 1.1 | 1.9 | ||
Other obstructionsA | 21.4 | 11.5 | 7.4 | 18.7 | 13.0 | 6.7 | ||
Gidgee | ||||||||
Distance from water-point (km) | 0.7 | 1.2 | 1.8 | 3.7 | 5.5 | 8.9 | ||
Length of transect surveyed (m) | 223 | 171 | 580 | 242 | 129 | 357 | ||
Slope along line-transect (%) | 1.0 | 0.6 | 1.0 | 3.7 | 1.4 | 0.7 | ||
Local surface roughness (mm) | 10.4 | 5.6 | 7.8 | 13.0 | 5.8 | 4.7 | ||
Grove linear-density (#/100 m) | 2.7 | 2.3 | 1.7 | 2.5 | 1.6 | 1.1 | ||
Cover (%) | Tree groves | 9.4 | 8.5 | 8.6 | 19.5 | 10.2 | 9.4 | |
Open slopes | 57.6 | 70.4 | 55.2 | 54.8 | 75.8 | 78.4 | ||
Grass bands | 0.7 | 0.0 | 3.6 | 9.6 | 4.1 | 1.5 | ||
Soil mounds | 4.4 | 1.5 | 4.0 | 6.4 | 0.0 | 4.5 | ||
Other obstructionsA | 27.9 | 19.6 | 28.6 | 9.7 | 9.9 | 6.2 |
The linear density and cover of groves of trees and the cover of inter-grove grass bands, soil mounds and other patches that obstruct surface flows are reported for sites located at greater distances from water.
The relative cover of tree groves at sites (Table 3), as estimated from the length of line intercepted by groves as a percentage of the total length, was >14% at mulga sites and <11% at gidgee sites except at gidgee Site 4 (19.5%). Tree groves located just upslope of drainage lines often took the form of a band of trees. The areas between tree groves (inter-groves) were mostly open slopes (45.5–78.4%), which provided little obstruction to runoff during rain events. Inter-grove areas also had features that effectively slowed or captured run-off, termed run-on areas. These included soil mounds formed by individual shrubs, fallen logs and termites (0.0–6.6% cover). Other features in these landscapes that obstructed and captured run-off (6.2–28.6% cover) included small depressions or sink-holes (gilgai), ant and other animal holes, and patches of litter. Gilgai were a feature of gidgee sites.
From line-transect data surveyed at the 12 sites, we found that the average size of tree groves, as estimated from line-intercept lengths, tended to increase with distance from water-points (Fig. 3a). The positive linear trend in gidgee grove size with distance was statistically significant (P = 0.02), whereas the positive trend for mulga groves was weak (P = 0.10). The average size of mulga groves was larger than that of gidgee groves at distances from 0.5 to 8.9 km.
For distance from water: (a) size of mulga and gidgee tree groves, as estimated by the mean length (m) of line-transect intercepted by groves at each site, (b) percentage of mulga and gidgee tree groves with distinctive grass bands located upslope of groves, and (c) soil erosion rills intercepted along line transects (%) at sites.
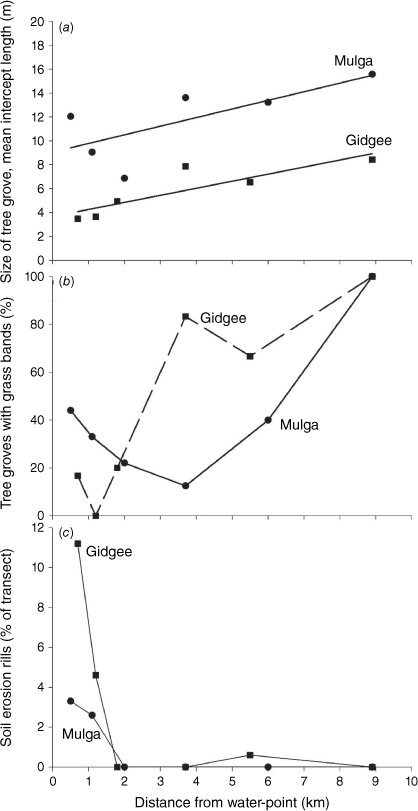
Most tree groves in these semi-arid landscapes were also characterised by having a band of grass located just upslope of the grove (0.0–9.6% cover; Table 3). Importantly, 100% of the tree groves at mulga and gidgee sites in ‘good’ condition (8.9 km from water) had a thick and healthy band of perennial grasses upslope of groves (Fig. 3b). A lower percentage of groves had an upslope band of grass at gidgee sites within 2 km of water-points (<25%), and for mulga sites within 6 km (<45%). When these grass bands within inter-groves did occur above a grove closer to water, they were in ‘very poor’ or ‘poor’ rangeland condition (Table 2).
The presence and length of line transect intercepting soil erosion rills at mulga and gidgee sites were notably within 2 km of a water-point (Fig. 3c); beyond 2 km, no rills were recorded, except for 0.6% at gidgee Site 5. Close to water, the percentage cover of erosion rills on gidgee Sites 1 and 2 was higher than on mulga Sites 1 and 2, which contributed to the gidgee sites being assessed as being in ‘very poor’ rangeland condition’ (Table 2).
Because soil sampling and obtaining soil characteristics and chemistry data are time consuming and costly, they are not useful as simple soil-health indicators. However, these data are useful for confirming the reliability of related indicators. We found that soil fertility, as measured by organic carbon (OC) and Kjeldahl nitrogen (KN) concentrations (%), was always significantly higher within tree groves than within inter-groves (Table 4). This finding indicated the importance of maintaining healthy tree groves in these landscapes. As expected, soil fertility in the 0–1-cm of soil was consistently greater than that in soil samples collected down to 10 cm.
Woodland and attribute | Patch type | Study site | ||||||
---|---|---|---|---|---|---|---|---|
1 | 2 | 3 | 4 | 5 | 6 | |||
Mulga | ||||||||
Distance from water-point (km) | 0.5 | 1.1 | 2.0 | 3.7 | 6.0 | 8.9 | ||
OC, 0–1 cm (%)A | Tree groves | 1.21 | 1.51 | 1.91 | 1.35 | 2.53 | 1.86 | |
Open slopes | 0.28 | 0.39 | 0.47 | 0.42 | 0.40 | 0.48 | ||
OC, 0–10 cm (%)A | Tree groves | 0.64 | 0.61 | 0.78 | 0.56 | 1.24 | 0.77 | |
Open slopes | 0.18 | 0.32 | 0.37 | 0.36 | 0.39 | 41.2 | ||
KN, 0–1 cm (%)A | Tree groves | 0.094 | 0.104 | 0.132 | 0.106 | 0.172 | 0.136 | |
Open slopes | 0.017 | 0.027 | 0.045 | 0.046 | 0.036 | 0.036 | ||
KN, 0–10 cm (%)A | Tree groves | 0.043 | 0.039 | 0.046 | 0.036 | 0.074 | 0.048 | |
Open slopes | 0.006 | 0.021 | 0.023 | 0.026 | 0.024 | 0.016 | ||
Gidgee | ||||||||
Distance from water-point (km) | 0.7 | 1.2 | 1.8 | 3.7 | 5.5 | 8.9 | ||
OC, 0–1 cm (%)A | Tree groves | 0.94 | 0.63 | 2.01 | 2.07 | 1.33 | 2.32 | |
Open slopes | 0.29 | 0.16 | 0.31 | 0.38 | 0.43 | 0.28 | ||
OC, 0–10 cm (%)A | Tree groves | 1.17 | 0.64 | 1.29 | 1.85 | 0.72 | 1.26 | |
Open slopes | 0.32 | 0.13 | 0.34 | 0.49 | 0.45 | 0.25 | ||
KN, 0–1 cm (%)A | Tree groves | 0.070 | 0.047 | 0.159 | 0.177 | 0.094 | 0.196 | |
Open slopes | 0.016 | 0.005 | 0.014 | 0.024 | 0.028 | 0.015 | ||
KN, 0–10 cm (%)A | Tree groves | 0.083 | 0.047 | 0.089 | 0.148 | 0.051 | 0.101 | |
Open slopes | 0.014 | 0.010 | 0.024 | 0.035 | 0.026 | 0.017 |
We also found that soil OC and KN concentrations (%) in the 0–1-cm significantly increased (P = 0.05) with the size of gidgee groves (Fig. 4a). This statistically significant trend was largely due to the fact that smaller gidgee groves (<5 m of line intercept) had low concentrations of OC (<1%) and KN (<0.1%). This positive relationship for gidgee groves did not hold for mulga groves where OC and KN concentration varied widely with grove size from 6 to 16 m of line intercept.
Organic carbon (OC) and Kjeldahl nitrogen (KN) concentrations (%) in the 0–1-cm of soils sampled in mulga and gidgee groves differing in (a) size as estimated by the mean length (m) of line transect intercepted by groves at each site, and (b) distance from water-point (km).
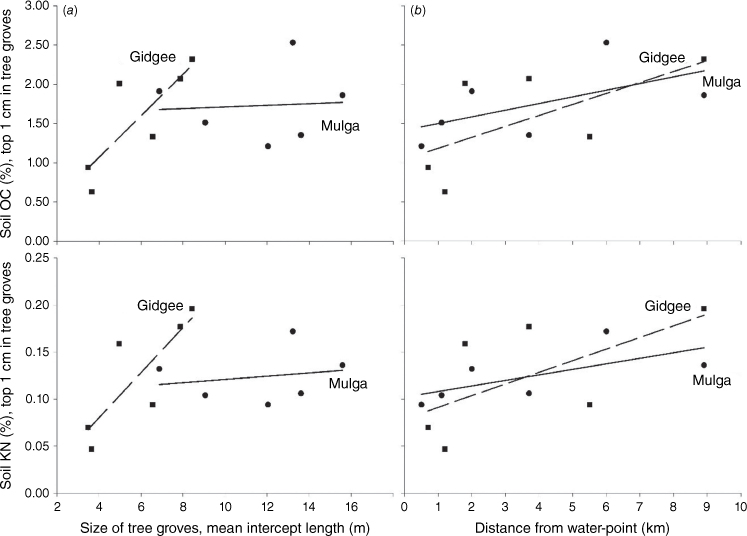
Soil OC and KN also appeared to increase with distance from water at mulga and gidgee sites (Fig. 4b); however, these trend lines were significant only at P-values ranging from P = 0.15 to P = 0.23, owing to low sample sizes (N = 6). Similarly, also likely owing to small sample size, the nutrient-cycling index assessed at 12 sites had a positive slope (+6.0) with OC in the 0–1-cm [NCI = 20.9 + (6.0 × OC)], but with P = 0.23. The nutrient-cycling index also had a positive slope (+48.4) with KN in the top 1 cm [NCI = 24.7 + (48.4 × KN)], but with P = 0.44.
Discussion
In the rugged and patchy mulga and gidgee semi-arid woodlands of eastern Australia, we applied a rapid, qualitative, on-ground assessment of the health of tree groves by using established tree dieback and rangeland-condition criteria that are known to reliably indicate the health of landscapes (Landsberg 1985; Rice et al. 2004; Beutel et al. 2021). Using these criteria and a grazing-gradient approach, mulga and gidgee landscapes located well away from water-points (8.9 km) were assessed to be in ‘good’ health, whereas those close to water (<2 km) were in ‘poor’ or ‘very poor’ health.
In this study, the size of mulga and gidgee tree groves declined near water-points, reducing their ability to function by capturing and utilising limited water and nutrients in soils flowing and blowing around the landscape. Groves of mulga and gidgee trees furthest from water were not only larger and in ‘good’ health, but they also had a band of healthy perennial grasses located upslope of groves, compared with none or fewer close to water where soil surfaces were bare and eroded into rills. Thus, if vegetation patches such as tree groves fail to function by capturing soil sediments, the concentration of soil nutrients in these patches is likely to decrease with time. Here we found that mulga and gidgee groves had significantly higher concentrations of organic carbon and nitrogen in their surface soils than did soils in inter-groves, confirming that tree patches function to retain and concentrate resources. These ‘fertile islands’ have been described for other patchy landscapes in semi-arid rangelands (e.g. Schlesinger et al. 1996; Whitford 2002).
The reduction of landscape function has important implications for the sustainable management of arid and semi-arid rangelands (Ludwig and Freudenberger 1997; Eldridge et al. 2017). The preservation of perennial vegetation patches is critical for maintaining landscape functions such as conservation and use of limited resources (Tongway and Ludwig 1997a), and the provision of habitats for a wide diversity of organisms (e.g. James et al. 1995), particularly because it is more cost effective to prevent landscape degradation than it is to restore it (Ludwig 1999).
In this 1995 study, we applied LFA procedures along a grazing gradient. Underlying LFA is the concept of landscape functionality related to the role of vegetation patches on rangeland health, which was developed in Australia (Tongway and Ludwig 1997a, 1997b), and then applied elsewhere in Australia by Friedel (1997). To evaluate landscape functionality, LFA has now been applied to rangelands by researchers in many different countries (Whitford et al. 1998, and De Soyza et al. 2000, in western USA; Smet and Ward 2006, in South Africa; Hoshino et al. 2009, in Mongolia; Manthey and Peper 2010, in Azerbaijan; and Zimmer et al. 2024, in Namibia). Notably, in a global study evaluating LFA (Eldridge et al. 2020), the authors found that easily assessed litter cover, and its degree of incorporation into the soil, and the stability of the soil surface (e.g. largely due to biocrust cover), were useful indicators of soil functionality and health in grazed drylands worldwide.
In this study, LFA soil-surface condition indices for stability, infiltration and nutrient cycling did not significantly improve in value with distance from water-points (less grazing intensity), as expected from other LFA studies in semi-arid rangelands in eastern Australia (e.g. Eldridge et al. 2017). Other LFA studies examined more sites than in our study where time constrained us to two sites with six transects at different distances from water-points. However, even with our limited sample sizes (N = 6), we found significantly higher LFA index values in mulga and gidgee tree groves than in open inter-grove spaces, which indicates the importance of these patches of trees in capturing resources.
For mulga sites, the soil-surface stability indices for tree groves were about 60, indicating a satisfactory level of surface condition relative to studies in other areas (Tongway 1995). The surface stability of the inter-groves was about 45, which is probably in a ‘safe’ range, given surfaces often had a stony-rocky armour. The surface infiltration potential in groves was <50, whereas values of >50 would indicate a greater capacity for these patches to capture water during run-off events. Nutrient-cycling potential was satisfactory in mulga groves, but was low within inter-groves (<15), indicating a lack of a potential for biological activity.
For gidgee sites, surface-stability index values for groves were about 10 units lower than those for mulga groves. From experience (Tongway 1995), it would be expected that they should be similar, suggesting that the gidgee sites studied here had a history of higher utilisation than did other gidgee country in the semi-arid woodlands of eastern Australia. The surface stability of gidgee inter-groves was ‘safe’, similar to that for mulga sites. Although infiltration-potential index values for soils in gidgee groves were higher than those for soils in inter-groves, differences were not large, and from experience elsewhere, values were barely adequate to effectively capture run-off. Nutrient-cycling potential was very low (mostly <25) in both gidgee groves and inter-groves, indicating a historic low growth and high utilisation of vegetation. The nutrient-cycling index was found to be a useful indicator of grazing-induced disturbances on rangeland health across a range of climatic zones in eastern Australia (Eldridge and Delgado-Baquerizo 2018).
Conclusions
To answer the question of whether simple, on-ground vegetation and soil measures can reliably indicate the health of rangelands in rugged, patchy semi-arid woodlands in eastern Australia, we identified four indicators. First, using tree dieback and rangeland-condition criteria, a monitoring site can be rapidly assessed into one of the following four health classes: ‘good’, ‘fair’, ‘poor’ or ‘very poor’.
Using LFA procedures along a grazing gradient, we identified two indicators of rangeland health related to vegetation patchiness, namely (1) size of tree groves and (2) the presence of a healthy band of perennial grasses upslope of groves. The size of tree groves, at both mulga and gidgee sites, declined near water, indicating a loss in their capacity to capture resources. Sites away from water in ‘good’ rangeland condition always had a thick band of grass upslope of tree groves, which enhances the capacity of sites to capture and utilise limited resources.
We identified a fourth easily observed indicator related to soil-surface condition, namely, the presence of soil-erosion rills. At both gidgee and mulga sites, rills were abundant at sites less than 2 km from water-points, whereas rills were absent or rare at sites more than 2 km from water-points.
We found that these four indicators were applicable for assessing the health of two different semi-arid rangeland sites characterised by vegetation and soils forming run-on/run-off patches within rugged landscapes. Because these four indicators can be easily and rapidly assessed, they can be used to frequently monitor a wide variety of rangeland landscapes, so as to detect any changes in health.
Data availability
The 1995 data used in this study are in public domain. Contact the corresponding author for availability.
Declaration of funding
At the time of this study, the authors were employed by CSIRO Wildlife & Ecology and did not receive any specific funding for this research. Field research conducted in October 1995 was supported in part by CSIRO colleague, Jill Landsberg, from a grant with the Land & Water Resources Research and Development Corporation.
Acknowledgements
We thank CSIRO for their support, and our CSIRO colleagues Jill Landsberg, Craig James, Steve Morton and Jacqui Stol for giving us the opportunity to conduct this field study in October 1995, and for providing logistic support, as part of their biodiversity study in the semi-arid woodlands of south-western Queensland. We also thank CSIRO colleague Rogert Eager for assisting with surface-roughness calculations. J. L. and D. T. thank reviewers who provided useful comments that improved this paper. D. T. and J. L. dedicate this paper to the memory of our coauthor and colleague Norman Hindley.
References
Adhikari K, Hartemink AE (2016) Linking soils to ecosystem services. A global review. Geoderma 262, 101-111.
| Crossref | Google Scholar |
Bartley R, Corfield JP, Hawdon AA, Kinsey-Henderson AE, Abbott BN, Wilkinson SN, Keen RJ (2014) Can changes to pasture management reduce runoff and sediment loss to the Great Barrier Reef? The results of a 10-year study in the Burdekin catchment, Australia. The Rangeland Journal 36, 67-84.
| Crossref | Google Scholar |
Bastin GN, Pickup G, Chewings VH, Pearce G (1993) Land degradation assessment in central Australia using a grazing gradient method. The Rangeland Journal 15, 190-216.
| Crossref | Google Scholar |
Bastin G, Cowley R, Friedel M, Materne C (2023) Applying two remotely-sensed methods for monitoring grazing impacts in the Australian arid zone. The Rangeland Journal 45, 141-159.
| Crossref | Google Scholar |
Beutel TS, Shepherd R, Karfs RA, Abbott BN, Eyre T, Hall TJ, Barbi E (2021) Is ground cover a useful indicator of grazing land condition? The Rangeland Journal 43, 55-64.
| Crossref | Google Scholar |
Damdinsuren B, Herrick JE, Pyke DA, Bestelmeyer BT, Havstad KM (2008) Is rangeland health relevant to Mongolia? Rangelands 30, 25-29.
| Crossref | Google Scholar |
De Soyza AG, Van Zee JW, Whitford W, Neale A, Tallent-Hallsel N, Herrick JE, Havstad KM (2000) Indicators of Great Basin rangeland health. Journal of Arid Environments 45, 289-304.
| Crossref | Google Scholar |
Duff G, Garnett D, Jacklyn P, Landsberg J, Ludwig J, Morrison J, Novelly P, Walker D, Whitehead P (2009) A collaborative design to adaptively manage for landscape sustainability in north Australia: lessons from a decade of cooperative research. Landscape Ecology 24, 1135-1143.
| Crossref | Google Scholar |
Eldridge DJ, Delgado-Baquerizo M (2018) Grazing reduces the capacity of Landscape Function Analysis to predict regional-scale nutrient availability or decomposition, but not total nutrient pools. Ecological Indicators 90, 494-501.
| Crossref | Google Scholar |
Eldridge DJ, Delgado-Baquerizo M, Travers SK, Val J, Oliver I (2017) Do grazing intensity and herbivore type affect soil health? Insights from a semi-arid productivity gradient. Journal of Applied Ecology 54, 976-985.
| Crossref | Google Scholar |
Eldridge DJ, Delgado-Baquerizo M, Quero JL, Ochoa V, Gozalo B, Garcia-Palacios P, Escolar C, Garcia-Gómez M, Prina A, Bowker MA, Bran DE, Castro I, Cea A, Derak M, Espinosa CI, Florentino A, Gaitán JJ, Gatica G, Gómez-González S, Ghiloufi W, Gutierrez JR, Gusmán-Montalván E, Hernández RM, Hughes FM, Muiño W, Monerris J, Ospina A, Ramirez DA, Ribas-Fernández YA, Romão RL, Tores-Dias C, Koen TB, Maestre FT (2020) Surface indicators are correlated with soil multifunctionality in global drylands. Journal of Applied Ecology 57, 424-435.
| Crossref | Google Scholar |
Friedel MH (1997) Discontinuous change in arid woodland and grassland vegetation along gradients of cattle grazing in central Australia. Journal of Arid Environments 37, 145-164.
| Crossref | Google Scholar |
Graetz RD, Ludwig JA (1978) A method for the analysis of piosphere data applicable to range assessment. The Rangeland Journal 1, 126-136.
| Crossref | Google Scholar |
Hoshino A, Yoshihara Y, Sasaki T, Okayasu T, Jamsran U, Okuro T, Takeuchi K (2009) Comparison of vegetation changes along grazing gradients with different numbers of livestock. Journal of Arid Environments 73, 687-690.
| Crossref | Google Scholar |
James CD, Landsberg J, Morton SR (1995) Ecological functioning in arid Australia and research to assist conservation of biodiversity. Pacific Conservation Biology 2, 126-142.
| Crossref | Google Scholar |
Landsberg J (1985) Drought and dieback of rural eucalypts. Austral Ecology 10, 87-90.
| Crossref | Google Scholar |
Landsberg J, Lavorel S, Stol J (1999) Grazing response groups among understory plants in arid rangelands. Journal of Vegetation Science 10, 683-696.
| Crossref | Google Scholar |
Landsberg J, James CD, Morton SR, Muller WJ, Stol J (2003) Abundance and composition of plant species along grazing gradients in Australian rangelands. Journal of Applied Ecology 40, 1008-1024.
| Crossref | Google Scholar |
Lange RT (1969) The piosphere, sheep track and dung patterns. Journal of Range Management 22, 396-400.
| Crossref | Google Scholar |
Ludwig JA, Tongway DJ (1995) Spatial organisation of landscapes and its function in semi-arid woodlands, Australia. Landscape Ecology 10, 51-63.
| Crossref | Google Scholar |
Ludwig JA, Eager RW, Williams RJ, Lowe LM (1999) Declines in vegetation patches, plant diversity, and grasshopper diversity near cattle watering-points in the Victoria River District, northern Australia. The Rangeland Journal 21, 135-149.
| Crossref | Google Scholar |
Ludwig JA, Bastin GN, Wallace JF, McVicar TR (2007) Assessing landscape health by scaling with remote sensing: when is it not enough? Landscape Ecology 22, 163-169.
| Crossref | Google Scholar |
Manthey M, Peper J (2010) Estimation of grazing intensity along grazing gradients – the bias of nonlinearity. Journal of Arid Environments 74, 1351-1354.
| Crossref | Google Scholar |
Morton SR (1993) Changing conservation perceptions in the Australian rangelands. The Rangeland Journal 15, 145-153.
| Crossref | Google Scholar |
Printz JL, Toledo D, Boltz SC (2014) Rangeland health assessment: the key to understanding and assessing rangeland soil health in the Northern Great Plains. Journal of Soil and Water Conservation 69, 73A-77A.
| Crossref | Google Scholar |
Rice KJ, Matzner SL, Byer W, Brown JR (2004) Patterns of tree dieback in Queensland, Australia: the importance of drought stress and the role of resistance to cavitation. Oecologia 139, 190-198.
| Crossref | Google Scholar | PubMed |
Safaei M, Bashari H, Mosaddeghi MR, Jafari R (2019) Assessing the impacts of land use and land cover changes on soil functions using landscape function analysis and soil quality indicators in semi-arid natural ecosystems. Catena 177, 260-271.
| Crossref | Google Scholar |
Safaei M, Bashari H, Kleinebecker T, Fakheran S, Jafari R, Grosse-Stolenberg A (2023) Mapping terrestrial ecosystem health in drylands: comparison of field-based information with remotely sensed data at watershed level. Landscape Ecology 38, 705-724.
| Crossref | Google Scholar |
Schlesinger WH, Raikes JA, Harley AE, Cross AE (1996) On the spatial pattern of sol nutrients in desert ecosystems. Ecology 77, 364-374.
| Crossref | Google Scholar |
Smet M, Ward D (2006) Soil quality gradients around water-points under different management systems in a semi-arid savanna, South Africa. Journal of Arid Environments 64, 251-269.
| Crossref | Google Scholar |
Sparrow AD, Friedel MH, Tongway DJ (2003) Degradation and recovery processes in arid grazing lands of central Australia. Part 3. Implications at landscape scale. Journal of Arid Environments 55, 349-360.
| Crossref | Google Scholar |
Tongway DJ (1995) Monitoring soil productive potential. Environmental Monitoring and Assessment 37, 303-318.
| Crossref | Google Scholar | PubMed |
Tongway DJ, Ludwig JA (2001) Theories on the origins, maintenance, dynamics and functioning of banded landscapes. In ‘Banded vegetation patterning in arid and semiarid environments: Ecological processes and consequences for management’. (Eds DJ Tongway, C Valentine, J Segheri) pp. 20–31. (Springer Science: New York, NY, USA)
Tongway DJ, Smith EL (1989) Soil surface features as indicators of rangeland site productivity. Australian Rangeland Journal 11, 15-20.
| Crossref | Google Scholar |
Webb AA, Walker PJ, Gunn RH, Mortlock AT (1980) Soils of the poplar box (Eucalyptus populnea) communities of eastern Australia. Australian Rangeland Journal 2, 17-30.
| Crossref | Google Scholar |
Whitford WG, De Soyza AG, Van Zee JW, Herrick JE, Havstad KM (1998) Vegetation, soil, and animal indicators of rangeland health. Environmental Monitoring and Assessment 51, 179-200.
| Crossref | Google Scholar |
Zimmer K, Amputu V, Schwarz L-M, Linstädter A, Sandhage-Hofmann A (2024) Soil characteristics within vegetation patches are sensitive indicators of savanna rangeland degradation in central Namibia. Geoderma Regional 36, e00771.
| Crossref | Google Scholar |
Appendix 1.Attributes used to assess three soil-surface condition (SSC) indices: surface stability, nutrient-cycling and infiltration potentials (after Tongway 1995; Tongway and Hindley 2004; procedures available on request at lfa_procedures@iinet.net.au). The interpretation and relevance of attributes courtesy of David Eldridge, pers. comm., after supporting information section S4, in Eldridge et al. (2020)
Attribute | Interpretation and relevance | |
---|---|---|
Surface roughness | Surface microtopography. Rougher surfaces have a greater ability to retain abiotic and biotic resources; qualitative; visual assessment. | |
Crust resistance | The ability of the soil to resist erosion; qualitative. | |
Crust brokenness | Extent to which the soil crust is broken. Broken crusts are more susceptible to erosion. Cracks may be indicative of potential microsites for seeds to settle; qualitative; visual assessment. | |
Crust stability | The degree to which surface soil aggregates maintain their stability when wetted; qualitative; assessed with the Emerson slake test (Tongway 1995). | |
Surface integrity | 100 minus the cover of erosional features (e.g. rills, scalds, pedestals); qualitative, visual assessment. | |
Deposited materials | Extent and nature of materials deposited on the surface from upslope; quantitative, visual assessment. | |
Biocrust cover | Cover of biological soil crusts, which protect the soil against erosion, fix nutrients and provide habitat for seeds and soil biota; quantitative, visual assessment. | |
Plant basal cover | Indicates examine the effect of cover on overland flow processes. Indicates stability and potential nutrient cycling; quantitative; visual assessment. | |
Litter cover | Indicates the potential for decomposition of plant material and protects the soil against erosion; quantitative; visual assessment. | |
Litter origin | Assesses if litter has been transported from elsewhere; qualitative; visual assessment. | |
Litter incorporation | The extent to which litter is incorporated into the soil; qualitative; visual assessment. |
As described in Tongway and Hindley (2004), soil surface roughness is a measure of the surface microtopography and is determined by noting the difference between the lowest and highest points of the soil surface. Soil surface roughness defines the ability of the soil to capture and retain resources such as water and organic matter.
Crust resistance is a measure of the ability of the soil to resist erosion and is measured on dry soil. It involves assessing the ease with which the soil can be disturbed, producing material suitable for erosion by wind or water. Crust brokenness is a measure of the extent to which the surface crust is broken. A more detached crust is likely to indicate material prone to movement by erosion, but can also relate to the provision of potential microsites for the collection of seeds. Crust stability, which relates to the stability of soil fragments, is measured using the Emerson Slake Test.
Surface integrity is the cover of uneroded surface, i.e. 100 minus the cover of wind or water erosion, assessed by measuring features such as rills, water sheeting, scalds, terracettes and pedestals. Deposited materials is material that is deposited by wind or water erosion and derived from elsewhere.
Cover of biocrusts provides a useful measure of surface stability because of the tendency of cryptogams to stabilise surface soils. Plant basal cover is the percentage of perennial vegetation cover that extends into the soil. This indicator assesses the belowground contribution of the vegetation to nutrient-cycling and water-infiltration processes.
The cover of litter was assessed, as well as its origin, whether it is derived from local plants or transported from elsewhere, and its degree of incorporation into the surface, i.e. how well the litter and soil are mixed together, and the proportion of litter that was derived from groundstorey plants.
These 11 attributes are used to derive three soil-surface condition (SSC) indices: (1) surface stability, (2) nutrient cycling, and (3) infiltration.
An index of surface stability was derived for each quadrat assessed as the sum of the six scores for crust resistance, crust stability, cover of biocrusts, cover of erosion, cover of deposited material, and litter cover expressed as a percentage of 36, the maximum possible score.
A score for the nutrient-cycling index, based on the ability of soil to cycle and retain nutrients, was derived by combining four surface attributes (surface roughness, biocrust cover, basal plant cover, and a combined litter index derived from the product of its origin, cover and degree of incorporation). These four surface attributes were summed and divided by the maximum score.
An index of infiltration, that is, how well the soil surface accepts water, uses the sum of values for surface roughness, crust resistance, crust stability, basal cover and the product of litter cover–origin–incorporation. This sum is divided by 57, the maximum score.
These three soil-surface condition indices have been used widely to assess different landscapes worldwide and have been confirmed to be related to laboratory and field measurements of their related processes (e.g. Eldridge et al. 2020).