Adaptive multi-paddock grazing management reduces diet quality of yearling cattle in shortgrass steppe
Tamarah R. Jorns A , J. Derek Scasta B , Justin D. Derner
A
B
C
D
E
F
G
Abstract
Adaptive multi-paddock (AMP) grazing is hypothesised to improve livestock diet quality by allowing managers to move livestock among paddocks in a manner that tracks phenological variation in forage growth related to variation in plant community composition. We compared yearling steer (Bos taurus) dietary crude protein (CP), digestible organic matter (DOM), and diet composition on a ranch-scale (2600 ha, 10 pairs of 130 ha paddocks) experiment in shortgrass steppe for 6 years (2014–2019) in contrasting grazing treatments: Collaborative Adaptive Rangeland Management (CARM, a type of AMP with a stakeholder group making science-informed management decisions within the grazing season) and season-long, continuous grazing (TRM, traditional rangeland management). These grazing treatments had the same system-level stocking rate annually but differed in stocking density, with CARM being 10-fold higher than TRM because of all steers in the CARM treatment being in a single herd. Mean grazing-season dietary CP was consistently 13–28% higher in TRM than CARM; in contrast, DOM in TRM was similar to CARM or only slightly higher (2.5%). Differences in diet quality between CARM and TRM grazing strategies were largest early in the grazing season, with CP being 14–36% higher for TRM compared to CARM steers during the first 4 weeks; these diet-quality differences between treatments disappeared towards the end of the grazing season. Implementing AMP on the basis of a diverse stakeholder group’s experiential knowledge about plant communities, soils, and ecological sites did alter within-grazing season plant functional group contributions to steer diets. Although we implemented adaptive decision-making within the grazing season, which allowed CARM steers to track the seasonal phenology of C3 versus C4 grasses as steers rotated among paddocks varying in plant community composition, these adaptive movements did not offset the detrimental effects on diet quality associated with grazing in a herd with 10-fold higher stocking density. Managers applying AMP in semiarid rangelands should be cognisant that high stocking density can be associated with lower diet quality early in the growing season, which can compromise livestock gain responses.
Keywords: adaptive multi-paddock grazing, collaborative adaptive rangeland management, crude protein, digestible organic matter, DNA metabarcoding, semiarid rangeland, stocker cattle, stocking density.
Introduction
A key tenet of adaptive multi-paddock (AMP) grazing management is explicitly incorporating management decisions on when and how to rotate livestock among paddocks to facilitate varable-length grazing and recovery periods. This is typically achieved by rotating grazing animals, at a higher stocking density, through multiple paddocks during the grazing season compared to season-long continuous grazing (i.e. non-rotational). Presumed benefits of AMP include improving vegetation and soil biota, and enhancing soil chemical, physical, and hydrological properties for ecosystem functioning (Teague et al. 2011; Park et al. 2017). Proponents of AMP also argue that season-long grazing can lead to land degradation through non-uniform livestock distribution and regrazing of preferred individual plants without sufficient recovery times (Teague et al. 2013, but see Porensky et al. 2021). Stocking density (defined as the number of grazing animals per unit area) is typically several-fold higher within a paddock in AMP than with season-long grazing where animals have longer grazing periods within a paddock. At the timescale of a grazing season, increased stocking density combined with longer rest intervals between periods when a paddock is grazed can affect the quality of the diet consumed by grazing animals through two mechanisms. First, animals grazing at increased stock density may forage less selectively and have reduced diet quality because of greater intra-herd competition and the faster rate at which forage available is depleted (e.g. McCollum and Gillen 1998; Hall et al. 2016). Second, long rest periods may allow maturation of forage in paddocks prior to the time that it is grazed (Denny et al. 1977).
Owing to limitations of spatial scale and lack of land manager or rancher involvement, prior experimental studies of AMP grazing effects on diet quality have not accounted for adaptive decisions regarding movement of livestock among paddocks that vary in soils, plant communities, and forage phenology. Landscape features such as topography, distance to water, and forage quantity/quality influence grazing behaviour, diet selection, and grazing activity of livestock (Bailey et al. 1996; Bailey and Brown 2011; Gersie et al. 2019; Raynor et al. 2021a; Augustine et al. 2023). Thus, adaptive decision-making within the grazing season concerning the timing and sequence of livestock rotations among paddocks depends on ranchers matching animal nutritional demands with a myriad of factors, including forage phenology, composition, quantity, and quality in near-real time (Brunson and Burritt 2009). Scientific research on grazing management has largely avoided incorporating inter- and intra-annual adaptive decision-making by ranchers and land managers to enhance control in experimental designs (Briske et al. 2011a, 2011b; Hawkins 2017). Adaptive decisions within the grazing season regarding when and where livestock graze individual paddocks can affect plant communities and the provision of multiple ecosystem services (O’Reagain and Turner 1992; Augustine et al. 2020; Derner et al. 2021). Incorporating experiential knowledge about soils, plant communities, livestock behaviour, and local conditions through a collaborative management–science partnership with direct stakeholder involvement could hypothetically minimise or eliminate the negative effects of high stocking density and long rest periods on diet quality, and thereby minimise the loss in individual animal weight gains under AMP grazing with high stocking density (Derner et al. 2021). At the same time, the degree to which adaptive management within the grazing season can mitigate reductions in cattle diet quality in AMP grazing with high stocking density remains unclear, especially in semiarid rangelands.
Adaptive decision-making within the grazing season is also important for matching animal demand with spatial and temporal variability in forage supply. This is particularly difficult in low-productivity, semiarid rangelands such as the shortgrass steppe of the North American western Great Plains. Here, high spatial and temporal variability in growing-season precipitation at the ranch scale (Augustine 2010) is further compounded by plant communities differing in relative abundance of dominant C3 [western wheatgrass (Pascopyrum smithii (Rydb.) Á Löve) and needle-and-thread (Hesperostipa comata (Trin. & Rupr.) Barkworth)] and C4 [blue grama (Bouteloua gracilis (Willd. Ex Kunth) Lag. Ex Griffiths)] forage grasses. These C3 and C4 grasses differ substantially in terms of within grazing season temporal dynamics of crude protein (CP) and digestible organic matter (DOM), and potential biomass per bite (Milchunas et al. 2005; Scasta 2017; Raynor et al. 2021b). Incorporating knowledge of the spatiotemporal variation in C3 and C4 grasses in these plant communities influences diet quality and livestock weight gains (Reppert 1960; Reynolds et al. 2019), which provides potential benefits of AMP grazing (Derner et al. 2021).
Few studies have examined the seasonality of diet quality of cattle managed with AMP grazing. Insights into how adaptive decision-making affects diet quality of free-ranging livestock could be provided by measuring how plant species composition in the diet changes seasonally and in response to management decisions. In contrast to diet quality, plant composition of the diet is more difficult to measure in extensive rangeland because of the laborious nature of techniques such as microhistology, bite counts, and fistulation that have been employed in prior work (Van Dyne and Torell 1964; Sanders et al. 1980; Genin et al. 1994; Shrestha and Wegge 2006; Kowalczyk et al. 2011). DNA metabarcoding technology has been used to quantify diet composition and selectivity from faecal samples encompassing multiple herbivorous species (Quéméré et al. 2013, Soininen et al. 2013, Kartzinel et al. 2015, Scasta et al. 2020). Rather than true diet composition, DNA metabarcoding provides the percentage of protein derived from each species in a faecal sample (Craine et al. 2016; Garnick et al. 2018). Scasta et al. (2019) noted limitations in the DNA metabarcoding technique with a feeding trial, and proposed modifications to increase the utility of this technique for determining diet composition of free-ranging animals on extensive rangelands.
We examined the degree to which adaptive within-season decision-making associated with cattle rotations employing AMP grazing influenced seasonal diet quality and diet composition of cattle compared with non-adaptive, non-rotational grazing. We hypothesised that the higher stock density with AMP grazing would increase inter-animal competition for forage and result in reduced diet quality, with differences more pronounced early in the grazing season. This behaviour was expected because steers in AMP grazing would have less selectivity for nutritious forage and therefore need to ingest low-quality, prior-year standing biomass for gut fill. Conversely, steers that are not rotated would express more selectivity for highly palatable forages due to the low stock density. We expected diet quality of steers in the different grazing treatments to converge in the late stages of the grazing season because prior-year standing biomass is mostly senesced by early summer forage-quality declines with advancing plant phenology, and highly variable precipitation (Augustine 2010) limits plant regrowth.
Materials and methods
Study site and design
This experiment was conducted at the United States Department of Agriculture–Agricultural Research Service (USDA-ARS) Central Plains Experimental Range, a Long-Term Agroecosystem Research (LTAR, https://ltar.ars.usda.gov/) network site located in north-central Colorado, USA (40°50′N, 104°43′W). Long-term mean annual precipitation for this site is 340 mm, with precipitation predominately occurring from April to September (Augustine et al. 2014). Mean temperature is −2.5°C in winter and 21.7°C in summer (PRISM Climate Group and Oregon State University 2023). Key forage graminoids are the perennial warm-season (C4) grass species blue grama and the perennial cool-season (C3) graminoids, western wheatgrass and needle-and-thread. The most prevalent forb is scarlet globemallow (Sphaeralcea coccinea (Nutt.) Rydb.). Topography is flat to gently rolling. To characterise heterogeneity in soils and plant communities within and among paddocks, we used ecological site maps derived from the US national soil survey (SSURGO, https://www.nrcs.usda.gov/resources/data-and-reports/soil-survey-geographic-database-ssurgo), where an ecological site represents a distinctive kind of land with specific climate and soil processes and properties that determine the land’s ability to support certain kinds and amounts of vegetation (Duniway et al. 2010). Study paddocks encompassed three types of ecological sites, namely, loamy plains, sandy plains, and salt flats (USDA 2007a, 2007b, 2007c). The loamy plains ecological site is dominated by the C4 shortgrass blue grama (USDA 2007a), and is the most prevalent but least productive ecological site. The sandy plains ecological site is characterised by increased co-dominance by the C3 midgrasses western wheatgrass and needle-and-thread, and scattered shrubs (e.g. four-wing saltbush, Atriplex canescens (Pursh) Nutt.; USDA-NRCS 2007b), and is moderately prevalent and productive. Finally, the salt flats ecological site is characterised by the dominance of C4 saltgrasses, including alkali sacaton (Sporobolus airoides (Torr.) Torr.) and inland saltgrass (Distichlis spicata (L.) Greene), and is the least prevalent but most productive ecological site (USDA 2007c) at the Central Plains Experimental Range.
Experimental design
Comprehensive experimental design details can be found in Wilmer et al. (2018) and Augustine et al. (2020). Grazing treatments were as follows: (1) Collaborative Adaptive Rangeland Management (CARM), where a science-management partnership implemented data- and monitoring-informed decision-making using AMP grazing during the grazing season through a participatory, multi-stakeholder approach, and (2) traditional rangeland management (TRM; continuous, season-long grazing, Bement 1969). A diverse 11-member stakeholder group, composed of four local ranchers, four public land management agencies, and three non-government conservation organisations, developed shared goals and objectives regarding vegetation productivity, structure, and composition, livestock performance and drought resilience, grassland bird habitat, and social learning (Wilmer et al. 2018, 2022). The stakeholder group was responsible for inter- and intra-annual adaptive decisions regarding stocking rates, grazing sequences of paddocks, triggers for livestock movement among paddocks, and incorporation of prescribed burns (Wilmer et al. 2018). During the grazing season, weekly updates were provided to stakeholder group, including data on forage quantity within the grazed pasture, soil moisture, animal behaviour, precipitation, and diet quality.
Twenty 130-ha paddocks were grouped into 10 pairs. Paddock pairs varied in the degree to which they were dominated by loamy plains, sandy plains and salt flat ecological sites (USDA 2007a, 2007b, 2007c; Augustine et al. 2020). Three pairs of paddocks were dominated by loamy plains, three by sandy plains, and the remaining four pairs contained a mixture of multiple different ecological sites and were classified as mixed. Grazing treatments were randomly applied to paddocks within each pair. Yearling steers, weighing approximately 270 kg (0.6 animal unit equivalent, AUE) at the beginning of the grazing season in mid-May, grazed both treatments until early October in each of 6 years (2014–2019). This is a typical grazing-season length in the shortgrass steppe (Derner et al. 2021), with yearlings then being placed in a feedlot for the finishing phase on grain diets prior to harvest.
Yearling steers in both treatments were stocked at the same stocking rate (number of steers for the 1300 ha treatment area, for the same grazing-season length) annually, but stocking density (number of steers per a given grazed paddock) was 10-fold higher each year in the CARM than TRM treatment because of the stakeholder group decision to have a single herd for the CARM treatment (Wilmer et al. 2018; Augustine et al. 2020). Stocking rates were determined annually by the stakeholder group prior to the grazing season, and numbers of yearling steers per treatment were 214, 224, 234, 244, 280, and 244 in 2014–2019 respectively. In the CARM treatment, the stakeholder group managed the cattle as one large herd adaptively moved among paddocks on the basis of forage quantity and composition, livestock behaviour, and day limit triggers, typically resulting in movements every 14–28 days (Augustine et al. 2020). Two of the ten 130-ha paddocks in CARM were selected each year for rest (non-use) prior to the grazing season by the stakeholder group, with the objective of enhancing C3 perennial grass abundance in rested paddocks, creating grassbanks for future use in the event of a drought, and creating tall-structure vegetation for grassland birds of conservation concern (Davis et al. 2020, 2021). On the basis of the described criteria set for rotations combined with weekly measurements of available forage and livestock behaviour, the CARM herd grazed eight paddocks in 2019, seven paddocks in 2014 and 2016, and four paddocks in 2015 owing to above-average forage production (Augustine et al. 2020). With below-average precipitation and forage availability in 2017 and 2018, the CARM herd grazed nine paddocks. Rested paddocks were considered part of the overall CARM grazing system and were not available for alternative uses. The 10 TRM paddocks were each grazed season-long by one small herd, one-tenth the number of the CARM herd. In 2015, 2017, and 2018, animals had access to 32-ha of patch burns in one (2017 and 2018) or two (2015) CARM paddocks, and in the paired TRM paddocks. Patch burns were conducted the previous fall to control plains pricklypear (Opuntia polycantha Haw) abundance within the pastures and increase forage quality. The stakeholder group typically chose to graze CARM paddocks with patch burns at the beginning of the grazing season to capitalise on increased forage quality of vegetation (Augustine et al. 2010; Augustine and Derner 2014) in 25% of the paddocks.
Diet quality
To measure cattle diet quality, faecal samples were collected weekly from each grazing treatment across the 6 years. We subsampled fresh faecal pats on the same day from 10 individual steers from the CARM herd and four individual steers from the paired-paddock (i.e. same ecosite) TRM herd. Subsamples were combined in the field into one composite sample per treatment, and composite samples were then frozen and sent to the Grazingland Animal Nutrition laboratory (Texas A&M University, Temple, TX, USA) and analysed for CP and DOM by using Near Infrared Reflectance Spectroscopy (NIRS; Lyons and Stuth 1992).
Diet composition
To quantify cattle diet composition, faecal samples were collected twice monthly during the 2015–2017 grazing seasons from five (CARM) and two (TRM, again from the paired paddock) individual animals. Samples collected from multiple animals were combined into one composite sample per treatment and sampling date. Composite samples were frozen and sent to Jonah Ventures (Boulder, CO, USA) and analysed using DNA metabarcoding (Craine et al. 2015, 2016). This methodology reconstructs the diet by using DNA located in the plant chloroplast cells (Valentini et al. 2009). Individual plant species samples were concurrently collected and analysed. Clustered DNA sequences from the faecal and plant samples were assigned an exact sequence variant (ESV) identification number. Each ESV can match with multiple plant species depending on the variability in the DNA sequence; multiple ESVs may also represent the same species. When this occurred, location-specific voucher-sample DNA matches were considered most accurate and were the preferred match for an ESV. When multiple species were similar in base pair matches, or when species could not be accurately detected, the species with the highest match by base pair and the least number of misses in the sequence was selected to represent the ESV or the species were grouped at a higher level (genus or family) (Scasta et al. 2019). Overall, this method provides a means to describe diet contributions, which incorporates frequency of detections of plant groups consumed by large herbivores (Kartzinel and Pringle 2020).
Statistical analysis
RStudio (4.2.3, R Core Team 2023) was used to conduct all data analyses. We used mixed-effects models in the lmerTest package (Kuznetsova et al. 2017) to assess differences in diet quality across the grazing season for each year of the experiment. Fixed effects included treatment, day of year, and their interaction, and rotation was used as a random intercept to allow the model’s intercept to vary as the CARM herd rotated through the paddocks. The TRM treatment was set as the baseline factor. Because rotations of the CARM herd were not the same each year, resulting in paddocks being grazed at different times during the grazing season, we conducted analyses only within year and not across years. Furthermore, the CARM cattle grazed four to nine paddocks each year depending on the forage conditions (with the remainder being rested), whereas the TRM cattle always grazed all 10 paddocks available to them. Over the course of the 6 years of results reported here, the CARM cattle utilised all 10 of the available paddocks, and thus were managed at the same system-level stocking rate as for the TRM cattle. However, in any given year, the fact that the CARM herd grazed fewer than 10 paddocks means that they were managed at a higher within-year stocking rate. To address this difference, we conducted additional analyses of the difference in diet quality between the two treatments. If the CARM cattle had been rotated through all 10 paddocks, rotations would have occurred at 14-day intervals (10 paddocks over a 140-day grazing season), rather than on the basis of actual forage quantity and phenological state. Because faecal samples were collected weekly, we analysed rarified models that were based only on samples collected during the first 2 weeks (14 days) that cattle grazed in each pasture in the sequence for that year, and compared our results to the model fit by using samples collected in all weeks. This second analyses was conducted to evaluate whether the length of time CARM cattle spent in individual pastures (so as to allow others to be rested) was the primary driver of differences in diet quality.
For diet composition analyses, data were grouped at the lowest consistent taxonomic grouping available (functional group) by using the top 70 ESVs representing means across all composite samples. Functional groups were C3 annual grasses (C3AG), C3 perennial grasses (C3PG), C4 perennial grasses (C4PG), digestible forbs, sedges, and shrubs. Indigestible plants or unknown in cases when ESVs could not be adequately distinguished (Scasta et al. 2019) constituted 3.8% of the diet on average and were excluded from further analyses. Digestible forbs and indigestible plants were determined using scientific and experiential expert knowledge. Diet composition data were then relativised by functional group and averaged by month of grazing season for each grazing treatment. Variation in diet composition was analysed using a principal-component analysis in the FactoMineR package (Lê et al. 2008).
Results
Crude protein
Mean grazing-season CP in diets of yearling steers was consistently 13–28% higher each year in the TRM versus the CARM treatment (Table 1). Interactions between grazing treatment and day of year occurred in 4 of the 6 years (P < 0.05, exceptions were 2014 and 2019). Day of year was a significant predictor of dietary CP (P < 0.05) in all years, with dietary CP declining as the growing season progressed and treatments converging towards the end of the grazing season (Fig. 1). During the first 4 weeks of the grazing season, dietary CP was consistently 14–36% greater for TRM than for CARM steers. In rarified models assessing differences in dietary CP between treatments for samples collected during the first 2 weeks CARM animals occupied each pasture, treatment was again a significant predictor across all years (P < 0.05, data not shown).
Year | Day of year | Treatment | Treatment × Day of year | ||||
---|---|---|---|---|---|---|---|
Dietary crude protein content (%) | |||||||
2014 | F = 20.25 | P < 0.001* | F = 4.24 | P < 0.05* | F = 1.94 | P = 0.175 | |
2015 | F = 53.62 | P < 0.001* | F = 17.39 | P < 0.001* | F = 7.61 | P < 0.001* | |
2016 | F = 68.55 | P < 0.001* | F = 55.10 | P < 0.05* | F = 36.42 | P < 0.001* | |
2017 | F = 33.97 | P < 0.001* | F = 12.84 | P < 0.01* | F = 6.26 | P < 0.05* | |
2018 | F = 97.01 | P < 0.001* | F = 9.24 | P < 0.001* | F = 5.72 | P < 0.05* | |
2019 | F = 23.29 | P < 0.05* | F = 5.97 | P < 0.05* | F = 3.34 | P = 0.80 | |
Dietary digestible organic matter content (%) | |||||||
2014 | F = 46.88 | P < 0.001* | F = 0.02 | P = 0.90 | F = 0.10 | P = 0.75 | |
2015 | F = 25.71 | P = 0.08 | F = 0.04 | P = 0.84 | F = 0.45 | P = 0.51 | |
2016 | F = 9.49 | P < 0.05* | F = 6.47 | P < 0.05* | F = 3.61 | P = 0.07 | |
2017 | F = 88.84 | P < 0.001* | F = 8.51 | P < 0.05* | F = 9.06 | P < 0.01* | |
2018 | F = 10.50 | P < 0.05* | F = 4.70 | P < 0.05* | F = 2.62 | P = 0.12 | |
2019 | F = 14.50 | P < 0.01* | F = 11.81 | P < 0.005* | F = 9.51 | P < 0.05* |
Asterisks indicate significant effects (P < 0.05).
Dietary crude protein (%) and digestible organic matter (%) across the grazing season for yearling steers in CARM (circles and solid line) and TRM (triangles and dashed line). Within each year, changes in the intercept of the fitted regression line indicate dates when cattle rotated into a new block of the experiment. Background shading also changes when animals in the CARM treatment were rotated into a new pasture. Symbols enclosed with a red box indicate weeks when cattle were grazing within an experimental block where paddocks in both treatments contained a patch burn.
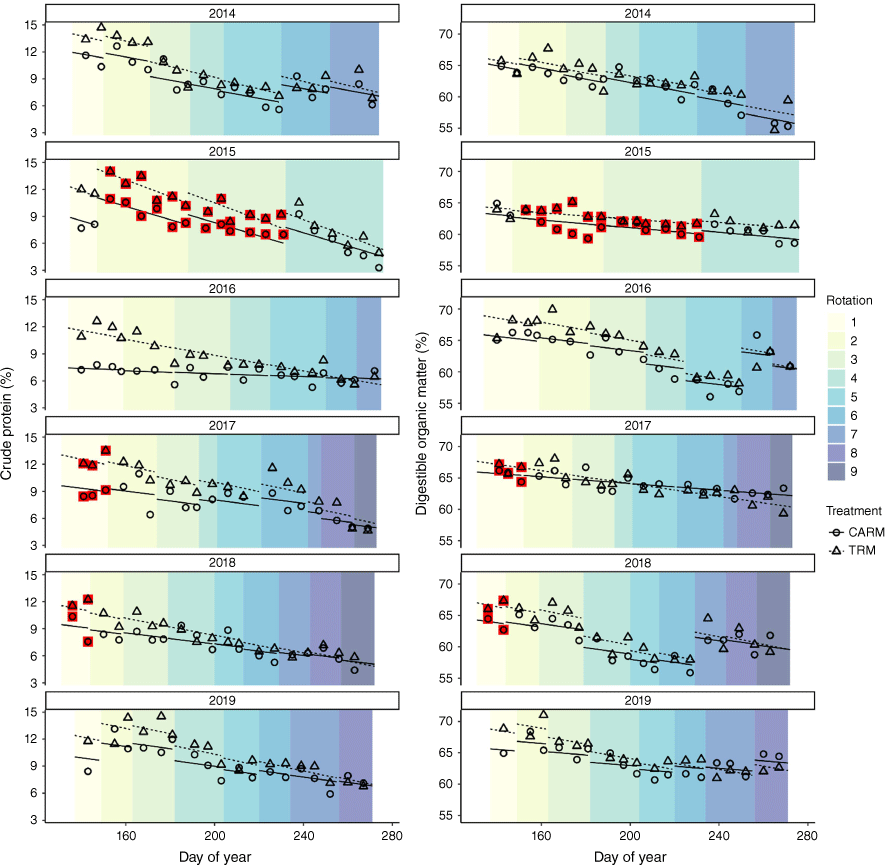
Dietary CP increased in 2015 for CARM steers rotated into a paddock with patch burns present, and also for the steers in the TRM pasture pair also with a patch burn (Fig. 2). Dietary CP values were >12% in years when steers started the grazing season in a paddock pair with patch burns (2017 and 2018; Fig. 1).
Plant functional-group diet composition (%, box and whisker plots, mean ± 1 s.e.) for the 2015–2017 grazing seasons for yearling steers in CARM and TRM. Functional groups are cool-season annual grasses (C3AG), cool-season perennial grasses (C3PG), warm-season grasses (C4PG), digestible forbs, sedges, and shrubs. Asterisks indicate significant differences between treatments within a year.
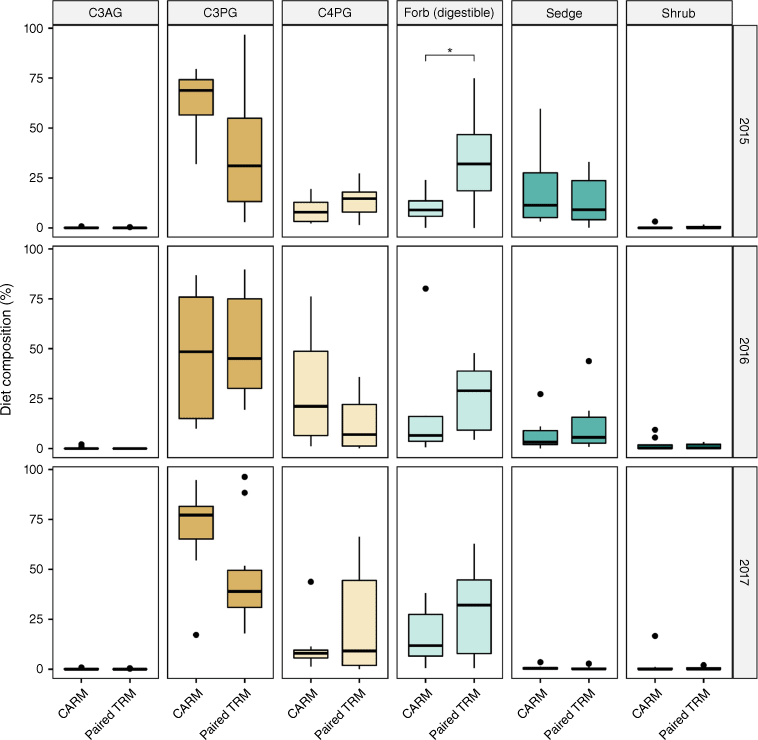
Digestible organic matter
Mean grazing season DOM in diets of yearling steers was 2.3–2.6% higher in the TRM treatment than in CARM in 4 of the 6 years (2016–2019; Table 1; P < 0.05). Interactions between grazing treatment and day of year occurred in only 2 years (2017 and 2019; P < 0.05). Day of year was a significant predictor of DOM (P < 0.05) in all years except 2015, with DOM declining as the growing season progressed and treatments converging at the end of the grazing season (Fig. 1). During the first 4 weeks of the grazing season, dietary DOM was 2.3–4.5% greater for TRM steers than their counterparts in CARM in the 4 years when treatments differed significantly (2016–2019).
Diet composition
For the three grazing seasons when we measured diet composition (2015–2017), CARM and TRM steers in the paired paddock did not differ in plant functional-group contributions to their diets (Fig. 2; P > 0.05), except in 2015 when the TRM steers consumed significantly more digestible forbs than did the CARM steers (P < 0.05), and CARM steers consumed more C3 perennial graminoids (Fig. 2; P < 0.05). In 2016, contributions of plant functional groups to steer diets in both treatments were generally similar, although CARM steers showed a trend of increased C4 grass consumption in the middle of 2016. The opposite trend was observed in 2017, with more C4 grass consumption by TRM cattle (Fig. 2). The high proportion of digestible forbs in the diet of TRM steers in 2015 occurred during the second half of the grazing season, and hence was not correlated with the timing of the large difference in dietary CP between treatments in the first half of the grazing season. Furthermore, the varying trends in contribution of C4 grasses to TRM versus CARM diets in 2016 and 2017 (primarily in the middle of the grazing season) also did not correspond to timing of the largest differences in dietary CP (Fig. 3 vs Fig. 2). We also note that shrubs and C3AG were largely absent from diets of both CARM and TRM steers for all months during these three grazing seasons, and that sedges contributed significantly to diet composition in the latter part of 2015 and 2016, but were absent from diets in 2017.
Functional-group diet composition (%, mean) by month of grazing season in 2015–2017 for yearling steers in CARM (top panel) and TRM, (bottom panel). Functional groups are cool-season annual grasses (C3AG), cool-season perennial grasses (C3PG), warm-season grasses (C4PG), digestible forbs, sedges, and shrubs. Shading indicates rotations between pastures in the CARM treatment and the paired TRM herd to that pasture rotation.
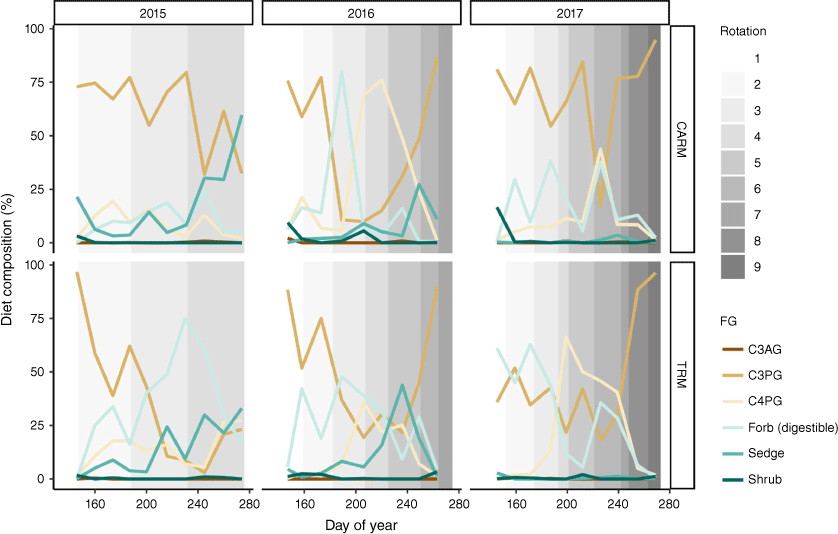
Principal-component analysis Axis 1 explained 34.1% of the variation in functional-group diet composition, with C3PG (42.3%) loading strongly and positively on Axis 1 (Fig. 4). Axis 2 explained 19.5% of the variation in the dataset, with C4PG (46.0%) loading strongly and positively on Axis 2. Digestible forbs and, to a lesser degree, sedges were associated with low scores on both axes. TRM steers had lower scores on both PCA Axes (Fig. 4) compared to CARM steers, indicating that averaged over the 3 years, TRM steer diets tended to contain more sedges and forbs than did CARM steer diets.
Principal-component analysis of functional-group diet composition (%) in 2015–2017 for CARM (black) and paired TRM (red). Ellipses were created at a 95% confidence level around the mean. Axis 1 explains 34.1% and Axis 2 explains an additional 19.5% of the variation in the dataset. Cool-season perennial grasses (C3PG) were most useful in explaining Axis 1 and warm-season grasses (C4PG) were most useful in explaining Axis 2.
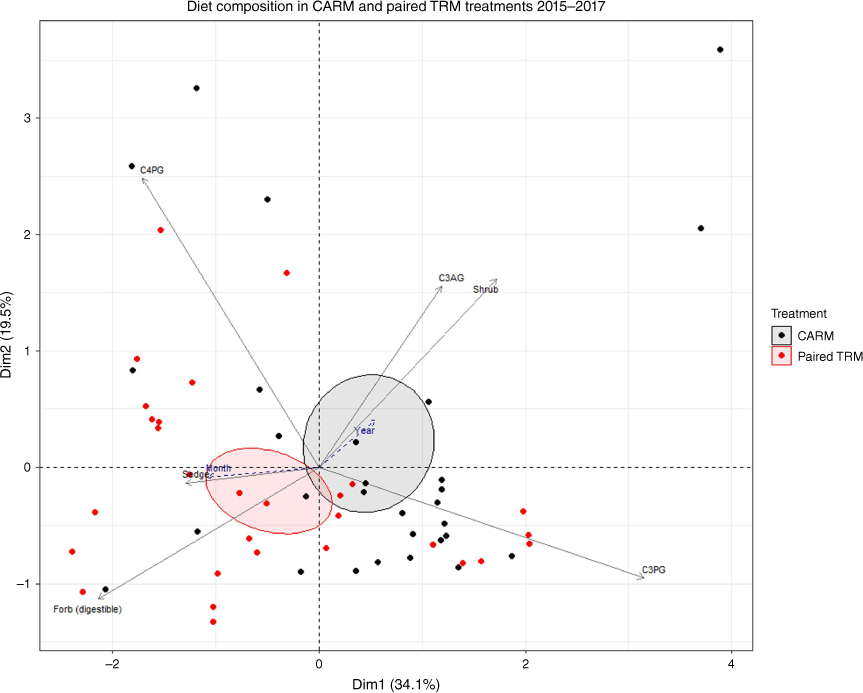
Discussion
A goal of adaptive multi-paddock (AMP) grazing is utilising the experiential and real-time knowledge of ranchers and land managers about plant communities, ecological sites, and plant phenology, to make decisions regarding the rotation of livestock among paddocks to match animal demand more effectively with forage availability and quality. This ranch-scale (2600 ha) experiment in the North American shortgrass steppe, a semiarid rangeland ecosystem, demonstrated that application of collaboratively designed AMP grazing via the CARM grazing treatment suppressed the amount of dietary crude protein (CP) in yearling-steer diets by 13–28% for all 6 years (2014–2019) compared with the TRM (season-long continuous grazing) treatment. These findings were consistent across years varying widely in precipitation, forage availability, and the rate of cattle rotation among paddocks. Similar results were reported by McCollum et al. (1994), who found a 15% reduction in dietary CP for yearling steers managed with time-controlled rotational grazing versus season-long grazing in tallgrass prairie. Although the contribution of different plant functional groups to cattle diets varied widely both within and across years, we did not detect any clear differences in functional group composition between the two grazing treatments that could explain the difference in dietary CP during the first half of the grazing season each year. Rather, cattle foraging-behaviour data indicated that the reduction in diet quality was associated with CARM steers foraging in more linear pathways and moving across shorter distances in each foraging bout, than did TRM steers (Augustine et al. 2023), because the 10-fold higher stock density increased inter-animal competition (Popp et al. 1997; Rind and Phillips 1999; Gusha et al. 2017). In contrast, TRM cattle foraged in pathways with greater tortuosity and moved over a longer distance during each foraging bout (Augustine et al. 2023), which suggests that they more selectively consume bites with less standing dead vegetation and more green leaves. More selective grazing by TRM cattle was also consistent with the overall trend of increased consumption of digestible forbs (Figs 2, 4). However, on the basis of differences in timing (Fig. 2), we conclude that the primary driver of increased early season dietary CP for TRM steers is likely to be reduced consumption of standing dead vegetation. Furthermore, we note that treatment effects on dietary CP remained significant even for models based only on samples collected during the first 2 weeks of each rotation, indicating that these differences were not simply driven by the length of the CARM rotations. Treatment effects on dietary DOM were more inconsistent but occurred in the latter half of the experiment when rainfall and forage production levels were lower, and the stakeholder group rotated the CARM steers through pastures more quickly. Although we implemented adaptive decision-making within the grazing season, which allowed CARM cattle to track the seasonal phenology of C3 versus C4 grasses as well as spatial and temporal variation in forage availability, these adaptive movements did not offset the dietary consequences of foraging within a herd with the much higher stocking density.
Our finding of reductions in seasonal diet quality of yearling steers as the growing season progressed is consistent with Jung et al. (1985) and Walker et al. (1989) in shortgrass steppe and Cline et al. (2009) in mixed-grass prairie, with differences observed here more pronounced for dietary CP than for DOM. The consistent diet-quality differences between cattle managed with AMP in CARM versus TRM are the most likely explanation for reduced livestock weight gain in CARM (reductions of 11–16% versus TRM; Augustine et al. 2020). Collectively, these results demonstrated that in semiarid rangelands, the complexity of semiarid environments make it difficult to manage in an adaptive manner within a grazing season for livestock production. This may not be necessarily true of temperate environments, with vegetation composition including improved forages with high growth rates (Rowntree et al. 2020; Bork et al. 2021).
Differences in diet quality of yearling steers between CARM and TRM grazing strategies were magnified early in the grazing season, with diet quality converging to similarly low values in both treatments towards the end of the grazing season. Substantial differences in diet quality (14–36% higher dietary CP values for TRM than for CARM steers) occurred during the first month of the grazing season when individual-animal daily gains are typically the highest in shortgrass steppe (Hyder et al. 1975). Adaptive management included incorporating fall patch burns in one-quarter (25%, 32.5 ha) of the 130-ha paddock for CARM and TRM paddock pairs in 2014, 2016, and 2017, and burning was associated with higher dietary CP in yearling-steer diets in both treatments for most sampling weeks when these paddocks were grazed early in the following grazing season (2015, 2017, and 2018, see boxes around data points in Fig. 1). Dietary CP of CARM steers increased most notably when they were rotated from an unburned paddock to a patch-burned paddock between weeks 2 and 3 in 2015. This finding is consistent with prior studies showing that prescribed burns in shortgrass steppe can enhance forage quality early in the growing season (Augustine et al. 2010).
C4 perennial grasses comprise most of the forage biomass in this ecosystem (e.g. Milchunas et al. 2005; Augustine et al. 2020), but this plant functional group was under-represented in diet composition (Figs 2, 3). Prior work at this site concurs with our findings because blue grama proportion in yearling faeces was from 21% (Shoop et al. 1985) to 38% (Vavra et al. 1977) during the summer grazing season. Scasta et al. (2019) indicated that the faecal DNA metabarcoding method used here does not detect blue grama as well as other species, particularly if blue grama represents a low physical proportion of ingested plant material. As a result, we interpret our diet composition results as indicating a similar composition between the two grazing treatments, but likely underestimating the true absolute contribution to the diet. The high representation of C3 perennial graminoids in diet composition early and late in the growing season is consistent with previous studies as well (Hansen and Gold 1977; Shoop et al. 1985). Our findings are also consistent with prior work indicating that forbs and shrubs comprise 20% and 5% of the yearling diet respectively (Shoop et al. 1985).
Implications
Grazing management decisions are unique to each ranching operation because local conditions, resources, enterprise goals, and management expertise differ (Roche et al. 2015). Recommendations for improving grazing management on semiarid rangelands have often emphasised altering timing of grazing within and across grazing seasons to promote plant community health and resilience. This approach has resulted in a promulgation of rotational grazing and particular emphasis on AMP grazing to increase flexibility in where, when, and how long livestock are grazing, while also providing more capacity for managers to assess forage availability and quality relative to animal demand and growth requirements (Teague and Barnes 2017). AMP strategies typically apply a greater stocking density within grazed paddocks. Our study combined AMP with a diverse stakeholder group’s experiential knowledge about plant communities, soils, and ecological sites. This provided the opportunity for within grazing-season adaptive management to alter plant functional group contributions to steer diets during the grazing season. Despite documented advantages over non-adaptive rotational systems (Derner et al. 2021), these efforts were unable to overcome the consequences of 10-fold higher stocking density on diet quality, with resultant reductions in cattle weight gain (Augustine et al. 2020). Managers employing AMP should therefore be cognisant of potential tradeoffs between livestock performance, which we found to be negatively affected by AMP, and the provision of other ecosystem services. For example, AMP can create a more heterogeneous vegetation structure that enhances habitat for grassland birds of conservation concern (Derner et al. 2009; Davis et al. 2020, 2021), and social cohesion, learning, and networking are benefited (Hawkins et al. 2022; Wilmer et al. 2022). Management science partnerships are especially important when managing complex problems in environments where high spatiotemporal variation necessitates creative and innovative solutions for provision of multiple ecosystem services from rangelands (Boyd and Svejcar 2009).
Acknowledgements
We thank the Crow Valley Livestock Cooperative Inc. for providing the cattle. We thank Matt Mortenson, Pam Freeman, Jeff Thomas, Jake Thomas, Craig Lawrence, Melissa Johnston, Averi Reynolds, and many other summer technicians for sampling and technical support. The authors also thank Seth Romero for his invaluable help on the fDNA bioinformatic analysis. The USDA–ARS, Plains Area, is an equal opportunity/affirmative action employer, and all agency services are available without discrimination. Any use of trade, firm, or product names is for descriptive purposes only and does not imply endorsement by the US Government. This research was a contribution from the Long-Term Agroecosystem Research (LTAR) network. LTAR is supported by the United States Department of Agriculture.
References
Augustine DJ (2010) Spatial versus temporal variation in precipitation in a semiarid ecosystem. Landscape Ecology 25, 913-925.
| Crossref | Google Scholar |
Augustine DJ, Derner JD (2014) Controls over the strength and timing of fire–grazer interactions in a semi-arid rangeland. Journal of Applied Ecology 51, 242-250.
| Crossref | Google Scholar |
Augustine DJ, Derner JD, Milchunas DG (2010) Prescribed fire, grazing, and herbaceous plant production in shortgrass steppe. 63, 317-323.
| Crossref | Google Scholar |
Augustine DJ, Brewer P, Blumenthal DM, Derner JD, Von Fischer JC (2014) Prescribed fire, soil inorganic nitrogen dynamics, and plant responses in a semiarid grassland. Journal of Arid Environments 104, 59-66.
| Crossref | Google Scholar |
Augustine DJ, Derner JD, Fernández-Giménez ME, Porensky LM, Wilmer H, Briske DD (2020) Adaptive, multipaddock rotational grazing management: a ranch-scale assessment of effects on vegetation and livestock performance in semiarid rangeland. Rangeland Ecology and Management 73, 796-810.
| Crossref | Google Scholar |
Augustine DJ, Kearney S, Raynor EJ, Porensky LM, Derner JD (2023) Adaptive, multi-paddock, rotational grazing management alters foraging behavior and spatial grazing distribution of free-ranging cattle. Agriculture, Ecosystems & Environment 352, 108521.
| Crossref | Google Scholar |
Bailey DW, Brown JR (2011) Rotational grazing systems and livestock grazing behavior in shrub-dominated semi-arid and arid rangelands. Rangeland Ecology and Management 64, 1-9.
| Crossref | Google Scholar |
Bailey DW, Gross JE, Laca EA, Rittenhouse LR, Coughenour MB, Swift DM, Sims PL (1996) Mechanisms that result in large herbivore grazing distribution patterns. Journal of Rangeland Management 49, 386-400.
| Crossref | Google Scholar |
Bement RE (1969) A stocking-rate guide for beef production on blue-grama range. Journal of Rangeland Management 22, 83-86.
| Crossref | Google Scholar |
Bork EW, Döbert TF, Grenke JSJ, Carlyle CN, Cahill Jr JF, Boyce MS (2021) Comparative pasture management on Canadian cattle ranches with and without adaptive multipaddock grazing. Rangeland Ecology and Management 78, 5-14.
| Crossref | Google Scholar |
Boyd CS, Svejcar TJ (2009) Managing complex problems in rangeland ecosystems. Rangeland Ecology and Management 62, 491-499.
| Crossref | Google Scholar |
Briske DD, Sayre NF, Huntsinger L, Fernández-Giménez ME, Budd B, Derner JD (2011b) Origin, persistence, and resolution of the rotational grazing debate: integrating human dimensions into rangeland research. Rangeland Ecology and Management 64, 325-334.
| Crossref | Google Scholar |
Brunson MW, Burritt EA (2009) Behavioral factors in rotational grazing systems. Rangelands 31, 20-25.
| Crossref | Google Scholar |
Cline HJ, Neville BW, Lardy GP, Caton JS (2009) Influence of advancing season on dietary composition, intake, site of digestion, and microbial efficiency in beef steers grazing a native range in western North Dakota. Journal of Animal Science 87, 375-383.
| Crossref | Google Scholar | PubMed |
Craine JM, Towne EG, Miller M, Fierer N (2015) Climatic warming and the future of bison as grazers. Scientific Reports 5, 16738.
| Crossref | Google Scholar | PubMed |
Craine JM, Angerer JP, Elmore A, Fierer N (2016) Continental-scale patterns reveal potential for warming-induced shifts in cattle diet. PLoS One 11, e0161511.
| Crossref | Google Scholar | PubMed |
Davis KP, Augustine DJ, Monroe AP, Derner JD, Aldridge CL (2020) Adaptive rangeland management benefits grassland birds utilizing opposing vegetation structure in the shortgrass steppe. Ecological Applications 30, e02020.
| Crossref | Google Scholar | PubMed |
Davis KP, Augustine DJ, Monroe AP, Aldridge CL (2021) Vegetation characteristics and precipitation jointly influence grassland bird abundance beyond the effects of grazing management. Ornithological Applications 123, 1-15.
| Crossref | Google Scholar |
Denny RP, Barnes DL, Kennan TCD (1977) Trials of multi paddock grazing systems on veld. 1. An explanatory trial of systems involving twelve paddocks and one herd. Rhodesian Journal of Agricultural Research 15, 11-16.
| Google Scholar |
Derner JD, Lauenroth WK, Stapp P, Augustine DJ (2009) Livestock as ecosystem engineers for grassland bird habitat in the western Great Plains of North America. Rangeland Ecology and Management 62, 111-118.
| Crossref | Google Scholar |
Derner JD, Augustine DJ, Briske DD, Wilmer H, Porensky LM, Fernández-Giménez ME, Peck DE, Ritten JP, the CARM Stakeholder Group (2021) Can collaborative adaptive management improve cattle production in multipaddock grazing systems?. Rangeland Ecology and Management 75, 1-8.
| Crossref | Google Scholar |
Duniway MC, Bestelmeyer BT, Tugel A (2010) Soil processes and properties that distinguish ecological sites and states. Rangelands 32, 9-15.
| Crossref | Google Scholar |
Garnick S, Barboza PS, Walker JW (2018) Assessment of animal-based methods used for estimating and monitoring rangeland herbivore diet composition. Rangeland Ecology and Management 71, 449-457.
| Crossref | Google Scholar |
Genin D, Villca Z, Abasto P (1994) Diet selection and utilization by llama and sheep in a high altitude arid rangeland of Bolivia. Journal of Rangeland Management 47, 245-248.
| Crossref | Google Scholar |
Gersie SP, Augustine DJ, Derner JD (2019) Cattle grazing distribution in shortgrass steppe: influences of topography and saline soils. Rangeland Ecology and Management 72, 602-614.
| Crossref | Google Scholar |
Gusha J, Masocha M, Mugabe PH (2017) Impact of grazing system on rangeland condition and grazing capacity in Zimbabwe. The Rangeland Journal 39, 219-225.
| Crossref | Google Scholar |
Hall TJ, McIvor JG, Jones P, Smith DR, Mayer DG (2016) Comparison of stocking methods for beef production in northern Australia: seasonal diet quality and composition. The Rangeland Journal 38, 553-567.
| Crossref | Google Scholar |
Hansen RM, Gold IK (1977) Blacktail prairie dogs, desert cottontails and cattle trophic relations on shortgrass range. Journal of Range Management 30, 210-214.
| Crossref | Google Scholar |
Hawkins H (2017) A global assessment of Holistic Planned GrazingTM compared with season-long, continuous grazing: meta-analysis findings. African Journal of Range & Forage Science 34, 65-75.
| Crossref | Google Scholar |
Hawkins HJ, Venter ZS, Cramer MD (2022) A holistic view of holistic management: what do farm-scale, carbon, and social studies tell us? Agriculture, Ecosystems, & Environment 323, 107702.
| Crossref | Google Scholar |
Jung HG, Rice RW, Koong LJ (1985) Comparison of heifer weight gains and forage quality for continuous and short-duration grazing systems. Journal of Rangeland Management 38, 144-148.
| Crossref | Google Scholar |
Kartzinel TR, Pringle RM (2020) Multiple dimensions of dietary diversity in large mammalian herbivores. Journal of Animal Ecology 89, 1482-1496.
| Crossref | Google Scholar | PubMed |
Kartzinel TR, Chen PA, Coverdale TC, Erickson DL, Kress WJ, Kuzmina ML, Rubenstein DI, Wang W, Pringle RM (2015) DNA metabarcoding illuminates dietary niche partitioning by African large herbivores. Proceedings of the National Academy of Sciences of the United States of America 112, 8019-8024.
| Crossref | Google Scholar | PubMed |
Kowalczyk R, Taberlet P, Coissac E, Valentini A, Miquel C, Kamiński T, Wójcik JM (2011) Influence of management practices on large herbivore diet: case of European bison in Bialowieża Primeal Forest (Poland). Forest Ecology and Management 261, 821-828.
| Crossref | Google Scholar |
Kuznetsova A, Brockhoff PB, Christensen RHB (2017) lmerTest package: tests in linear mixed effects models. Journal of Statistical Software 82, 1-26.
| Crossref | Google Scholar |
Lê S, Josse J, Husson F (2008) FactoMineR: an r package for multivariate analysis. Journal of Statistical Software 25, 1-18.
| Crossref | Google Scholar |
Lyons RK, Stuth JW (1992) Fecal NIRS equations for predicting diet quality of free-ranging cattle. Journal of Rangeland Management 45, 238-244.
| Crossref | Google Scholar |
McCollum FT, III, Gillen RL (1998) Grazing management affects nutrient intake by steers grazing tallgrass prairie. Journal of Rangeland Management 51, 69-72.
| Crossref | Google Scholar |
McCollum FT, III, Gillen RL, Brummer JE (1994) Cattle diet quality under short duration grazing on tallgrass prairie. Journal of Rangeland Management 47, 489-493.
| Crossref | Google Scholar |
Milchunas DG, Mosier AR, Morgan JA, LeCain DR, King JY, Nelson JA (2005) Elevated CO2 and defoliation effects on a shortgrass steppe: forage quality versus quantity for ruminants. Agriculture, Ecosystems & Environment 111, 166-184.
| Crossref | Google Scholar |
O’Reagain PJ, Turner JR (1992) An evaluation of the empirical basis for grazing management recommendations for rangeland in southern. Journal of the Grassland Society of Southern Africa 9, 38-49.
| Crossref | Google Scholar |
Park J, Ale S, Teague WR, Jeong J (2017) Evaluating the ranch and watershed scale impacts of using traditional and adaptive multi-paddock grazing on runoff, sediment and nutrient losses in North Texas, USA. Agriculture, Ecosystems & Environment 24, 32-44.
| Crossref | Google Scholar |
Popp JD, McCaughey WP, Cohen RDH (1997) Grazing system and stocking rate effects on the productivity, botanical composition and soil surface characteristics of alfalfa-grass pastures. Canadian Journal of Animal Science 77, 669-676.
| Crossref | Google Scholar |
Porensky LM, Augustine DJ, Derner JD, Wilmer H, Lipke MN, Fernandez-Gimenez ME, Briske DD, CARM Stakeholder Group (2021) Collaborative adaptive rangeland management, multipaddock rotational grazing, and the story of the regrazed grass plant. Rangeland Ecology & Management 78, 127-141.
| Crossref | Google Scholar |
PRISM Climate Group Oregon State University (2023) Available at https://prism.oregonstate.edu/explorer/ [accessed 25 September 2023]
Quéméré E, Hibert F, Miquel C, Lhuillier E, Rasolondraibe E, Champeau J, Rabarivola C, Nusbaumer L, Chatelain C, Gautier L, Ranirison P, Crouau-Roy B, Taberlet P, Chikhi L (2013) A DNA metabarcoding study of a primate dietary diversity and plasticity across its entire fragmented range. PLoS One 8, e58971.
| Crossref | Google Scholar | PubMed |
Raynor EJ, Derner JD, Soder KJ, Augustine DJ (2021b) Noseband sensor validation and behavioural indicators for assessing beef cattle grazing on extensive paddocks. Applied Animal Behavior Science 242, 105402.
| Crossref | Google Scholar |
Raynor EJ, Gersie SP, Stephenson MB, Clark PE, Spiegal SA, Boughton RK, Bailey DW, Cibils A, Smith BW, Derner JD, Estell RE, Nielson RM, Augustine DJ (2021a) Cattle grazing distribution patterns related to topography across diverse rangeland ecosystems of North America. Rangeland Ecology and Management 75, 91-103.
| Crossref | Google Scholar |
R Core Team (2023) ‘R: A Language and Environment for Statistical Computing.’ (R Foundation for Statistical Computing, Vienna, Austria) Available at https://www.R-project.org/ [accessed 1 May 2023]
Reppert JN (1960) Forage preference and grazing habits of cattle at the eastern Colorado range station. Journal of Rangeland Management 13, 58-65.
| Crossref | Google Scholar |
Reynolds AQ, Derner JD, Augustine DJ, Porensky LM, Wilmer H, Jorns T, Briske DD, Scasta JD, Fernández-Giménez ME (2019) Ecological sites: can they be managed to promote livestock production. Rangelands 41, 239-243.
| Crossref | Google Scholar |
Rind MI, Phillips CJC (1999) The effects of group size on the ingestive and social behaviour of grazing dairy cows. Animal Science 68, 589-596.
| Crossref | Google Scholar |
Roche LM, Cutts BB, Derner JD, Lubell MN, Tate KW (2015) On-ranch grazing strategies: context for the rotational grazing dilemma. Rangeland Ecology and Management 68, 248-256.
| Crossref | Google Scholar |
Rowntree JE, Stanley PL, Maciel ICF, Thorbecke M, Rosenzweig ST, Hancock DW, Guzman A, Raven MR (2020) Ecosystem impacts and productive capacity of multi-species pastured livestock system. Frontiers in Sustainable Food Systems 4, 232.
| Crossref | Google Scholar |
Sanders KD, Dahl BE, Scott G (1980) Bite-count vs fecal analysis for range animal diets. Journal of Range Management 33, 146-149.
| Crossref | Google Scholar |
Scasta J (2017) Seasonal forage dynamics of three grasses with different origins and photosynthetic pathways in a rural North American cold steppe. Livestock Research for Rural Development 29, 1-15.
| Google Scholar |
Scasta JD, Jorns T, Derner JD, Lake S, Augustine DJ, Windh JL, Smith TL (2019) Validation of DNA metabarcoding of fecal samples using cattle fed known rations. Animal Feed Science Technology 255, 114219.
| Crossref | Google Scholar |
Scasta JD, Jorns T, Derner JD, Stam B, McClaren M, Calkins C, Stewart W (2020) Technical note: toxic plants in sheep diets grazing extensive landscapes: insights from fecal DNA metabarcoding. Livestock Science 236, 104002.
| Crossref | Google Scholar |
Shoop MC, Clark RC, Laycock WA, Hansen RM (1985) Cattle diets on shortgrass ranges with different amounts of fourwing saltbush. Journal of Range Management 38, 443-449.
| Crossref | Google Scholar |
Shrestha R, Wegge P (2006) Determining the composition of herbivore diets in the trans-Himalayan rangelands: a comparison of field methods. Rangeland Ecology and Management 59, 512-518.
| Crossref | Google Scholar |
Soininen EM, Ravolainen VT, Bråthen KA, Yoccoz NG, Gielly L, Ims RA (2013) Arctic small rodents have diverse diets and flexible food selection. PLOS ONE 8, e68128.
| Crossref | Google Scholar | PubMed |
SSURGO (2022) Available at https://www.nrcs.usda.gov/resources/data-and-reports/description-of-gridded-soil-survey-geographic-gssurgo-database [accessed 25 October 2022]
Teague R, Barnes M (2017) Grazing management the regenerates ecosystem function and grazingland livelihoods. African Journal of Range & Forage Science 34, 77-86.
| Crossref | Google Scholar |
Teague R, Dowhower SL, Baker SA, Haile N, DeLaune PB, Conover DM (2011) Grazing management impacts on vegetation, soil biota and soil chemical, physical and hydrological properties in tall grass prairie. Agriculture, Ecosystems, & Environment 141, 310-322.
| Crossref | Google Scholar |
Teague R, Provenza F, Kreuter U, Steffens T, Barnes M (2013) Multi-paddock grazing on rangelands: why the perceptual dichotomy between research results and rancher experience. Journal of Environmental Management 128, 699-717.
| Crossref | Google Scholar | PubMed |
USDA (2007a) Ecological site description for Loamy Plains (R067BY002CO). Available at https://edit.jornada.nmsu.edu/catalogs/esd/067B/R067BY002CO [accessed 25 October 2022]
USDA (2007b) Ecological site description for Sandy Plains (R067BY024CO). Available at https://edit.jornada.nmsu.edu/catalogs/esd/067B/R067BY024CO [accessed 25 October 2022]
USDA (2007c) Ecological site description for Salt Flat (R067BY033CO). Available at https://edit.jornada.nmsu.edu/catalogs/esd/067B/R067BY033CO [accessed 25 October 2022]
Valentini A, Pompanon F, Taberlet P (2009) DNA barcoding for ecologists. Trends in Ecology & Evolution 24, 110-117.
| Crossref | Google Scholar | PubMed |
Van Dyne GMV, Torell DT (1964) Development and use of the esophageal fistula: a review. Journal of Rangeland Management 17, 7-19.
| Crossref | Google Scholar |
Vavra M, Rice RW, Hansen RM, Sims PL (1977) Food habits of cattle on shortgrass range in northcentral Colorado. Journal of Range Management 30, 261-263.
| Crossref | Google Scholar |
Walker JW, Heitschmidt RK, Moraes EAD, Kothmann MM, Dowhower SL (1989) Quality and botanical composition of cattle diets under rotational and continuous grazing treatments. Journal of Rangeland Management 42, 239-242.
| Crossref | Google Scholar |
Wilmer H, Derner JD, Fernández-Giménez ME, Briske DD, Augustine DJ, Porensky LM (2018) Collaborative adaptive rangeland management fosters management–science partnerships. Rangeland Ecology and Management 71, 646-657.
| Crossref | Google Scholar |
Wilmer H, Schulz T, Fernández-Giménez ME, Derner JD, Porensky LM, Augustine DJ, Ritten J, Dwyer A, Meade R (2022) Social learning lessons from collaborative adaptive rangeland management. Rangelands 44, 316-326.
| Crossref | Google Scholar |