Could metabolic imaging and artificial intelligence provide a novel path to non-invasive aneuploidy assessments? A certain clinical need
Fabrizzio Horta
A
B
C
D
E
F
Abstract
Pre-implantation genetic testing for aneuploidy (PGT-A) via embryo biopsy helps in embryo selection by assessing embryo ploidy. However, clinical practice needs to consider the invasive nature of embryo biopsy, potential mosaicism, and inaccurate representation of the entire embryo. This creates a significant clinical need for improved diagnostic practices that do not harm embryos or raise treatment costs. Consequently, there has been an increasing focus on developing non-invasive technologies to enhance embryo selection. Such innovations include non-invasive PGT-A, artificial intelligence (AI) algorithms, and non-invasive metabolic imaging. The latter measures cellular metabolism through autofluorescence of metabolic cofactors. Notably, hyperspectral microscopy and fluorescence lifetime imaging microscopy (FLIM) have revealed unique metabolic activity signatures in aneuploid embryos and human fibroblasts. These methods have demonstrated high accuracy in distinguishing between euploid and aneuploid embryos. Thus, this review discusses the clinical challenges associated with PGT-A and emphasizes the need for novel solutions such as metabolic imaging. Additionally, it explores how aneuploidy affects cell behaviour and metabolism, offering an opinion perspective on future research directions in this field of research.
Keywords: aneuploidy, artificial intelligence, embryo quality, infertility, IVF, label free imaging, metabolic imaging, PGT-A.
Introduction
Pre-implantation genetic testing for aneuploidy (PGT-A) by embryo biopsy aids embryo selection by determining the ploidy of the embryo. Although some studies suggest higher pregnancy rates with PGT-A (Scott et al. 2013), especially in older patients (Capalbo et al. 2017), several factors need consideration. These include the invasive nature of the embryo biopsy procedure, variations in PGT-A success rates among clinics and providers (Popovic et al. 2024), interpretation challenges such as copy number variants, mosaicism (Capalbo et al. 2017) and/or inaccurate representation of the whole embryo (Capalbo et al. 2017), treatment delays due to waiting for genetic results (Rothwell et al. 2020), high cost and significant additional time, skill, equipment and labour in IVF laboratories to accommodate these procedures (Veiga et al. 2022). Hence, there is significant clinical need to improve embryo diagnostic practices, including new procedures that do not harm embryos or increase treatment costs. Particularly considering that the accuracy of PGT-A testing is still undetermined and remains controversial after so many years of implementation (Practice Committee and Genetic Counseling Professional Group (GCPG) of the American Society for Reproductive Medicine 2020; Practice Committees of the American Society for Reproductive Medicine and the Society for Assisted Reproductive Technology 2024).
Considering this, there have been increasing efforts to develop non-invasive technologies that can aid and improve the current practice of embryo selection, including non-invasive PGT-A (Rubio et al. 2020) and application of artificial intelligence (AI) algorithms (Salih et al. 2023). So far, a non-invasive procedure to assess embryo ploidy has not been validated for routine clinical use, so current practice remains reliant on embryo biopsy. Non-invasive metabolic imaging that measures cellular metabolism via autofluorescence of metabolic cofactors (Blacker and Duchen 2016) has recently been studied in reproductive science and reproductive medicine to investigate oocytes and preimplantation embryos (Shah et al. 2020, 2022; Tan et al. 2022; Sakkas et al. 2024). Distinct metabolic activity signatures have been found in aneuploid embryos and human fibroblasts using hyperspectral microscopy and machine learning algorithms (Campbell et al. 2022; Tan et al. 2022). Similarly, fluorescence life time imaging microscopy (FLIM), another non-invasive metabolic imaging method, has successfully identified metabolic variations in mouse oocytes (Sanchez et al. 2018), embryos and human preimplantation embryos (Ma et al. 2019; Sanchez et al. 2019a, 2019b). These approaches show promise in distinguishing between euploid and aneuploid human embryos with accuracy (Shah et al. 2020, 2022; Tan et al. 2022; Sakkas et al. 2024). Following promising results in pre-clinical studies and studies using human blastocysts, further investigations into novel technologies for potential clinical use could open an opportunity to assess if this type of non-invasive technology could improve human embryo selection procedures. Considering these new developments, in this perspective we discuss; (1) the current practice of PGT-A and the need for novel solutions; (2) how aneuploidy can change cellular metabolism and behaviour; (3) current results in the application of metabolic imaging in reproductive science and AI applications and, (4) future research directions.
Current practice of PGT-A requires novel solutions
Aneuploidy is defined as a chromosomal aberration in which the copy number of chromosomes is diagnosed as altered (Nagaoka et al. 2012). Aneuploidies can be caused by meiotic errors during oocyte or sperm development or can occur sporadically as a mitotic chromosome segregation error during early embryo development (Nagaoka et al. 2012). All cells, or only a fraction of the cells in an embryo can be aneuploid, the later scenario giving rise to a mosaic aneuploid embryo (Taylor et al. 2014; Treff and Marin 2021). Embryo aneuploidy can lead to poor embryo development, miscarriages or stillbirth, except for cases with known aneuploidy conditions such as Down Syndrome that can be carried to term (Taylor et al. 2014).
Interestingly, recent studies argue that chromosomal mosaicism is a common feature in human preimplantation embryos (van Echten-Arends et al. 2011; Chavli et al. 2024). Indeed, the compelling study of Chavli et al. (2024), using single-cell whole-genome sequencing of human embryos, a powerful technology that can analyse the chromosome status of each individual cell of the entire blastocyst embryo, indicated that at least 82% of human blastocyst embryos are mosaic, with often less than 20% of cells in an embryo being aneuploid (Chavli et al. 2024). These findings demonstrate that mosaicism is a widespread and quite well tolerated cellular error event, highlighting that caution is needed around the clinical use of PGT-A. The reason is that only 5–10 cells are analysed after embryo biopsy, hence the odds of achieving accurate ploidy results with such high levels of mosaicism are low as biopsies represent less than 10% of cells of the entire embryo (Fig. 1). Moreover, current DNA bulk-sequencing methods cannot detect low levels of mosaicism in clinical practice, as aberrant DNA copy numbers cannot be detected when only 20% or fewer of the biopsied cells are aneuploid, including similar issues with reciprocal translocations that cancel each other out (Robertson and Richards 2024). This means that there has been a routinely undercounted detection of chromosomal abnormalities, classifying embryos as entirely normal when they may present aneuploid cells (Robertson and Richards 2024) (Fig. 1). Similarly, this study also demonstrated that most of these embryos (58%), exhibited mitotic origin aneuploidy with a similar distribution between the inner cell mass and trophectoderm cells. This finding again demonstrates that genetic analysis of trophectoderm cells only poorly reflects the ploidy of the human embryo proper. The fact that this contrasts with other studies (Rana et al. 2023) represents the controversial nature of PGT-A as it is currently practiced.
Pre-implantation genetic testing for aneuploidies (PGT-A) issues. The high frequency of embryo mosaicism and the number of cells normally biopsied leads to an inaccurate representation of the whole embryo, thus, interpretation challenges. Furthermore, the embryo biopsy process is invasive, potentially leading to embryo damage or detrimental effects during this additional labourious process in IVF laboratories. The intrinsic need of embryo vitrification (to wait for genetic testing results) leads to treatment delays when is not necessarily the best practice for patients.
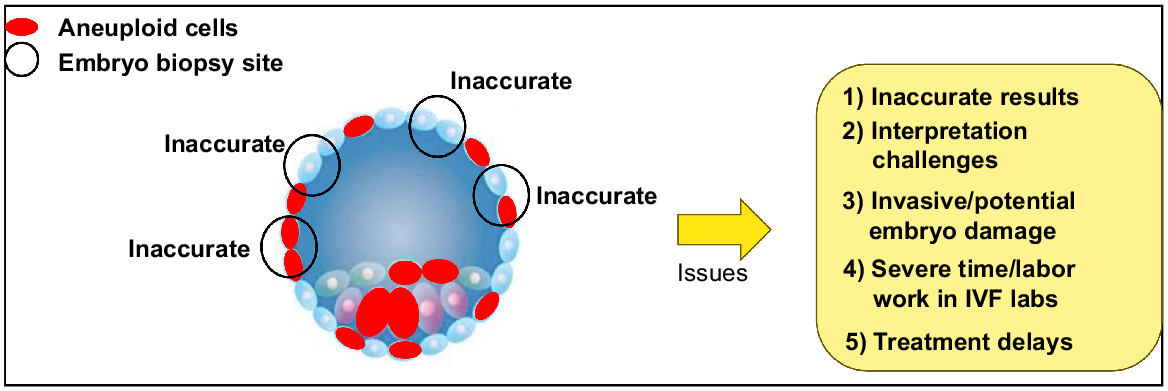
Clinical and patient perspective significance
Infertility remains one of the most devastating tragedies of modern times, afflicting up to 11% of women and 186 million individuals globally (Barbieri 2019; Bagade et al. 2022). The personal, emotional, and socioeconomic burden remains substantial (Bagade et al. 2022). However, for every assisted reproductive treatment (ART) cycle commenced, only one in four women will celebrate the birth of a newborn whilst the rest will have to deal with the unfulfilled hope and promise, forcing nearly 20% of women to abandon infertility treatment (Newman et al. 2022a). Over the past decade, only marginal improvements have been made in the live birth rate (LBR) per cycle of ART, creeping up from 22% (2010) to 28% (2019) (Newman et al. 2022a). What remains concerning globally is the increasing trend in the data demonstrating not only stagnant, but declining LBRs in some countries, prompting concerns around disparities in access to best-in-class care (Kushnir et al. 2017). In ongoing efforts to increase LBRs through ART, PGT-A has become widely used in ART procedures. This is evidenced by a significant rise in PGT-A usage, from 5% in 2013 to approximately 45% by 2020 (Kucherov et al. 2023). However, PGT-A exacerbates access disparity due to its out-of-pocket cost, averaging an additional ~USD4000 per treatment (Dunn et al. 2024). Moreover, it leads to changes in the treatment plan, such as the necessity to resort to frozen embryo transfer to allow time for the results of the PGT-A to be received, leading to longer waiting periods. Widespread use of PGT-A is also associated with reduced accessibility due to the high complexity and embryologist skill level required to perform embryo biopsy for PGT-A (Fig. 1). Thus, developing reliable non-invasive methods for assessing embryo quality that avoid the high cost and complexity of the current practice should be a key focus in ART. Improved embryo selection with avoidance of transfer of morphologically normal but non-viable aneuploid embryos would reduce the number of embryo transfers needed to achieve a viable pregnancy, reducing the time to pregnancy and easing emotional and financial burdens on patients.
Aneuploidy can change cell behaviour and metabolism
Aneuploid cells can exhibit aberrant cell function, metabolism and cell behaviour (Zhu et al. 2018; Li and Zhu 2022). Normal cell physiology depends on a balanced dosage of gene products, which is altered in aneuploid cells as the relative dosage of genes is affected by either gain or loss of chromosomes. The concept of gene dosage-effects holds that the concentration of the primary gene product is proportional to the copy number of genes (Epstein 1990). In addition to this direct effect, aneuploidy has also been linked to indirect effects on the expression of genes on other chromosomes (Rancati et al. 2008; Pavelka et al. 2010). Unbalanced gene dosage affects global gene expression leading to aneuploidy-associated cell stress. The extent of the stress can depend on the karyotype, ploidy level, cell type or tissue origin (Zhu et al. 2018; Li and Zhu 2022). For instance, using different aneuploid human cell lines, Dürrbaum et al. (2014) found a unique aneuploidy response pattern, characterised by upregulation of genes involved in diverse cellular functions, antigen processing, and metabolic pathways (Dürrbaum et al. 2014). However, in all cases, regardless of the specific karyotype, a general stress state was observed.
An unbalanced gene dosage affects cellular behaviour and metabolism (Fig. 2). It can be hypothesised that the unbalanced gene expression leads to proteotoxic stress, referring to the cellular systems that maintain optimal protein folding and homeostasis (Deshaies 2014). Structural and/or enzymatic protein complexes require a well-characterised stoichiometry for cellular function as this process is tightly regulated to allow a balanced expression of protein (Zhu et al. 2018; Li and Zhu 2022). For instance, α/β tubulin dimers require balanced protein expression to enable the specific function of their protein complex, but inadequate balance leads to cell lethality (Anders et al. 2009). Another consequence could be loss of proteostasis as aneuploid cells would exhibit an overproduction of proteins relative to the systems needed for their processing or degradation (Dai and Sampson 2016). This can result in accumulation of proteins and/or overloaded protein degradation machineries, taking them away from their normal cellular functions (Donnelly and Storchová 2015) (Fig. 2). Consequently, aneuploidy-induced metabolic stress could occur as the metabolic homeostasis depends on precise coordination of metabolic pathways and stoichiometry of enzymes and regulators that can lose their cellular function (Zhu et al. 2018; Li and Zhu 2022). It has been shown that altered metabolism in aneuploid human cell lines is reflected in alterations in glutamine use, the production of ammonium and lactate, as well as increases in glucose uptake (Williams et al. 2008). Furthermore, altered regulation of DNA, RNA, carbohydrate and mitochondrial metabolism has also been observed (Stingele et al. 2012). The observed alteration of DNA metabolic pathways is consistent with replication defects noted in aneuploid cells (Foijer et al. 2014; Leese et al. 2022). Finally, it has also been described that an increase in reactive oxygen species (ROS) as a result of increased metabolic stress (Apel and Hirt 2004) (Fig. 2). For the preimplantation embryo these imbalances due to aneuploidy may alter the necessary homeostasis needed for the embryo to function efficiently, as argued by the quiet hypothesis (Leese et al. 2022) or variations of the quiet hypothesis (Gardner and Wale 2013). Hence, as can be expected, aneuploidy leads to aberrant cellular metabolism and function, leading to a potential diagnostic opportunity based on this altered cell behaviour.
Aneuploidy can affect cell function, behaviour, and metabolism. Aneuploidy leads to an imbalanced gene dosage. This alteration changes the global gene expression of surrounding chromosomes, which leads to an imbalanced production of proteins and subsequent proteotoxic stress. The proteotoxic stress also affects enzymes critical within metabolism as well as proteins involved in regulation of protein production and degradation and increased reactive oxygen species. Similarly, proteins involved in genome stability can be affected due to this imbalance leading to overall cellular stress due to aneuploidy.
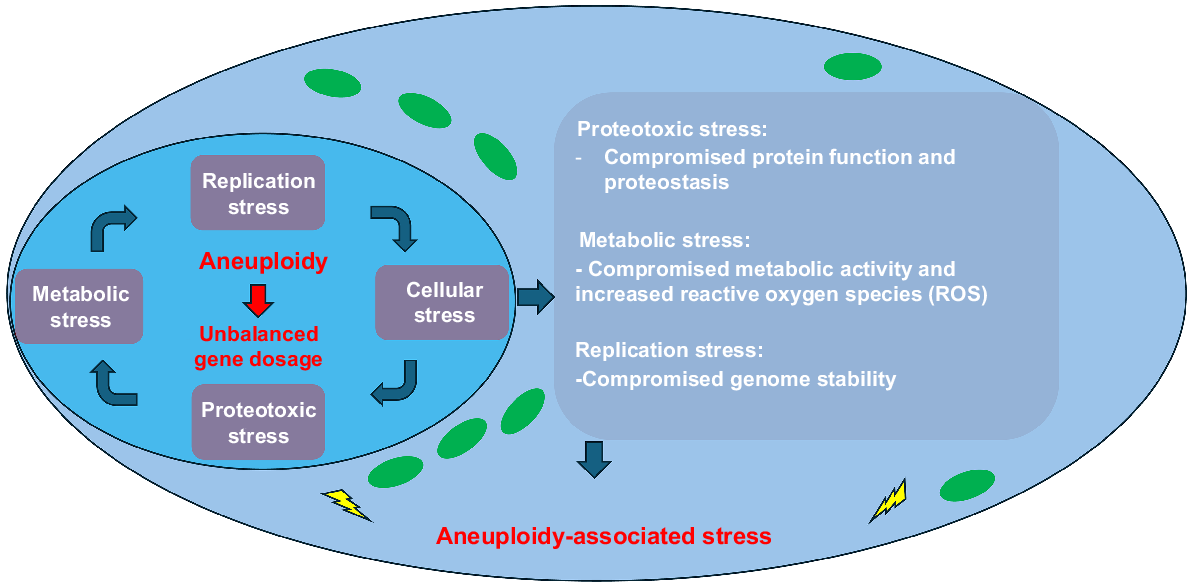
Metabolic imaging and aneuploidy detection
Nicotinamide adenine dinucleotide, NAD+, alongside the reduced forms NAD(P)H (nicotinamide adenine dinucleotide phosphate hydrogen), NADH (nicotinamide adenine dinucleotide hydrogen) as well as flavin adenine dinucleotide (FAD), play indispensable roles in metabolic pathways that are essential for energy synthesis (Xiao et al. 2018), cell signalling (Jiang et al. 2011), and DNA repair (Horta et al. 2020, 2021; Newman et al. 2022b), which are in turn associated with ageing and age-related diseases (Bonkowski and Sinclair 2016). Remarkably, NAD(P)H/NADH and FAD have auto-fluorescent properties, which have been extensively exploited as a label-free technique to monitor the intracellular redox status of living cells and tissues (Chance et al. 1979; Blacker and Duchen 2016). Because NAD(P)H and NADH have the same spectral peak at 340 nm, their light absorption qualities are identical and hence indistinguishable, and so their combined autofluorescence signal is labelled as NAD(P)H (Blacker and Duchen 2016).
Importantly, recognising the live spatial distribution of auto-fluorescent metabolic cofactors such nicotinamide adenine dinucleotide phosphate [NAD(P)H] and flavin adenine dinucleotide (FAD) (Ghukasyan and Heikal 2014) in oocytes and embryos may improve the interpretation of its relationship with conventional oocyte/embryo morphology (Sanchez et al. 2019a, 2019b) and metabolic activity at different stages of early oocyte/embryo development (Sanchez et al. 2018, 2019b). Currently, the optical redox ratio (ORR) status, which is the ratio between these two cofactors [NAD(P)H/(NAD(P)H + FAD) or FAD/(NAD(P)H + FAD)] (Chance et al. 1979; Wetzker and Reinhardt 2019), has been used as a non-invasive measurement of metabolic activity. Indeed, the ORR status has been shown to hold significant biological value when assessing metabolic activity in mammalian embryos. For instance, it has allowed the differentiation of the inner cell mass and trophectoderm cells in mice (Sanchez et al. 2018), human blastocysts (Shah et al. 2022; Sakkas et al. 2024) and detection of aneuploid cells in mice embryos and human fibroblast (Tan et al. 2022). Considering current outcomes, metabolic imaging could open new avenues to select the highest quality embryos based on their metabolic activity (Sanchez et al. 2017, 2018, 2019a, 2019b; Sutton-McDowall et al. 2017; Santos Monteiro et al. 2021; Parra et al. 2024) and/or ploidy status (Tan et al. 2022; Sakkas et al. 2024), without inducing phototoxicity or other damaging effects to the embryo as occur in PGT-A. Indeed, in the recent study of Sakkas et al. (2024), this pilot trial found 82.4% accuracy to predict aneuploidy in good quality human blastocyst that were already selected for embryo transfer (Sakkas et al. 2024). Interestingly, this study also found an altered metabolic activity in the inner cell mass of blastocyst with no differences in trophectoderm cells between euploid and aneuploid embryos. Similarly, previous work from this group (Shah et al. 2022) also found an altered metabolic activity in the inner cell mass of human blastocyst, indicating different metabolic activity profiles between both these two cell lines and ploidy status. Of surprise, these studies only included three focal planes per embryo, performing imaging analyses in a two-dimensional (2D) fashion. This suggested that with just few metabolic images it was possible to obtain high accuracy detection for aneuploidy. Hence, it could be hypothesised that increasing the number of focal planes to increase image resolution could further improve the accuracy detection as more resolution, information and three-dimensional (3D) analysis could be employed during imaging analysis and training of AI models. Considering this, the application of light sheet could be explored due to the negligible photodamaging potential properties of this type of microscopy as well as enabling high number of images and 3D capabilities (Morizet et al. 2023; Chow et al. 2024; Vargas-Ordaz et al. 2025). Nevertheless, this approach would require phototoxicity studies to ensure safety and application of light energy doses are carefully controlled and validated.
Quantitative analysis and AI can extract clinically valuable information from metabolic images
There are current concerted efforts to develop non-invasive methods to assess embryo quality and ploidy via machine learning algorithms (Jiang and Bormann 2023; Salih et al. 2023). This offers the possibility that such in-depth analysis of metabolic images could facilitate high accuracy performance to detect embryo aneuploidy with a particular focus on the inner cell mass, as recently described in mouse models (Tan et al. 2022). Indeed, the work of Tan et al. (2022) clearly demonstrated that digital image analysis and unmixing algorithms applied to spectrally defined two-dimensional auto-fluorescent images made it possible to detect aneuploid cells at high accuracy. Thus, we could speculate that future work, that includes higher spatial and spectral resolution metabolic images and/or analysis of similar images in 3D, could enhance the predictive potential of these methods (Morizet et al. 2023; Chow et al. 2024; Vargas-Ordaz et al. 2025).
In another perspective, training digital models with metabolic images could also facilitate streamlined procedures that take full advantage of recent developments in image analysis using deep AI (Vargas-Ordaz et al. 2025). Integrating deep AI models could automate the imaging analysis process through computer vision. This approach could facilitate future clinical decision support systems to assist embryologists at egg/embryo selection using metabolic information.
Future potential applications and research
Recent innovative studies have utilised imaging techniques such as FLIM and hyperspectral microscopy, however their technical complexity may create engineering challenges when translating these methodologies into clinical practice. Considering the application and development of novel advanced microscopy systems such as light-sheet systems (Vargas-Ordaz et al. 2025), there could be potential for the development of alternative non-invasive PGT-A methods using metabolic imaging and machine learning as pre-screening systems. Unlike existing approaches, light-sheet microscopy facilitates rapid results as only a few seconds of illumination are required to image an embryo, with a single plane of focus being illuminated at a time so that phototoxicity is minimised compared to standard fluorescence microscopy (Morizet et al. 2023). The small depth of focus during illumination (1.2–1.8 μm) makes it possible to obtain, between 50 and 70 focal plane images per embryo forming a 3D image. This could further enhance AI training capabilities as deep learning models perform with higher accuracies if large amount of data is available (Salih et al. 2023). Moreover, high image resolution facilitates three-dimensional analysis, potentially capable of identifying altered metabolic state in individual cell types such as the inner cell mass or trophectoderm cells. Indeed, if an altered metabolic state in individual cell types could be detected with high accuracy, this could potentially revolutionise and overcome the current controversies associated with existing PGT-A methods that can only analyse trophectoderm cells, and obviate the current challenges associated with mosaic embryos (Coll et al. 2021). This would be particularly helpful in cases of advanced reproductive age, where the total number of blastocysts produced by patients is limited, sometimes not permitting PGT-A.
Taking a step back in the process, oocyte quality and developmental potential is critical to successful fertilisation and subsequent embryo development. Currently, there is no available effective diagnostic of human oocyte quality prior to fertilisation and embryo selection. However, a decrease in NAD(P)H levels in oocytes has been associated with ageing (Bertoldo et al. 2020), which could open avenues for the introduction of new biomarkers of fertility. Thus, innovative approaches to assess oocyte viability through non-invasive metabolic imaging could also be developed to assist in improving oocyte screening for enhancing prognosis tools and patient experience. This would provide valuable information to patients with recurrent poor embryo development who may be able to accept oocyte donation if there was clear demonstration of a uniform poor oocyte quality. Additionally, this could also provide an individualised estimation of likelihood of pregnancy and healthy livebirth for women planning to cryopreserve their oocytes either to try to avoid the negative impact of female ageing on oocyte quality or before gonadotoxic chemotherapy. However, large scale prospective clinical studies are an essential step before this technology could be introduced in routine clinical practice, as validation of the application of emerging metabolic imaging technologies before their introduction is paramount.
As limitation, current metabolic imaging applications are in their initial stages of development in pre-clinical setting; thus, further safety studies are critical before large clinical studies could be conducted. In fact, the only metabolic imaging technology already employed on human embryos was FLIM (Shah et al. 2022; Sakkas et al. 2024) as safety studies on mouse embryos had already been conducted (Sanchez et al. 2018, 2019b). These paved the way for the first clinical pilot trial of this technology (Sakkas et al. 2024). Although follow up studies have not been conducted to assess the health of newborn that went through metabolic imaging, clinical pregnancies were equivalent to the standard of care in these studies (Sakkas et al. 2024).
Moreover, it is important to highlight that engineering challenges could also be faced during further technology development, particularly in the cases of using two-photon microscopy systems. The costs associated with these systems are significant, leading to potential challenges in adopting these technologies into clinical practice, as justifying their cost would require validated clinical benefits. Clinically promising metabolic imaging methods require specialised microscopy hardware, such as two-photon excitation microscopy, FLIM systems, light-sheet or a fluorescence microscopy imaging system adapted for autofluorescence imaging. Confocal microscopy, while utilised effectively in animal studies, is unlikely to be clinically useful due to high irradiance produced by its focussed lasers that can damage the embryos as compared to light-sheet microscopy (Chow et al. 2024). Clinical two-photon FLIM systems exist and have been used on human subjects, but are far from widespread, and they are expensive and not yet user-friendly (König 2020). Autofluorescence imaging at a sufficient standard requires hardware adaptations which are not yet commercially available. On top of hardware, dedicated image analysis is also required, which is currently only available in the academic sphere. As a result, clinical accessibility of metabolic imaging is, and will continue to be, limited by technical complexity and costs, unless innovative solutions could facilitate the translation of advanced microscopy into affordable ART devices for clinical and commercial use.
Conclusion
In conclusion, it is too early to anticipate that metabolic imaging coupled with AI will lead to aneuploidy testing in ART. However, the promise is certain, especially considering the high accuracy results obtained this far with methodologies that could be significantly improved with the fast development of advanced microscopy and bioengineering microsystems. Advanced imaging technologies providing a holistic view of the whole embryo and not just few cells as current embryo biopsies could open novel avenues for embryo selection. Controversially, it is quite clear that there is a significant clinical need to improve the current practice associated with PGT-A, due to several issues associated with its intrinsic invasive nature, its reliance on a biopsy to represent the whole embryo and the issues related to cut-off values of copy number, all leading to a limited analysis of embryonic cells. Thus, the ART field actively seeks innovative solutions to address current challenges so that we can advance and improve reproductive outcomes of couples world-wide.
Data availability
Data sharing is not applicable as no new data were generated or analysed during this study.
Conflicts of interest
Fabrizzio Horta has applied for a patent in the topic of metabolic imaging (PCT/AU2023/051132). The rest of authors declare no conflict of interest in this work.
References
Anders KR, Kudrna JR, Keller KE, Kinghorn B, Miller EM, Pauw D, Peck AT, Shellooe CE, Strong IJT (2009) A strategy for constructing aneuploid yeast strains by transient nondisjunction of a target chromosome. BMC Genetics 10, 36.
| Crossref | Google Scholar |
Apel K, Hirt H (2004) Reactive Oxygen Species: metabolism, oxidative stress, and signal transduction. Annual Review of Plant Biology 55(1), 373-399.
| Crossref | Google Scholar |
Bagade T, Thapaliya K, Breuer E, Kamath R, Li Z, Sullivan E, Majeed T (2022) Investigating the association between infertility and psychological distress using Australian Longitudinal Study on Women’s Health (ALSWH). Scientific Reports 12(1), 10808.
| Crossref | Google Scholar |
Bertoldo MJ, Listijono DR, Ho W-HJ, Riepsamen AH, Goss DM, Richani D, Jin XL, Mahbub S, Campbell JM, Habibalahi A, Loh W-GN, Youngson NA, Maniam J, Wong ASA, Selesniemi K, Bustamante S, Li C, Zhao Y, Marinova MB, Kim L-J, Lau L, Wu RM, Mikolaizak AS, Araki T, Le Couteur DG, Turner N, Morris MJ, Walters KA, Goldys E, O’Neill C, Gilchrist RB, Sinclair DA, Homer HA, Wu LE (2020) NAD+ repletion rescues female fertility during reproductive aging. Cell Reports 30(6), 1670-1681.e7.
| Crossref | Google Scholar |
Blacker TS, Duchen MR (2016) Investigating mitochondrial redox state using NADH and NADPH autofluorescence. Free Radical Biology and Medicine 100, 53-65.
| Crossref | Google Scholar |
Bonkowski MS, Sinclair DA (2016) Slowing ageing by design: the rise of NAD+ and sirtuin-activating compounds. Nature Reviews Molecular Cell Biology 17(11), 679-690.
| Crossref | Google Scholar |
Campbell JM, Mahbub SB, Bertoldo MJ, Habibalahi A, Goss DM, Ledger WL, Gilchrist RB, Wu LE, Goldys EM (2022) Multispectral autofluorescence characteristics of reproductive aging in old and young mouse oocytes. Biogerontology 23(2), 237-249.
| Crossref | Google Scholar |
Capalbo A, Ubaldi FM, Rienzi L, Scott R, Treff N (2017) Detecting mosaicism in trophectoderm biopsies: current challenges and future possibilities. Human Reproduction 32(3), 492-498.
| Crossref | Google Scholar |
Chance B, Schoener B, Oshino R, Itshak F, Nakase Y (1979) Oxidation-reduction ratio studies of mitochondria in freeze-trapped samples. NADH and flavoprotein fluorescence signals. Journal of Biological Chemistry 254(11), 4764-4771.
| Crossref | Google Scholar |
Chavli EA, Klaasen SJ, Van Opstal D, Laven JSE, Kops GJPL, Baart EB (2024) Single-cell DNA sequencing reveals a high incidence of chromosomal abnormalities in human blastocysts. The Journal of Clinical Investigation 134(6), e174483.
| Crossref | Google Scholar |
Chow DJX, Schartner EP, Corsetti S, Upadhya A, Morizet J, Gunn-Moore FJ, Dunning KR, Dholakia K (2024) Quantifying DNA damage following light sheet and confocal imaging of the mammalian embryo. Scientific Reports 14(1), 20760.
| Crossref | Google Scholar |
Coll L, Parriego M, Mateo S, García-Monclús S, Rodríguez I, Boada M, Coroleu B, Polyzos NP, Vidal F, Veiga A (2021) Prevalence, types and possible factors influencing mosaicism in IVF blastocysts: results from a single setting. Reproductive BioMedicine Online 42(1), 55-65.
| Crossref | Google Scholar |
Dai C, Sampson SB (2016) HSF1: guardian of proteostasis in cancer. Trends in Cell Biology 26(1), 17-28.
| Crossref | Google Scholar |
Deshaies RJ (2014) Proteotoxic crisis, the ubiquitin-proteasome system, and cancer therapy. BMC Biology 12, 94.
| Crossref | Google Scholar |
Donnelly N, Storchová Z (2015) Aneuploidy and proteotoxic stress in cancer. Molecular & Cellular Oncology 2(2), e976491.
| Crossref | Google Scholar |
Dunn A, Hunkler K, Torrealday S, Lewis T, DeCherney A, Hill M, Combs J (2024) Cost-effectiveness analysis of PGT-A in good prognosis patients. Fertility and Sterility 122(1), e25-e26.
| Crossref | Google Scholar |
Dürrbaum M, Kuznetsova AY, Passerini V, Stingele S, Stoehr G, Storchová Z (2014) Unique features of the transcriptional response to model aneuploidy in human cells. BMC Genomics 15, 139.
| Crossref | Google Scholar |
Epstein CJ (1990) The consequences of chromosome imbalance. American Journal of Medical Genetics 37(S7), 31-37.
| Crossref | Google Scholar |
Foijer F, Xie SZ, Simon JE, Bakker PL, Conte N, Davis SH, Kregel E, Jonkers J, Bradley A, Sorger PK (2014) Chromosome instability induced by Mps1 and p53 mutation generates aggressive lymphomas exhibiting aneuploidy-induced stress. Proceedings of the National Academy of Sciences 111(37), 13427-13432.
| Crossref | Google Scholar |
Gardner DK, Wale PL (2013) Analysis of metabolism to select viable human embryos for transfer. Fertility and Sterility 99(4), 1062-1072.
| Crossref | Google Scholar |
Horta F, Catt S, Ramachandran P, Vollenhoven B, Temple-Smith P (2020) Female ageing affects the DNA repair capacity of oocytes in IVF using a controlled model of sperm DNA damage in mice. Human Reproduction 35, 529-544.
| Crossref | Google Scholar |
Horta F, Ravichandran A, Catt S, Vollenhoven B, Temple-Smith P (2021) Ageing and ovarian stimulation modulate the relative levels of transcript abundance of oocyte DNA repair genes during the germinal vesicle-metaphase II transition in mice. Journal of Assisted Reproduction and Genetics 38(1), 55-69.
| Crossref | Google Scholar |
Jiang VS, Bormann CL (2023) Non-invasive genetic screening: current advances in artificial intelligence for embryo ploidy prediction. Fertility and Sterility 120, 228-234.
| Crossref | Google Scholar | PubMed |
Jiang F, Zhang Y, Dusting GJ, Sibley DR (2011) NADPH oxidase-mediated redox signaling: roles in cellular stress response, stress tolerance, and tissue repair. Pharmacological Reviews 63(1), 218-242.
| Crossref | Google Scholar |
König K (2020) Review: Clinical in vivo multiphoton FLIM tomography. Methods and Applications in Fluorescence 8(3), 034002.
| Crossref | Google Scholar |
Kucherov A, Fazzari M, Lieman H, Ball GD, Doody K, Jindal S (2023) PGT-A is associated with reduced cumulative live birth rate in first reported IVF stimulation cycles age≤ 40: an analysis of 133,494 autologous cycles reported to SART CORS. Journal of Assisted Reproduction and Genetics 40(1), 137-149.
| Crossref | Google Scholar |
Kushnir VA, Barad DH, Albertini DF, Darmon SK, Gleicher N (2017) Systematic review of worldwide trends in assisted reproductive technology 2004–2013. Reproductive Biology and Endocrinology 15, 6.
| Crossref | Google Scholar |
Leese HJ, Brison DR, Sturmey RG (2022) The quiet embryo hypothesis: 20 years on. Frontiers in Physiology 13, 899485.
| Crossref | Google Scholar |
Li R, Zhu J (2022) Effects of aneuploidy on cell behaviour and function. Nature Reviews Molecular Cell Biology 23(4), 250-265.
| Crossref | Google Scholar | PubMed |
Ma N, Mochel NRd, Pham PD, Yoo TY, Cho KWY, Digman MA (2019) Label-free assessment of pre-implantation embryo quality by the Fluorescence Lifetime Imaging Microscopy (FLIM)-phasor approach. Scientific Reports 9(1), 13206.
| Crossref | Google Scholar |
Morizet J, Chow D, Wijesinghe P, Schartner E, Dwapanyin G, Dubost N, Bruce GD, Anckaert E, Dunning K, Dholakia K (2023) UVA hyperspectral light-sheet microscopy for volumetric metabolic imaging: application to preimplantation embryo development. ACS Photonics 10(12), 4177-4187.
| Crossref | Google Scholar | PubMed |
Nagaoka SI, Hassold TJ, Hunt PA (2012) Human aneuploidy: mechanisms and new insights into an age-old problem. Nature Reviews Genetics 13(7), 493-504.
| Crossref | Google Scholar | PubMed |
Newman H, Catt S, Vining B, Vollenhoven B, Horta F (2022b) DNA repair and response to sperm DNA damage in oocytes and embryos, and the potential consequences in ART: a systematic review. Molecular Human Reproduction 28(1), gaab071.
| Crossref | Google Scholar |
Parra A, Denkova D, Burgos-Artizzu XP, Aroca E, Casals M, Godeau A, Ares M, Ferrer-Vaquer A, Massafret O, Oliver-Vila I, Mestres E (2024) METAPHOR: Metabolic evaluation through phasor-based hyperspectral imaging and organelle recognition for mouse blastocysts and oocytes. Proceedings of the National Academy of Sciences 121(28), e2315043121.
| Crossref | Google Scholar |
Pavelka N, Rancati G, Zhu J, Bradford WD, Saraf A, Florens L, Sanderson BW, Hattem GL, Li R (2010) Aneuploidy confers quantitative proteome changes and phenotypic variation in budding yeast. Nature 468(7321), 321-325.
| Crossref | Google Scholar |
Popovic M, Borot L, Lorenzon AR, Lopes ALRdC, Sakkas D, Lledó B, Morales R, Ortiz JA, Polyzos NP, Parriego M (2024) Implicit bias in diagnosing mosaicism amongst preimplantation genetic testing providers: results from a multicenter study of 36 395 blastocysts. Human Reproduction 39(1), 258-274.
| Crossref | Google Scholar |
Practice Committee and Genetic Counseling Professional Group (GCPG) of the American Society for Reproductive Medicine (2020) Clinical management of mosaic results from preimplantation genetic testing for aneuploidy (PGT-A) of blastocysts: a committee opinion. Fertility and Sterility 114(2), 246-254.
| Crossref | Google Scholar |
Practice Committees of the American Society for Reproductive Medicine and the Society for Assisted Reproductive Technology (2024) The use of preimplantation genetic testing for aneuploidy: a committee opinion. Fertility and Sterility 122, 421-434.
| Crossref | Google Scholar |
Rana B, Lambrese K, Mendola R, Xu J, Garrisi J, Miller K, Marin D, Treff NR (2023) Identifying parental and cell-division origins of aneuploidy in the human blastocyst. The American Journal of Human Genetics 110(4), 565-574.
| Crossref | Google Scholar |
Rancati G, Pavelka N, Fleharty B, Noll A, Trimble R, Walton K, Perera A, Staehling-Hampton K, Seidel CW, Li R (2008) Aneuploidy underlies rapid adaptive evolution of yeast cells deprived of a conserved cytokinesis motor. Cell 135(5), 879-893.
| Crossref | Google Scholar |
Robertson SA, Richards RI (2024) Single-cell sequencing shows mosaic aneuploidy in most human embryos. The Journal of Clinical Investigation 134(6), e179134.
| Crossref | Google Scholar |
Rothwell E, Lamb B, Johnson E, Gurtcheff S, Riches N, Fagan M, Sabatello M, Johnstone E (2020) Patient perspectives and experiences with in vitro fertilization and genetic testing options. Therapeutic Advances in Reproductive Health 14, 2633494119899942.
| Crossref | Google Scholar |
Rubio C, Navarro-Sánchez L, García-Pascual CM, Ocali O, Cimadomo D, Venier W, Barroso G, Kopcow L, Bahçeci M, Kulmann MIR, López L, De la Fuente E, Navarro R, Valbuena D, Sakkas D, Rienzi L, Simón C (2020) Multicenter prospective study of concordance between embryonic cell-free DNA and trophectoderm biopsies from 1301 human blastocysts. American Journal of Obstetrics and Gynecology 223(5), 751.e1-751.e13.
| Crossref | Google Scholar |
Sakkas D, Gulliford C, Ardestani G, Ocali O, Martins M, Talasila N, Shah JS, Penzias AS, Seidler EA, Sanchez T (2024) Metabolic imaging of human embryos is predictive of ploidy status but is not associated with clinical pregnancy outcomes: a pilot trial. Human Reproduction 39(3), 516-525.
| Crossref | Google Scholar |
Salih M, Austin C, Warty RR, Tiktin C, Rolnik DL, Momeni M, Rezatofighi H, Reddy S, Smith V, Vollenhoven B, Horta F (2023) Embryo selection through artificial intelligence versus embryologists: a systematic review. Human Reproduction Open 2023(3), hoad031.
| Crossref | Google Scholar |
Sanchez T, Seidler EA, Gardner DK, Needleman D, Sakkas D (2017) Will noninvasive methods surpass invasive for assessing gametes and embryos? Fertility and Sterility 108(5), 730-737.
| Crossref | Google Scholar |
Sanchez T, Wang T, Pedro MV, Zhang M, Esencan E, Sakkas D, Needleman D, Seli E (2018) Metabolic imaging with the use of fluorescence lifetime imaging microscopy (FLIM) accurately detects mitochondrial dysfunction in mouse oocytes. Fertility and Sterility 110(7), 1387-1397.
| Crossref | Google Scholar |
Sanchez T, Venturas M, Aghvami SA, Yang X, Fraden S, Sakkas D, Needleman DJ (2019a) Combined noninvasive metabolic and spindle imaging as potential tools for embryo and oocyte assessment. Human Reproduction 34(12), 2349-2361.
| Crossref | Google Scholar |
Sanchez T, Zhang M, Needleman D, Seli E (2019b) Metabolic imaging via fluorescence lifetime imaging microscopy for egg and embryo assessment. Fertility and Sterility 111(2), 212-218.
| Crossref | Google Scholar |
Santos Monteiro CA, Chow DJX, Leal GR, Tan TCY, Reis Ferreira AM, Thompson JG, Dunning KR (2021) Optical imaging of cleavage stage bovine embryos using hyperspectral and confocal approaches reveals metabolic differences between on-time and fast-developing embryos. Theriogenology 159, 60-68.
| Crossref | Google Scholar |
Scott RT, Jr., Upham KM, Forman EJ, Hong KH, Scott KL, Taylor D, Tao X, Treff NR (2013) Blastocyst biopsy with comprehensive chromosome screening and fresh embryo transfer significantly increases in vitro fertilization implantation and delivery rates: a randomized controlled trial. Fertility and Sterility 100(3), 697-703.
| Crossref | Google Scholar |
Shah JS, Venturas M, Sanchez TH, Penzias AS, Needleman D, Sakkas D (2020) Fluorescence lifetime imaging microscopy (FLIM) detects differences in metabolic signatures between euploid and aneuploid human blastocysts. Fertility and Sterility 114(3), e76-e77.
| Crossref | Google Scholar |
Shah JS, Venturas M, Sanchez TH, Penzias AS, Needleman DJ, Sakkas D (2022) Fluorescence lifetime imaging microscopy (FLIM) detects differences in metabolic signatures between euploid and aneuploid human blastocysts. Human Reproduction 37(3), 400-410.
| Crossref | Google Scholar |
Stingele S, Stoehr G, Peplowska K, Cox J, Mann M, Storchova Z (2012) Global analysis of genome, transcriptome and proteome reveals the response to aneuploidy in human cells. Molecular Systems Biology 8(1), 608.
| Crossref | Google Scholar |
Sutton-McDowall ML, Gosnell M, Anwer AG, White M, Purdey M, Abell AD, Goldys EM, Thompson JG (2017) Hyperspectral microscopy can detect metabolic heterogeneity within bovine post-compaction embryos incubated under two oxygen concentrations (7% versus 20%). Human Reproduction 32(10), 2016-2025.
| Crossref | Google Scholar |
Tan TCY, Mahbub SB, Campbell JM, Habibalahi A, Campugan CA, Rose RD, Chow DJX, Mustafa S, Goldys EM, Dunning KR (2022) Non-invasive, label-free optical analysis to detect aneuploidy within the inner cell mass of the preimplantation embryo. Human Reproduction 37(1), 14-29.
| Crossref | Google Scholar |
Taylor TH, Gitlin SA, Patrick JL, Crain JL, Wilson JM, Griffin DK (2014) The origin, mechanisms, incidence and clinical consequences of chromosomal mosaicism in humans. Human Reproduction Update 20(4), 571-581.
| Crossref | Google Scholar |
Treff NR, Marin D (2021) The “mosaic” embryo: misconceptions and misinterpretations in preimplantation genetic testing for aneuploidy. Fertility and Sterility 116(5), 1205-1211.
| Crossref | Google Scholar |
van Echten-Arends J, Mastenbroek S, Sikkema-Raddatz B, Korevaar JC, Heineman MJ, van der Veen F, Repping S (2011) Chromosomal mosaicism in human preimplantation embryos: a systematic review. Human Reproduction Update 17(5), 620-627.
| Crossref | Google Scholar |
Vargas-Ordaz E, Newman H, Austin C, Catt S, Nosrati R, Cadarso VJ, Neild A, Horta F (2025) Novel application of metabolic imaging of early embryos using a light-sheet on-a-chip device: a proof-of-concept study. Human Reproduction 40, 41-55.
| Crossref | Google Scholar |
Veiga E, Olmedo C, Sánchez L, Fernández M, Mauri A, Ferrer E, Ortiz N (2022) Recalculating the staff required to run a modern assisted reproductive technology laboratory. Human Reproduction 37(8), 1774-1785.
| Crossref | Google Scholar |
Wetzker C, Reinhardt K (2019) Distinct metabolic profiles in Drosophila sperm and somatic tissues revealed by two-photon NAD(P)H and FAD autofluorescence lifetime imaging. Scientific Reports 9(1), 19534.
| Crossref | Google Scholar |
Williams BR, Prabhu VR, Hunter KE, Glazier CM, Whittaker CA, Housman DE, Amon A (2008) Aneuploidy affects proliferation and spontaneous immortalization in mammalian cells. Science 322(5902), 703-709.
| Crossref | Google Scholar |
Xiao W, Wang R-S, Handy DE, Loscalzo J (2018) NAD(H) and NADP(H) redox couples and cellular energy metabolism. Antioxidants & Redox Signaling 28(3), 251-272.
| Crossref | Google Scholar |
Zhu J, Tsai H-J, Gordon MR, Li R (2018) Cellular stress associated with aneuploidy. Developmental Cell 44(4), 420-431.
| Crossref | Google Scholar |