The role of the oviduct environment in embryo survival
Jennifer L. Juengel

A
B
C
D
Abstract
Declining fertility is an issue in multiple mammalian species. As the site of fertilisation and early embryo development, the oviduct plays a critical role in embryo survival, yet there is a paucity of information on how the oviduct regulates this process.
We hypothesised that differences in steroid hormone signalling and/or immune function would be observed in a model of poor embryo survival, the peripubertal ewe.
We examined expression of steroid hormones in systemic circulation, oviductal expression of oestrogen receptor α and genes important in steroid hormone signalling, and immune function in pregnant and cyclic peripubertal and adult ewes on day 3 after oestrus.
Concentrations of progesterone, but not oestradiol, were decreased in the peripubertal ewe compared to the adult ewe. Oestrogen receptor α protein expression was increased in the peripubertal ewe, but pathway analysis of gene expression revealed downregulation of the oestrogen signalling pathway compared to the adult ewe. Differential expression of several genes involved in immune function between the peripubertal and adult ewe was consistent with an unfavourable oviductal environment in the peripubertal ewe lamb. Oestradiol concentration was positively correlated with the expression of multiple genes involved in the regulation of immune function.
Differences in the immune environment of the oviduct, potentially linked to differential modulation by steroid hormones, may partially underly the poor fertilisation and early embryo survival observed in the peripubertal ewe.
A unfavourable oviductal environment may play an important role in limiting reproductive success.
Keywords: correlations, embryo survival, gene expression, immune function, oestrogen, oviduct, progesterone, sheep.
Introduction
While the oviduct is known to be essential for natural reproduction, fertilisation and normal early embryo development can occur in vitro. This indicates that the functions of the oviduct can be replaced with defined culture conditions. This has focused research attention on the quality of the oocyte as the primary driver of early embryo development, with less attention given to the potential of the oviduct environment as a key regulator of fertilisation and the health and development of the early embryo. However, only 18.6% of initiated human-assisted reproduction cycles in 2020 resulted in a live birth (Newman et al. 2022), indicating the in vitro environment is sub-optimal. In cattle in vitro embryo production only 20–40% of the presumptive zygotes develop into blastocysts with approximately 30% of the blastocysts failing to hatch (Ferre et al. 2020). Recent studies have highlighted the potential role steroid hormones and immune regulators may play in modulating the oviduct environment and, thereby, fertility.
In sheep, increased oestradiol and progesterone concentrations tended to increase the percentage of healthy embryos early in gestation (Gonzalez-Bulnes et al. 2005). Similarly, lowering oestradiol concentrations during maturation of the preovulatory follicle in sheep increased unfertilised oocytes and retarded embryos (Oussaid et al. 1999). More intense mating behaviour, linked to increased oestradiol concentrations, is associated with increased embryo survival in both sheep (Juengel et al. 2020) and cattle (Madureira et al. 2015). In mice, specific ablation of the oestrogen receptor 1 gene (Esr1) in oviductal and uterine endothelial cells induced infertility through impaired fertilisation and early embryo death (Winuthayanon et al. 2015).
Progesterone is linked with enhanced embryo survival in cattle (Mann 2001; Mann and Lamming 2001; Green et al. 2005; Robinson et al. 2008) and sheep (Parr 1992), although excessive progesterone can be detrimental to embryo health (Diskin et al. 2016). Furthermore, in sheep, ewes with naturally enhanced embryo survival were shown to have increased concentrations of progesterone, both systemically and in the uterine vein, during the first few days of either the oestrous cycle or pregnancy (O’Connell et al. 2013).
The immune system is known to play an important role in establishment and maintenance of pregnancy. It has long been known that seminal plasma/sperm illicit an immune response in the female reproductive tract, including the oviduct (Schjenken and Robertson 2015; Saint-Dizier et al. 2020). Genes normally associated with immune function are also expressed in the embryo and regulate early embryo development (Hansen 2014). Activin and follistatin, known for their key regulatory roles in fertility (Wijayarathna and de Kretser 2016) and inflammation (Phillips et al. 2009), are also linked to early embryo development and survival (Rajput et al. 2013; O’Connell et al. 2016a). Pregnancy induced by natural mating does cause changes in gene expression of the ovine oviduct/uterine endometrium from the tip of the uterus on day 5 of pregnancy, with a key pathway underlying this observed difference to non-pregnant animals related to T-cell function (Quirke et al. 2021). Furthermore, persistence of innate immune factors in the oviduct was associated with damage to embryos and embryo death in mice with ablation of Esr1 expression in oviductal and uterine endothelial cells (Winuthayanon et al. 2015). The oviduct may not be essential for fertilisation and normal embryo development given the ability to fertilise and develop embryos in vitro, thereby bypassing the oviduct. However, changes in the oviductal environment could either enhance or suppress fertilisation and early embryo development.
We hypothesised that differences in steroid hormone signalling and/or immune function would be observed in a model of poor embryo survival. To test this hypothesis, we examined expression of steroid hormones in systemic circulation, oviductal expression of oestrogen receptor α (ERα) and expression of genes important in steroid hormone signalling and immune function in pregnant and cyclic peripubertal and adult ewes on day 3 after oestrus expression.
The peripubertal ewe was chosen as a model of poor embryo survival as they are known to have approximately twice the embryo loss when compared to adult ewes (Edwards et al. 2016). Studies following multiple ovulation and embryo transfer also highlighted that the poor fertility of the peripubertal ewe was driven by oocyte and/or oviductal quality (Quirke and Hanrahan 1977, 1983). In naturally cycling and mated peripubertal ewes, a majority of this loss occurred during the first 3 days of pregnancy, with fertilisation failure and embryo degradation during the very early stages of development being key components of this loss (Juengel et al. 2023). In contrast to this, while a majority of embryo/fetal loss occurs during the first 2 weeks of pregnancy in adult ewes, loss between days 4 and 14 was approximately twice that observed prior to day 4 (O’Connell et al. 2016b). This highlights that embryo loss may be driven by potentially different pathways in peripubertal ewes compared with adult ewes, with differences in oviductal function potentially underlying the reduced fertilisation and early embryo survival observed in peripubertal ewes.
Materials and methods
Experimental design
The experiment was approved by the Invermay Animal Ethics Committee (application number 13979) and was in accordance with the New Zealand Animal Welfare regulations. Animals used in the experiment were crossbreds (dominant breeds Romney, Coopworth and Texel). Peripubertal ewes were approximately 8 months of age, which is the average age of puberty (Edwards and Juengel 2017), and adult ewes were 3.5–5.5 years of age. The animals underwent standard farm management with supplementation with iodine and vaccinations against infectious diseases as described (Juengel et al. 2023), with the adults only receiving booster immunisations as required. Animals were run as a single group, with the animals exposed to three vasectomised rams initially for collection of samples from non-pregnant ewes, and then three fertile rams replaced the vasectomised rams for collection of samples from pregnant ewes. Given the lower reproductive rate of peripubertal ewes, some peripubertal ewes were joined with the fertile rams from the beginning. This allowed some tissues from the pregnant and non-pregnant animals to be collected during the same time period, and all tissues were collected within a 2-week period. All animals were kept on similar pastures throughout the trial. Weight of each animal was measured prior to introduction of the rams. All rams were fitted with a mating harness with crayon and ewes were observed for mating marks each morning. The first morning a ewe was observed with a mating mark was considered day 1 as mating would have occurred in the previous 24 h and collection of tissue occurred on the afternoon of day 3.
Blood and tissue collection
Two blood samples (approximately 10 mL) were collected by jugular venepuncture using a vacutainer with lithium heparin additive (Becton Dickinson from Export Lodge Veterinary Supplies; Levin, New Zealand). The first was collected at first observation of a mating mark and the second was collected just prior to tissue collection on day 3. Samples were centrifuged shortly after collection and plasma was stored at −20°C until utilised for determination of steroid concentrations.
On day 3 following observation of a mating mark, animals were humanely killed by captive bolt and exsanguination and their reproductive tracts were collected post-mortem. The number of corpora lutea (CL) with visible ovulation stigma on each ovary was recorded. Unfertilised oocytes or embryos were collected by flushing the uterine horn and oviduct twice with 5 mL of compound sodium lactate (Hartmann’s) solution (Baxter Healthcare Pty. Ltd, Toongabbie, Australia) with 1 mM 3-(N-morpholino) propanesulfonic acid (MOPS) using a syringe with a blunt needle. This solution was collected from a polyethylene tube (OD 2.5 mm, ID 1.5 mm, Tyco Electronics; Biocorp Aust Pty Ltd, Huntingdale, Victoria, Australia) inserted into the oviduct fimbria. An approximately 1 cm piece of the isthmus of the oviduct, adjacent to the utero-tubal junction, was collected from each side of the reproductive tract. Tissue was frozen in liquid nitrogen and stored at −80°C until processing for protein/RNA analysis. In animals with bilateral ovulations, one isthmus was fixed in 4% (w/v) paraformaldehyde and paraffin-embedded for immunohistochemistry (Smith et al. 2019). Only animals with a recovered unfertilised oocyte (non-pregnant; only exposed to the vasectomised ram) or embryo (pregnant group exposed to fertile ram) were utilised in downstream analysis.
Oestradiol and progesterone measurements
Concentrations of progesterone and oestradiol were measured in plasma samples using RIA kits (Ultra-sensitive Estradiol RIA kit, Beckman Coulter CA, USA and IBL Coat-a-Count RIA, IBL, Hamburg, Germany) as previously described (Smith et al. 2019; Juengel et al. 2020). Concentrations of oestradiol were measured from the samples collected on day 1 and 3, whereas concentrations of progesterone were only measured on day 3 given the known low concentrations of progesterone at the time of oestrus in sheep. The sensitivities of the assays were 2 pg/mL and 0.2 ng/mL for oestradiol and progesterone, respectively. No samples were below the sensitivity of the assays. The intra- and inter-assay coefficients of variation were all <16% for both hormones.
Isolation of RNA and protein
A total of six animals per age and group (24 total animals) were used for isolation of RNA and protein. Protein was extracted from the frozen oviduct tissue using a Nucleospin miRNA kit (Macherey-Nagel, Duren, Germany) following manufacturer’s instructions. The isolated protein was stored at −80°C in Protein Solving Buffer. Protein quantification was performed using a Protein Quantification Assay kit (Macherey-Nagel) as per the manufacturer’s instructions. Total RNA was also isolated from the frozen oviduct tissue using the Nucleospin miRNA kit as previously described (Quirke et al. 2021) with minor modifications. Samples were homogenised using an automated tissue homogeniser (SPEX SamplePrep 2010 Geno/Grinder, NJ, USA) with 2 × 3 mm stainless steel beads for 3 min at 1500 rpm. The RNA was eluted in 30 μL RNase-free water and stored at −80°C.
Immunohistochemistry
Tissue from six peripubertal (three each of pregnant and non-pregnant animals) and six adult (four non-pregnant, two pregnant animals) were used for immunohistochemistry. The sections were dewaxed, and antigen retrieval was performed in a microwave (1000 Watts for 12 min) in 0.01 M sodium citrate buffer at pH 6.0. Slides were quenched for 5 min in 3% H2O2, washed two times in PBS, blocked for 20 min in CAS-Block reagent (Cat#00812; Thermo Fisher, Auckland, New Zealand), then incubated in 2.5 μg/mL of rabbit anti-ERα antibody (Cat# 06-935; Merck, Auckland, New Zealand) overnight. Secondary antibody detection was performed using the DAKO EnVision + Dual Link System-HRP Rabbit/Mouse (DAKO, Glostrup, Denmark) with a 15 min diaminobenzidine (DAB) incubation followed by hematoxylin counterstaining. Negative control rabbit immunoglobulin fraction (Cat# X0936; DAKO) was used as a negative control at the same concentration as the primary antibody. Slides were imaged on an Aperio CS2 Digital Slide Scanner (Leica Biosystems, Victoria, Australia).
Western blotting
For each oviduct sample, 30 μg of protein was separated on a 12% SDS-PAGE acrylamide gel, under reducing conditions, and transferred to a nitrocellulose membrane. After blocking in Intercept blocking buffer (Millenium Science, Auckland, New Zealand), membranes were incubated overnight in 0.5 μg/mL rabbit IgG anti-ERα antibody (Cat# ab108398; Abcam) and mouse IgG1 anti-alpha tubulin at 0.09 μg/mL (Cat#38735; Cell Signalling, New England Biolabs, Auckland, New Zealand) as a loading control. Secondary antibody detection was performed using IRDye 800 CW goat anti-rabbit IgG at 1:10 000 dilution and IRDye 680 LT goat anti-mouse IgG1 at 1:20 000 dilution (Li-Cor, Millennium Science) and imaged with an Odyssey Classic scanner. This was repeated in two to three replicate western blots and relative signal intensities were compared between samples, normalised to alpha tubulin, using the Image Studio Lite software (Li-Cor).
Quantification of RNA expression
The nCounter Analysis System (NanoString Technologies Inc. Seattle, WA) was used to quantify expression of 131 genes related to immune function and steroid hormone signalling. The ProbeSet also included nine reference genes. The procedures, including the ProbeSet, described in Quirke et al. (2021) were used for RNA quantification. As expected, the normalisation factor for all samples was inside the 0.1–30 range.
Statistical analysis
Differences in live weight, ovulation rate, concentrations of progesterone and estradiol in plasma, and amount of ERα protein between age groups were determined by ANOVA. The model included effects of pregnancy status and the interaction of age and pregnancy status. For assessment of ERα protein quantification by western blot, the technical replicates were averaged per animal and the value was transformed (natural log) to improve normality of the data for analysis. Overall differences in gene expression between groups were determined using permutation ANOVA with 1 000 000 iterations. This was undertaken in R using the aovp function in the lmPerm package ver. 2.1.0 (Wheeler et al. 2016). The Benjamini-Hochberg (FDR) method (Benjamini and Hochberg 1995), undertaken with the p.adjust function in R, was used to correct for multiple testing. The model included both age and pregnancy status as well as their interaction. For pathway analysis, genes with uncorrected P-values below 0.05 were used given that the oviduct represents a highly complex system, the transcriptome only provides a ‘snapshot’ of gene expression at a single time point, and not all genes are expected to be highly differentially expressed at once. Gene categories were assigned and analysed as previously described (Quirke et al. 2021). Pearson correlation analysis between gene expression and oestradiol concentrations on day 1 and progesterone concentrations at time of tissue collection were undertaken as described by Quirke et al. (2021). Oestradiol concentrations on day 1 were chosen as previous work has shown an association among oestrous behaviour, embryo survival and oestradiol concentrations from plasma collected around this time (Juengel et al. 2020).
Results
General characterisation of animals
Information regarding weight, ovulation rate and concentrations of oestradiol and progesterone in plasma are shown in Table 1. No interactions were observed between age and pregnancy status for weight, ovulation rate or steroid concentrations. Therefore, pregnant and non-pregnant animals were grouped together for presentation. Peripubertal ewes weighed less than adult ewes, with a decreased ovulation rate. Concentrations of oestradiol did not differ between peripubertal and adult ewes at either time point, but concentrations of progesterone in plasma were increased in adult compared with peripubertal animals.
Measurement | Peripubertal ewe lamb | Adult ewe | |
---|---|---|---|
Live weight | 47.2 ± 1.3 | 70.5 ± 1.8*** | |
Ovulation rate | 2.1 ± 0.1 | 2.8 ± 0.2** | |
Oestradiol day 1 (pg/mL) | 9.97 ± 1.44 | 7.45 ± 0.86 | |
Oestradiol day 3 (pg/mL) | 7.21 ± 0.98 | 5.88 ± 1.01 | |
Progesterone day 3 (ng/mL) | 0.78 ± 0.08 | 1.21 ± 0.16* | |
ERα protein arbitrary units | 99.6 (73.8–134.5) | 59.1 (43.7–79.7)* |
*P < 0.05, **P < 0.01, ***P < 0.001. Values within rows differ from each other.
Expression of ERα in the oviduct
The pattern of expression of ERα in the oviduct was similar among all groups, with no obvious differences observed. As expected, signal was strongly expressed in the nucleus of the cell, with expression strongest in epithelial cells of the oviduct (Fig. 1). No interactions were observed between age and pregnancy status for amount of protein as determined by immunoblot. However, concentrations of ERα protein were increased in peripubertal ewe lambs compared with adult ewes (Table 1).
Photomicrographs of oviduct isthmus from peripubertal ewe lambs (a, b) and adult ewes (c, d) from day 3 of the oestrous cycle (a, c) or pregnancy (b, d). Nuclear staining (brown colour) for oestrogen receptor α (ERα) was observed in the nucleus of many cell types of the oviduct, with very strong staining in the epithelial cells. The inset in the top right-hand corner represents an increased magnification of the epithelium (arrows) and underlying stroma (S) cells. A photomicrograph of the negative control IgG is presented in the lower right-hand corner of panel d. Scale bar = 300 μm.
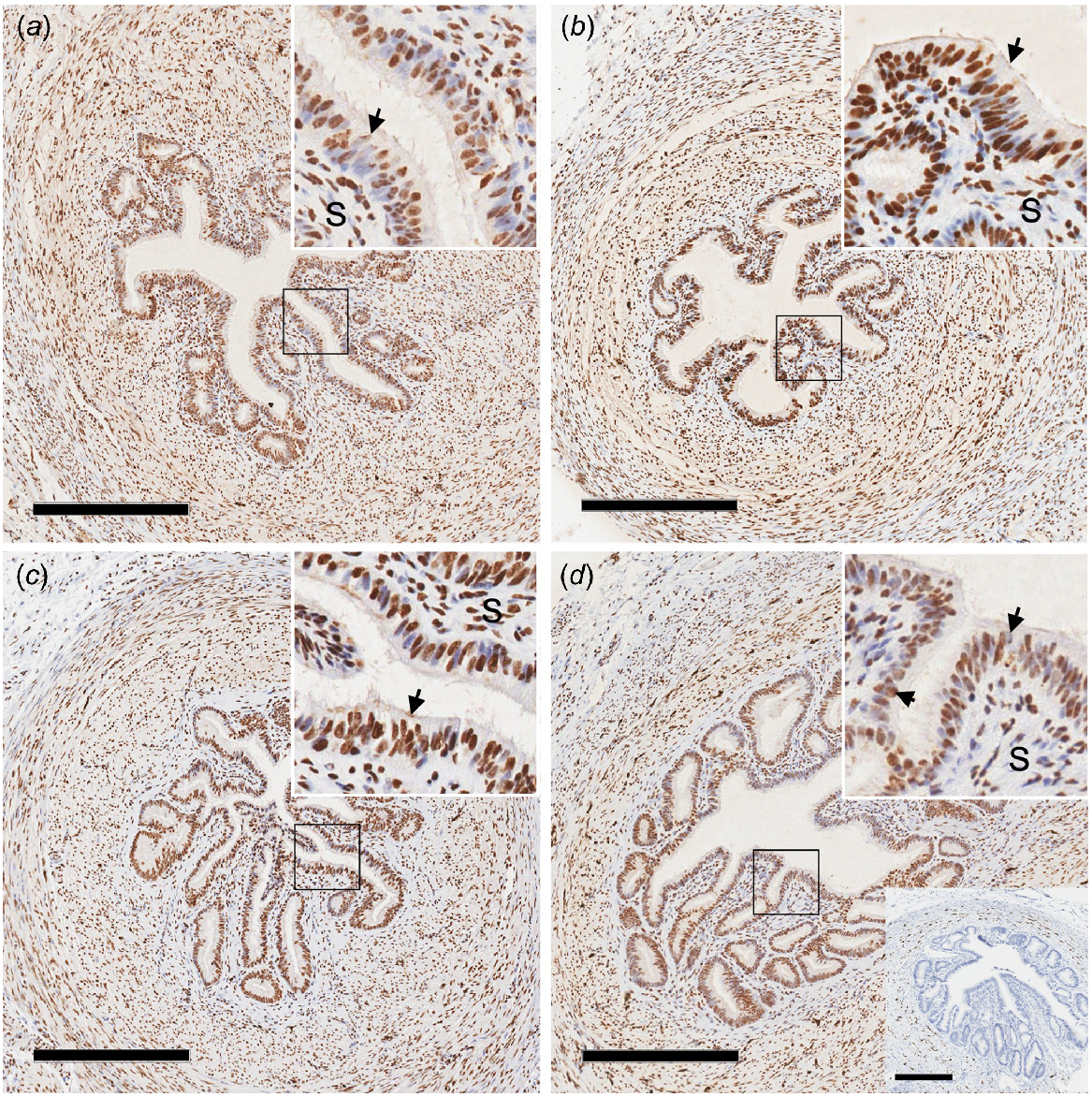
Permutation ANOVA analysis of the effects of age and pregnancy status on expression of individual genes
There were no genes with interactions between age and pregnancy that reached significance when adjusting for multiple comparisons. Likewise, when adjusted for multiple comparisons, pregnancy status did not significantly affect expression of any gene (FDR > 0.65). Differential expression of 13 genes was identified (FDR < 0.05) when comparing peripubertal to adult isthmus tissue. Expression of ESRRG, EPCAM, IGF1R, IL6, MCTP1, NFKB1, PAX8, PGR, PGRMC1 and PTX3 was decreased in peripubertal ewes compared to adult ewes (Table 2). In contrast, concentrations of mRNA for IL12RB1, NOS2 and SERPINE1 were increased in peripubertal compared with adult isthmus tissue (Table 2). Expression of four other genes also strongly tended (FDR < 0.055) to differ between peripubertal ewe lambs and adult ewes. Expression of ESR1 and EPHX1 was decreased, whereas CD86 and SELL was increased (Table 2).
Gene | FDR | Expression peripubertal/adult | Comments | |
---|---|---|---|---|
IGF1R | 0.004 | 0.75 ↓ | Growth factor | |
MCTP1 | 0.045 | 0.68 ↓ | Upregulated in oviduct in response to embryos | |
NFKB1 | 0.045 | 0.82 ↓ | Regulatory, multiple cell types | |
PAX8 | 0.045 | 0.67 ↓ | Regulatory, multiple cell types | |
EPCAM | 0.025 | 0.66 ↓ | Adhesion, epithelial cells and immune cells | |
SELL | 0.051 | 2.25 ↑ | Adhesion factor, expressed by leukocytes, homing to tissues | |
IL6 | 0.033 | 0.66 ↓ | Inflammatory but anti-inflammatory in muscle | |
IL12RB1 | 0.045 | 1.23 ↑ | Inflammatory | |
CD86 | 0.051 | 1.64 ↑ | Upregulated during antigen presentation | |
SERPINE1 | 0.045 | 1.66 ↑ | Protease inhibitor with antiangiogenic effects | |
PTX3 | 0.045 | 0.70 ↓ | Inflammatory also linked to oocyte quality | |
NOS2 | 0.045 | 1.63 ↑ | Inflammatory, generate ROS | |
EPHX1 | 0.054 | 0.73 ↓ | Removal of ROS | |
PGRMC1 | 0.045 | 0.82 ↓ | Progesterone signalling | |
PGR | 0.045 | 0.64 ↓ | Progesterone signalling | |
ESRRG | 0.045 | 0.60 ↓ | Oestrogen signalling pathway | |
ESR1 | 0.051 | 0.74 ↓ | Oestrogen signalling |
A downward arrow (↓) in expression indicates that expression was decreased in peripubertal ewe lambs compared with adult ewes, whereas an upwards arrow (↑) indicates expression was greater in peripubertal ewe lambs compared with adult ewes.
Pathway analysis
Given the weak separation of tissues based on pregnancy status, pathway analysis was undertaken only for differences relating to age. Focusing on immune and steroid hormone signalling pathways, several genes in the oestrogen signalling pathway were noted to be upregulated (P < 0.01) in the adult tissues compared with the oviducts from the peripubertal animals, including ESR1 itself with FDR = 0.051 (Fig. 2). While single genes relating to immune function were identified as differentially expressed between peripubertal and adult animals, with the limited gene numbers included in the nanostring analysis, no specific immune pathway was highlighted.
Schematic view of oestrogen signalling pathway. Genes coloured blue were differentially expressed between isthmus of the oviduct of peripubertal ewe lambs and adult ewes, with expression being decreased in the peripubertal ewe lambs. (Modified with permission from ‘KEGG map04915 estrogen signalling pathway’; Kanehisa 2019).
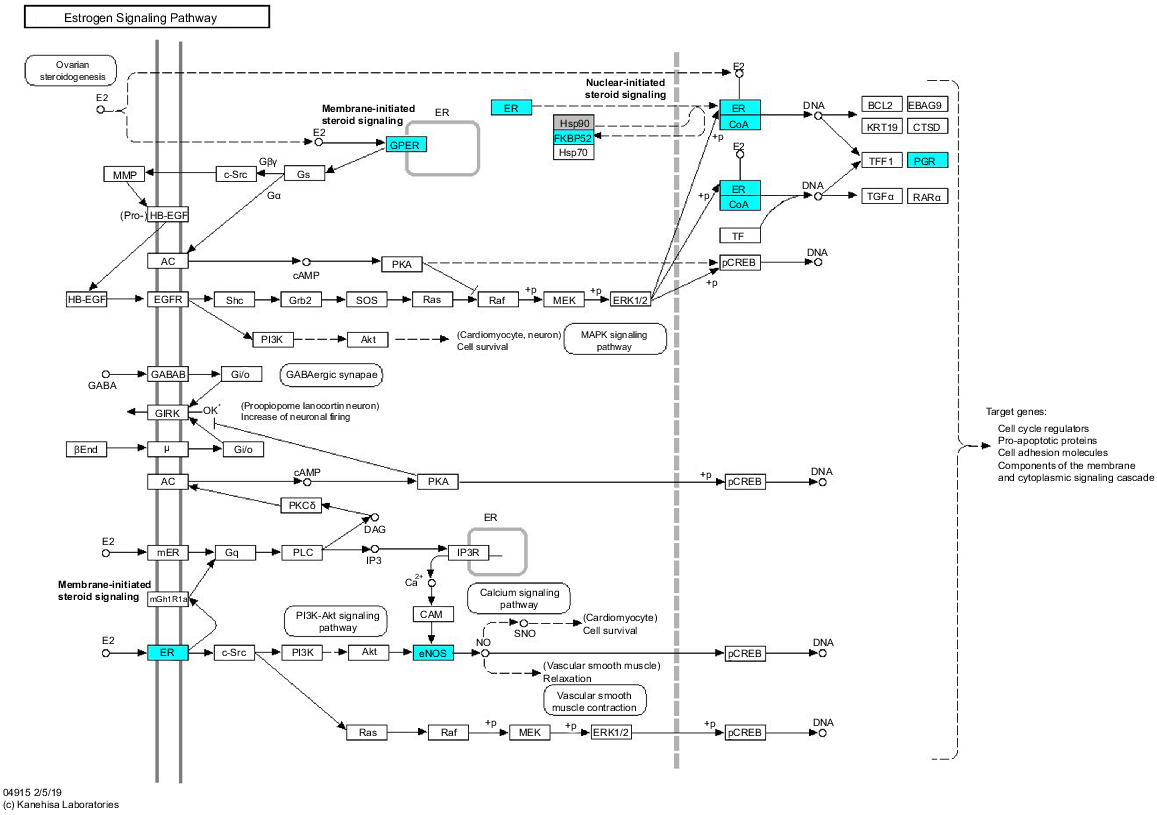
Correlation analysis between plasma steroid hormone concentration and gene expression
Correlations were observed between multiple genes and progesterone concentrations on day 3 and oestradiol concentrations on day 1 (Fig. 3). Highlighted among these correlations are the negative correlations between day 3 progesterone concentrations and TGFBR1 and TGFBR2, with there being a positive correlation between day 1 oestradiol concentrations and TGFB1. Two genes, NOS2 and CSFR2B, were positively correlated with day 1 oestradiol concentrations but negatively correlated with day 3 progesterone concentrations. Several genes known to be involved in regulation of immune function, such as FOXP3, IL4R, IL10RB, TNFRSF1A, INFAR1, DQB, ALOX5, CSF2RB, NOS2 and SERPRINE1, were also positively correlated with day 1 oestradiol concentrations.
Representation of correlations (indicated on the line for each gene) observed between selected genes and concentrations of oestradiol on day 1 (E2, D1) and progesterone on day 3 (P4, D3) of the reproductive cycle. Only genes with correlations greater than |0.50| were included and all are significant (P < 0.05).
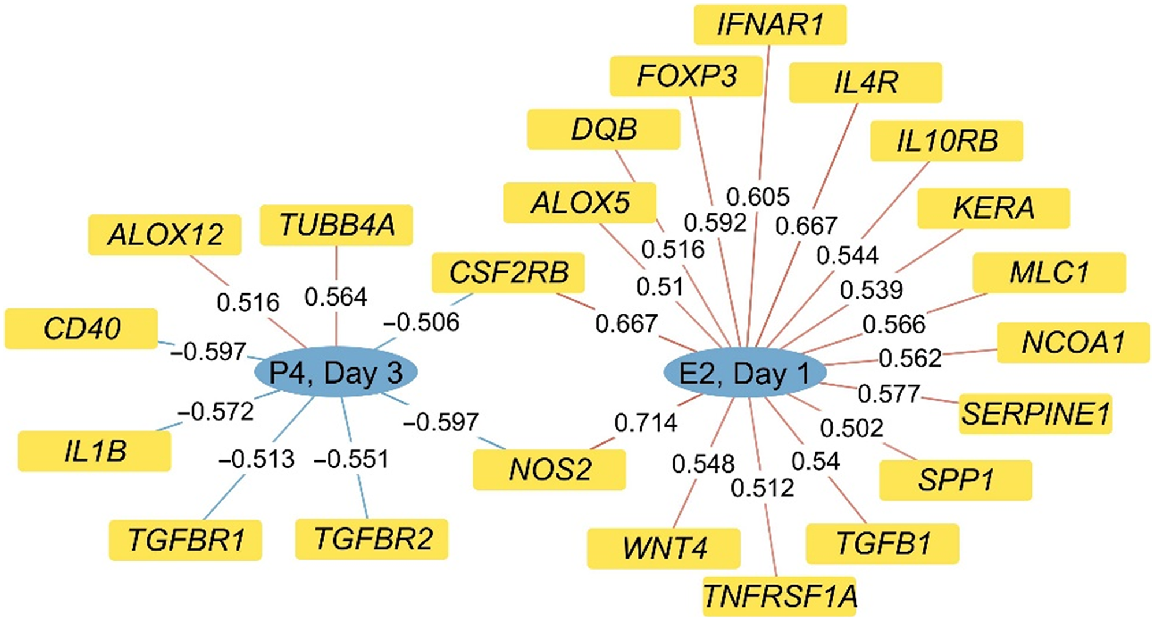
Discussion
Infertility is considered a major issue in both humans and livestock species (Schmidt 2006; Inhorn and Patrizio 2015; Leroy et al. 2015; Campanile et al. 2021). Infertility is a multifactorial issue, with factors influencing pregnancy loss spanning from poor quality oocytes, failure of fertilisation and early embryo development, failure of maternal recognition of pregnancy and implantation, to placental insufficiency and poor fetal growth and development. However, in multiple species, the very early stages, from fertilisation to early embryo development before maternal recognition of pregnancy, are the most vulnerable periods, when a majority of pregnancies fail (O’Connell et al. 2016b; Berg et al. 2022; Juengel et al. 2023). The current experiment highlighted potential functional differences in the isthmus of the oviduct between peripubertal and adult animals. These differences could at least partially underlie the known decrease in fertility observed in peripubertal ewe lambs when compared to adult ewes.
As hypothesised, there appears to be reduced oestrogen signalling in peripubertal animals as supported by pathway analysis for oestrogen receptor activation. This extended to include decreased expression of ESR1 mRNA as well as signalling co-factors (Co-A) and progesterone receptors. However, it is important to note that the amount of ERα protein in the isthmus was decreased in adult ewes compared with peripubertal ewe lambs. This appears in contrast to the apparent activation of the oestrogen signalling pathway when examining expression of various genes in this pathway. It is known that oestrogen can down-regulate ER protein expression independent of effects on its mRNA through ubiquitin-proteosome stimulated degradation of oestrogen receptor protein (Alarid et al. 1999; Nawaz et al. 1999). This was specific to this degradation pathway as calpain and lysosomal proteases were ineffective (Alarid et al. 1999). Potentially, this apparent discrepancy between ESR1 mRNA and protein expression patterns relates to differences in the turnover rates of the proteins in the cells. However, examination of the pathway as a whole supports decreased oestrogen signalling in the isthmus of the oviduct of the peripubertal ewe lamb compared with more mature adults.
Expression of several genes that are known to be involved in immune function were positively correlated to concentrations of oestradiol on the day of breeding. These included SERPINE1, NOS2, IL4R, IL10BR, IFNAR1, FOXP3, DQP, ALOX5, CSF2RB and TNFRS1A. This observation is consistent with oestrogen potentially being a key regulator of the immune environment of the oviduct. In mice lacking ESRα in epithelial cells of the oviduct, the oviduct developed an unfavourable environment, with increases in proteases and factors involved in immune function (Winuthayanon et al. 2015). Similarly, differences in gene expression in the oviduct of the peripubertal animal are in general consistent with an unfavourable environment. This included an increased expression of SELL, an adhesion factor expressed by leukocytes, facilitating homing to tissues (Ivetic et al. 2019). Also increased in expression were: IL12RB1, part of the receptor complex for IL12 and IL23, with known links to autoinflammatory diseases (Verstockt et al. 2023; Yousaf et al. 2023) and CD86, important for activation of T-cells for clonal expansion and acquisition of effector functions (Somoza and Lanier 1995) and IgG1 production from B cells (Podojil and Sanders 2005). Expression of NOS2, involved in synthesis of nitrous oxide and generation of reactive oxygen species (ROS) (Korhonen et al. 2005), was upregulated, whereas expression of EPHX1, which reduces reactive species (Cheong et al. 2009), was down-regulated, consistent with a more ROS active environment in the oviduct of peripubertal ewe lambs. Pregnancy has also been shown to upregulate EPHX1 in the oviduct on day 2–3 of pregnancy in pigs (Martyniak et al. 2018), and oviductal EPHX1 reduced ROS levels during preimplantation of mouse embryos and improved embryo development (Cheong et al. 2009) providing support for a role for this protein in facilitating early pregnancy.
Two genes potentially not fitting this pattern were the expression of IL6 and PTX3, which were down-regulated in the peripubertal ewe oviduct. While IL6 is generally known to be inflammatory, it also has anti-inflammatory effects (Xing et al. 1998; Scheller et al. 2011), with the differential expression of receptor sub-types on cells determining cellular response (Scheller et al. 2011). In the current analysis, RNA analysis was undertaken on the whole oviduct and thus which cell type(s) express IL6, and if the regulation in the different cell types is consistent, is unknown and worthy of further study. Additionally, IL6 is known to support early embryo development (Campanile et al. 2021). Addition of IL6 in culture media of in vitro–produced bovine embryos increased the number of inner mass cells and pregnancy establishment (Seekford et al. 2021). PTX3 can also be considered as a marker of an inflammatory environment, as it is upregulated by several inflammatory cytokines and is linked to inflammatory diseases (Geyer et al. 2021; Zhang et al. 2022). However, PTX3 can also have anti-inflammatory effects. A complex of hyaluronan, the heavy chains of inter-α-trypsin inhibition proteoglycan and PTX3, isolated from amniotic membranes, was shown to be anti-inflammatory in a model of chronic graft-versus-host disease (Ogawa et al. 2017). This complex inhibited M1 macrophage infiltration and supports polarising them to the M2 phenotype (He et al. 2014). This complex also forms in the cumulus cells and has been proposed to dampen leukocyte activity in the reproductive tract to help generate an optimal environment for fertilisation (Camaioni et al. 2018). It is important to note that PTX3 is also highly expressed in the cumulus cells. Comparative expression levels between granulosa and cumulus cells, and epithelial cells of the uterotubal junction/uterine horn in pigs provide evidence for much greater expression in the granulosa/cumulus cells (Waberski et al. 2018), whereas in goats, expression levels in the isthmus and ovary were similar (Fu et al. 2022). Research to better understand differences in PTX3 expression in the cumulus cells, as well as interactions with PTX3 expression in the oviduct would seem warranted to understand if reduced PTX3 expression may be a critical component of the increased fertilisation failure observed in the peripubertal ewe (Juengel et al. 2023).
It is also worth noting that expression of progesterone receptors, both the nuclear receptor and one component of the membrane progesterone receptor, along with progesterone concentrations itself, were decreased in peripubertal ewes compared with adult ewes. There are multiple studies in both sheep and cattle that link increased embryo survival to increased progesterone concentrations, including both peripheral and local circulation (Parr 1992; Mann 2001; Mann and Lamming 2001; Green et al. 2005; Robinson et al. 2008; O’Connell et al. 2013), although it should be noted that this relationship may be quadratic, with excess progesterone also detrimental to embryo health (Diskin et al. 2016). Expression of some genes was correlated with progesterone concentrations on day 3, with many of these correlations being negative. Of note were negative correlations with TGFBR1 and TGFBR2 and negative correlations with NOS2 and CSFR2B, as opposed to the positive correlations observed for NOS2 and CSFR2B with oestradiol concentrations on day of breeding. Given the potential negative role of ROS on embryo development (Cheong et al. 2009) and NOS2 role in generating ROS (Korhonen et al. 2005), suppression of NOS2 expression by progesterone could be one mechanism by which progesterone supports early embryo development. TGFB1, which signals through TGFBR1 and TGFBR2 (Heldin and Moustakas 2016), was identified as a key component in seminal plasma that stimulates CSF2 production and evokes an inflammatory-like reaction in reproductive tissue (Tremellen et al. 1998). Additionally, CSF2 was shown to be upregulated in response to Chlamydia trachomatis infection in reproductive tissue, consistent with an inflammatory response in the oviduct (Zhu et al. 2021). Thus, negative correlations between TGFBR1, TGFBR2 and CSF2RB expression and progesterone concentrations supports a role for progesterone in reducing inflammatory responses following the initial stimulation by mating. However, it should be noted that provision of CSF2 to embryo culture media enhances embryo development (Block et al. 2011), thus CSF2 most likely needs to be at optimum concentrations to support healthy embryo development, with either too much or too little detrimental. Taken together, this is consistent with progesterone being important for generating a favourable oviductal environment for fertilisation and early embryo growth.
The embryo can affect gene expression in oviductal cells (Schmaltz-Panneau et al. 2014; Hamdi et al. 2019; Passaro et al. 2019; Quirke et al. 2021). Therefore, differences in ovum or embryo quality between peripubertal and adult ewes that affect embryo secretions or direct interactions between the oviduct and gametes or embryos, could also drive differences in gene expression in oviduct. However, we did not observe any interactions between the age of the ewe and pregnancy status, supporting that the observed differences are likely inherent to the oviduct and not dependent on the presence of an embryo. Thus, the influence of the ovum or embryo in the observed differences in gene expression is likely to be minimal.
In conclusion, the isthmus of the oviduct of the peripubertal ewe, a model of poor fertilisation and reduced embryo survival (Juengel et al. 2023), had reduced steroid signalling and differential gene expression consistent with a more inflammatory environment than the more reproductively competent adult ewe. This unfavourable oviductal environment may be one of the key mechanisms underlying the poorer reproductive potential of peripubertal ewe lambs. Development of mechanisms to optimise the inflammatory response of the oviduct to mating, ensuring that the oviductal environment does not become hostile to fertilisation and early embryo development, may provide a novel approach to improve fertility, particularly in conditions associated with chronic low-grade inflammation such as polycystic ovarian syndrome, endometriosis and obesity (Herath et al. 2009; Broughton and Moley 2017; Amjadi et al. 2022; Bonavina and Taylor 2022).
Conflicts of interest
Jennifer L. Juengel is co-editor-in-chief of Reproduction, Fertility and Development. She was blinded to the review process throughout. All other authors declare they have no known conflicts of interest.
Declaration of funding
This work was supported by AgResearch’s Strategic Science Investment Fund from NZ Ministry of Business, Innovation and Employment. Approval for publication was granted by AgResearch.
Acknowledgements
The authors would like to thank Peter Smith for his help with collection of oviductal tissue. The authors would also like to thank the farm staff at Invermay for help with animal management and collection of tissue. Slide scanning was carried out in the Otago Micro and Nanoscale Imaging Histology unit at the University of Otago.
References
Alarid ET, Bakopoulos N, Solodin N (1999) Proteasome-mediated proteolysis of estrogen receptor: a novel component in autologous down-regulation. Molecular Endocrinology 13, 1522-1534.
| Crossref | Google Scholar | PubMed |
Amjadi F, Zandieh Z, Mehdizadeh M, Ajdary M, Aghamajidi A, Raoufi E, Aflatoonian R (2022) Molecular signature of immunological mechanism behind impaired endometrial receptivity in polycystic ovarian syndrome. Archives of Endocrinology and Metabolism 66, 303-311.
| Crossref | Google Scholar | PubMed |
Benjamini Y, Hochberg Y (1995) Controlling the false discovery rate: a practical and powerful approach to multiple testing. Journal of the Royal Statistical Society: Series B (Statistical Methodology) 57, 289-300.
| Google Scholar |
Berg DK, Ledgard A, Donnison M, McDonald R, Henderson HV, Meier S, Juengel JL, Burke CR (2022) The first week following insemination is the period of major pregnancy failure in pasture-grazed dairy cows. Journal of Dairy Science 105, 9253-9270.
| Crossref | Google Scholar | PubMed |
Block J, Hansen PJ, Loureiro B, Bonilla L (2011) Improving post-transfer survival of bovine embryos produced in vitro: actions of insulin-like growth factor-1, colony stimulating factor-2 and hyaluronan. Theriogenology 76, 1602-1609.
| Crossref | Google Scholar | PubMed |
Bonavina G, Taylor HS (2022) Endometriosis-associated infertility: from pathophysiology to tailored treatment. Frontiers in Endocrinology 13, 1020827.
| Crossref | Google Scholar | PubMed |
Broughton DE, Moley KH (2017) Obesity and female infertility: potential mediators of obesity’s impact. Fertility and Sterility 107, 840-847.
| Crossref | Google Scholar | PubMed |
Camaioni A, Klinger FG, Campagnolo L, Salustri A (2018) The influence of pentraxin 3 on the ovarian function and its impact on fertility. Frontiers in Immunology 9, 2808.
| Crossref | Google Scholar | PubMed |
Campanile G, Baruselli PS, Limone A, D’Occhio MJ (2021) Local action of cytokines and immune cells in communication between the conceptus and uterus during the critical period of early embryo development, attachment and implantation – implications for embryo survival in cattle: a review. Theriogenology 167, 1-12.
| Crossref | Google Scholar | PubMed |
Cheong AWY, Lee Y-L, Liu W-M, Yeung WSB, Lee K-F (2009) Oviductal microsomal epoxide hydrolase (EPHX1) reduces reactive oxygen species (ROS) level and enhances preimplantation mouse embryo development. Biology of Reproduction 81, 126-132.
| Crossref | Google Scholar | PubMed |
Diskin MG, Waters SM, Parr MH, Kenny DA (2016) Pregnancy losses in cattle: potential for improvement. Reproduction, Fertility and Development 28, 83-93.
| Crossref | Google Scholar | PubMed |
Edwards SJ, Juengel JL (2017) Limits on hogget lambing: the fertility of the young ewe. New Zealand Journal of Agricultural Research 60, 1-22.
| Crossref | Google Scholar |
Edwards SJ, Smaill B, O’Connell AR, Johnstone PD, Stevens DR, Quirke LD, Farquhar PA, Juengel JL (2016) Reduced ovulation rate, failure to be mated and fertilization failure/embryo loss are the underlying causes of poor reproductive performance in juvenile ewes. Animal Reproduction Science 167, 125-132.
| Crossref | Google Scholar | PubMed |
Ferre LB, Kjelland ME, Strobech LB, Hyttel P, Mermillod P, Ross PJ (2020) Review: recent advances in bovine in vitro embryo production: reproductive biotechnology history and methods. Animal 14, 991-1004.
| Crossref | Google Scholar | PubMed |
Fu K, Chen X, Guo W, Zhou Z, Zhang Y, Ji T, Yang P, Tian X, Wang W, Zou Y (2022) Effects of N acetylcysteine on the expression of genes associated with reproductive performance in the goat uterus during early gestation. Animals 12, 2431.
| Crossref | Google Scholar |
Geyer CE, Mes L, Newling M, den Dunnen J, Hoepel W (2021) Physiological and pathological inflammation induced by antibodies and pentraxins. Cells 10, 1175.
| Crossref | Google Scholar |
Gonzalez-Bulnes A, Veiga-Lopez A, Garcia P, Garcia-Garcia RM, Ariznavarreta C, Sanchez MA, Tresguerres JAF, Cocero MJ, Flores JM (2005) Effects of progestagens and prostaglandin analogues on ovarian function and embryo viability in sheep. Theriogenology 63, 2523-2534.
| Crossref | Google Scholar | PubMed |
Green MP, Hunter MG, Mann GE (2005) Relationships between maternal hormone secretion and embryo development on day 5 of pregnancy in dairy cows. Animal Reproduction Science 88, 179-189.
| Crossref | Google Scholar | PubMed |
Hamdi M, Sanchez-Calabuig MJ, Rodriguez-Alonso B, Bages Arnal S, Roussi K, Sturmey R, Gutierrez-Adan A, Lonergan P, Rizos D (2019) Gene expression and metabolic response of bovine oviduct epithelial cells to the early embryo. Reproduction 158, 85-94.
| Crossref | Google Scholar | PubMed |
He H, Tan Y, Duffort S, Perez VL, Tseng SCG (2014) In vivo downregulation of innate and adaptive immune responses in corneal allograft rejection by HC-HA/PTX3 complex purified from amniotic membrane. Investigative Opthalmology & Visual Science 55, 1647-1656.
| Crossref | Google Scholar | PubMed |
Heldin C-H, Moustakas A (2016) Signaling receptors for TGF-β family members. Cold Spring Harbor Perspectives in Biology 8, a022053.
| Crossref | Google Scholar |
Herath S, Lilly ST, Santos NR, Gilbert RO, Goetze L, Bryant CE, White JO, Cronin J, Sheldon IM (2009) Expression of genes associated with immunity in the endometrium of cattle with disparate postpartum uterine disease and fertility. Reproductive Biology and Endocrinology 7, 55.
| Crossref | Google Scholar | PubMed |
Inhorn MC, Patrizio P (2015) Infertility around the globe: new thinking on gender, reproductive technologies and global movements in the 21st century. Human Reproduction Update 21, 411-426.
| Crossref | Google Scholar | PubMed |
Ivetic A, Hoskins Green HL, Hart SJ (2019) L-selectin: a major regulator of leukocyte adhesion, migration and signaling. Frontiers in Immunology 10, 1068.
| Crossref | Google Scholar | PubMed |
Juengel JL, Quirke LD, Hyslop K, Meenken ED, Peers-Adams J, Smith P, Edwards SJ (2020) Association of fertility with group mating behavior in ewes. Animal Reproduction Science 216, 106359.
| Crossref | Google Scholar | PubMed |
Juengel JL, Quirke LD, Peers-Adams J, Johnstone PD, Smith P (2023) Embryo development and survival in peripubertal ewe lambs. Animal Production Science 63(12), 1177-1187.
| Crossref | Google Scholar |
Kanehisa M (2019) Toward understanding the origin and evolution of cellular organisms. Protein Science 28, 1947-1951.
| Crossref | Google Scholar | PubMed |
Korhonen R, Lahti A, Kankaanranta H, Moilanen E (2005) Nitric oxide production and signaling in inflammation. Current Drug Target -Inflammation & Allergy 4, 471-479.
| Crossref | Google Scholar | PubMed |
Leroy JLMR, Valckx SDM, Jordaens L, De Bie J, Desmet KLJ, Van Hoeck V, Britt JH, Marei WF, Bols PEJ (2015) Nutrition and maternal metabolic health in relation to oocyte and embryo quality: critical views on what we learned from the dairy cow model. Reproduction, Fertility and Development 27, 693-703.
| Crossref | Google Scholar | PubMed |
Madureira AML, Silper BF, Burnett TA, Polsky L, Cruppe LH, Veira DM, Vasconcelos JLM, Cerri RLA (2015) Factors affecting expression of estrus measured by activity monitors and conception risk of lactating dairy cows. Journal of Dairy Science 98, 7003-7014.
| Crossref | Google Scholar | PubMed |
Mann GE (2001) Pregnancy rates during experimentation in dairy cows. The Veterinary Journal 161, 301-305.
| Crossref | Google Scholar | PubMed |
Mann GE, Lamming GE (2001) Relationship between maternal endocrine environment, early embryo development and inhibition of the luteolytic mechanism in cows. Reproduction 121, 175-180.
| Crossref | Google Scholar | PubMed |
Martyniak M, Zglejc-Waszak K, Franczak A, Kotwica G (2018) Transcriptomic analysis of the oviduct of pigs during the peri-conceptional period. Animal Reproduction Science 197, 278-289.
| Crossref | Google Scholar | PubMed |
Nawaz Z, Lonard DM, Dennis AP, Smith CL, O’Malley BW (1999) Proteasome-dependent degradation of the human estrogen receptor. Proceedings of the National Academy of Sciences, USA 96, 1858.
| Crossref | Google Scholar |
Newman JE, Paul RC, Chambers GM (2022) Assisted reproductive technology in Australia and New Zealand 2020. Available at https://npesu.unsw.edu.au/sites/default/files/npesu/data_collection/Assisted%20Reproductive%20Technology%20in%20Australia%20and%20New%20Zealand%202020.pdf [Verified 3 July 2022]
O’Connell AR, Hurst PR, Davis GH, McNatty KP, Taylor SL, Juengel JL (2013) An earlier rise in systemic progesterone and increased progesterone in the uterine vein during early pregnancy are associated with enhanced embryonic survival in the ewe. Theriogenology 80, 269-274.
| Crossref | Google Scholar | PubMed |
O’Connell AR, McNatty KP, Hurst PR, Spencer TE, Bazer FW, Reader KL, Johnstone PD, Davis GH, Juengel JL (2016a) Activin A and follistatin during the oestrous cycle and early pregnancy in ewes. Journal of Endocrinology 228, 193-203.
| Crossref | Google Scholar | PubMed |
O’Connell AR, Demmers KJ, Smaill B, Reader KL, Juengel JL (2016b) Early embryo loss, morphology, and effect of previous immunization against androstenedione in the ewe. Theriogenology 86, 1285-1293.
| Crossref | Google Scholar | PubMed |
Ogawa Y, He H, Mukai S, Imada T, Nakamura S, Su C-W, Mahabole M, Tseng SCG, Tsubota K (2017) Heavy chain-hyaluronan/pentraxin 3 from amniotic membrane suppresses inflammation and scarring in murine lacrimal gland and conjunctiva of chronic graft-versus-host disease. Scientific Reports 7, 42195.
| Crossref | Google Scholar | PubMed |
Oussaid B, Mariana JC, Poulin N, Fontaine J, Lonergan P, Beckers JF, Cognie Y (1999) Reduction of the developmental competence of sheep oocytes by inhibition of LH pulses during the follicular phase with a GnRH antagonist. Journal of Reproduction and Fertility 117, 71-77.
| Crossref | Google Scholar | PubMed |
Parr RA (1992) Nutrition-progesterone interactions during early pregnancy in sheep. Reproduction, Fertility and Development 4, 297-300.
| Crossref | Google Scholar | PubMed |
Passaro C, Tutt D, Bages-Arnal S, Maicas C, Laguna-Barraza R, Gutierrez-Adan A, Browne JA, Rath D, Behura SK, Spencer TE, Fair T, Lonergan P (2019) Global transcriptomic response of bovine endometrium to blastocyst-stage embryos. Reproduction 158, 223-235.
| Crossref | Google Scholar | PubMed |
Phillips DJ, de Kretser DM, Hedger MP (2009) Activin and related proteins in inflammation: not just interested bystanders. Cytokine & Growth Factor Reviews 20, 153-164.
| Crossref | Google Scholar | PubMed |
Podojil JR, Sanders VM (2005) CD86 and β2-adrenergic receptor stimulation regulate B-cell activity cooperatively. Trends in Immunology 26, 180-185.
| Crossref | Google Scholar | PubMed |
Quirke JF, Hanrahan JP (1977) Comparison of the survival in the uteri of adult ewes of cleaved ova from adult ewes and ewe lambs. Journal of Reproduction and Fertility 51, 487-489.
| Crossref | Google Scholar | PubMed |
Quirke JF, Hanrahan JP (1983) Comparison of the survival of fertilized eggs from adult ewes in the uteri of adult ewes and ewe lambs. Journal of Reproduction and Fertility 68, 289-294.
| Crossref | Google Scholar | PubMed |
Quirke LD, Maclean PH, Haack NA, Edwards SJ, Heiser A, Juengel JL (2021) Characterization of local and peripheral immune system in pregnant and nonpregnant ewes. Journal of Animal Science 99, skab208.
| Crossref | Google Scholar |
Rajput SK, Lee KB, Zhenhua G, Di L, Folger JK, Smith GW (2013) Embryotropic actions of follistatin: paracrine and autocrine mediators of oocyte competence and embryo developmental progression. Reproduction, Fertility and Development 26, 37-47.
| Crossref | Google Scholar | PubMed |
Robinson RS, Hammond AJ, Wathes DC, Hunter MG, Mann GE (2008) Corpus luteum–endometrium–embryo interactions in the dairy cow: underlying mechanisms and clinical relevance. Reproduction in Domestic Animals 43(s2), 104-112.
| Crossref | Google Scholar | PubMed |
Saint-Dizier M, Mahe C, Reynaud K, Tsikis G, Mermillod P, Druart X (2020) Sperm interactions with the female reproductive tract: a key for successful fertilization in mammals. Molecular and Cellular Endocrinology 516, 110956.
| Crossref | Google Scholar | PubMed |
Scheller J, Chalaris A, Schmidt-Arras D, Rose-John S (2011) The pro- and anti-inflammatory properties of the cytokine interleukin-6. Biochimica et Biophysica Acta (BBA) – Molecular Cell Research 1813, 878-888.
| Crossref | Google Scholar | PubMed |
Schjenken JE, Robertson SA (2015) Seminal fluid signalling in the female reproductive tract: implications for reproductive success and offspring health. In ‘The male role in pregnancy loss and embryo implantation failure. Advances in Experimental Medicine and Biology. Vol. 868’. (Ed. R Bronson) pp. 127–158. (Springer) doi:10.1007/978-3-319-18881-2_6
Schmaltz-Panneau B, Cordova A, Dhorne-Pollet S, Hennequet-Antier C, Uzbekova S, Martinot E, Doret S, Martin P, Mermillod P, Locatelli Y (2014) Early bovine embryos regulate oviduct epithelial cell gene expression during in vitro co-culture. Animal Reproduction Science 149, 103-116.
| Crossref | Google Scholar | PubMed |
Schmidt L (2006) Psychosocial burden of infertility and assisted reproduction. The Lancet 367, 379-380.
| Crossref | Google Scholar | PubMed |
Seekford ZK, Wooldridge LK, Dias NW, Timlin CL, Sales AF, Speckhart SL, Pohler KG, Cockrum RR, Mercadante VRG, Ealy AD (2021) Interleukin-6 supplementation improves post-transfer embryonic and fetal development of in vitro-produced bovine embryos. Theriogenology 170, 15-22.
| Crossref | Google Scholar | PubMed |
Smith P, Stanton J-AL, Quirke L, Juengel JL (2019) Gestational nutrition 1: alterations to gestational nutrition can increase indicators of fertility in sheep. Reproduction 157, 199-213.
| Crossref | Google Scholar | PubMed |
Somoza C, Lanier LL (1995) T-cell costimulation via CD28-CD80/CD86 and CD40-CD40 ligand interactions. Research in Immunology 146, 171-176.
| Crossref | Google Scholar | PubMed |
Tremellen KP, Seamark RF, Robertson SA (1998) Seminal transforming growth factor β1, stimulates granulocyte-macrophage colony-stimulating factor production and inflammatory cell recruitment in the murine uterus. Biology of Reproduction 58, 1217-1225.
| Crossref | Google Scholar | PubMed |
Verstockt B, Salas A, Sands BE, Abraham C, Leibovitzh H, Neurath MF, Vande Casteele N, Alimentiv Translational Research Consortium (ATRC) (2023) IL-12 and IL-23 pathway inhibition in inflammatory bowel disease. Nature Reviews Gastroenterology & Hepatology 20, 433-446.
| Crossref | Google Scholar |
Waberski D, Schafer J, Bolling A, Scheld M, Henning H, Hambruch N, Schuberth H-J, Pfarrer C, Wrenzycki C, Hunter RHF (2018) Seminal plasma modulates the immune-cytokine network in the porcine uterine tissue and pre-ovulatory follicles. PLoS ONE 13, e0202654.
| Crossref | Google Scholar | PubMed |
Wheeler B, Torchiano M, Torchiano MM (2016) Package ‘lmPerm’. R package version, 2.1.0. Available at https://cran.r-project.org/package=lmPerm
Wijayarathna R, de Kretser DM (2016) Activins in reproductive biology and beyond. Human Reproduction Update 22, 342-357.
| Crossref | Google Scholar |
Winuthayanon W, Bernhardt ML, Padilla-Banks E, Myers PH, Edin ML, Lih FB, Hewitt SC, Korach KS, Williams CJ (2015) Oviductal estrogen receptor α signaling prevents protease-mediated embryo death. elife 4, e10453.
| Crossref | Google Scholar | PubMed |
Xing Z, Gauldie J, Cox G, Baumann H, Jordana M, Lei XF, Achong MK (1998) IL-6 is an antiinflammatory cytokine required for controlling local or systemic acute inflammatory responses. Journal of Clinical Investigation 101, 311-320.
| Crossref | Google Scholar | PubMed |
Yousaf H, Khan MIU, Ali I, Munir MU, Lee KY (2023) Emerging role of macrophages in non-infectious diseases: an update. Biomedicine & Pharmacotherapy 161, 114426.
| Crossref | Google Scholar | PubMed |
Zhang H, Wang R, Wang Z, Wu W, Zhang N, Zhang L, Hu J, Luo P, Zhang J, Liu Z, Feng S, Peng Y, Liu Z, Cheng Q (2022) Molecular insight into pentraxin-3: update advances in innate immunity, inflammation, tissue remodeling, diseases, and drug role. Biomedicine & Pharmacotherapy 156, 113783.
| Crossref | Google Scholar | PubMed |
Zhu G-D, Cao X-J, Li Y-P, Li J-X, Leng Z-J, Xie L-M, Guo X-G (2021) Identification of differentially expressed genes and signaling pathways in human conjunctiva and reproductive tract infected with Chlamydia trachomatis. Human Genomics 15, 22.
| Crossref | Google Scholar | PubMed |