Hepatitis B virus surface protein induces oxidative stress by increasing peroxides and inhibiting antioxidant defences in human spermatozoa
Lin Cheng A B C * , Pingnan Sun A B C * , Xiaoling Xie A B C , Dongmei Sun D , Qi Zhou A B C , Shaozhe Yang A B C , Qingdong Xie A B C and Xiaoling Zhou
A Stem Cell Research Center, Shantou University Medical College, Shantou 515041, PR China.
B Research Center for Reproductive Medicine, Shantou University Medical College, Shantou 515041, PR China.
C Guangdong Provincial Key Laboratory of Infectious Diseases and Molecular Immunopathology, Shantou University Medical College, Shantou 515041, PR China.
D Shenzhen Longgang District Maternity & Child Healthcare Hospital, Shenzhen 518172, PR China.
E Corresponding author. Email: xlzhou@stu.edu.cn
Reproduction, Fertility and Development 32(14) 1180-1189 https://doi.org/10.1071/RD20130
Submitted: 16 May 2020 Accepted: 10 August 2020 Published: 1 October 2020
Journal Compilation © CSIRO 2020 Open Access CC BY-NC-ND
Abstract
Hepatitis B virus (HBV) infection may affect sperm motility in patients with HBV. HBV surface protein (HBs) decreases mitochondrial membrane potential, impairs motility and induces apoptotic-like changes in human spermatozoa. However, little is known about how human spermatozoa respond to reactive oxygen species (ROS; mainly peroxides) induced by HBs. In this study, HBs induced supraphysiological ROS levels in human spermatozoa and reduced the formation of 2-cell embryos (obtained from hamster oocytes and human spermatozoa). HBs induced a pre-apoptotic status in human spermatozoa, as well as antioxidant defences by increasing glutathione peroxidase 4 (GPX4) and peroxiredoxin 5 (PRDX5) levels. These results highlight the molecular mechanism responsible for the oxidative stress in human spermatozoa exposed to HBV and the antioxidant defence response involving GPX4 and PRDX5.
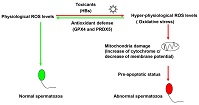
Keywords: 2-cell embryo, glutathione peroxidase 4 (GPX4), peroxiredoxin 5 (PRDX5).
Introduction
Worldwide, approximately two billion people are infected with hepatitis B virus (HBV), of whom 240 million are chronic carriers (Schweitzer Horn et al. 2015). Further, one million people die annually from HBV-related diseases, such as liver cirrhosis and hepatocellular carcinoma (Liaw and Chu 2009). As a prototype of the family Hepadnaviridae, HBV primarily replicates in and damages the human liver. However, HBV is also a sexually transmissible virus that may be present in human semen. In the 1980s, Scott et al. (1980) and Hadchouel (1985) reported that HBV was present in the semen of male HBV patients. Later, Huang et al. (2002) proposed that HBV may integrate into the chromosome and be vertically transmitted to offspring. HBV DNA (~2–3 × 105 copies mL–1) has been detected by real-time polymerase chain reaction (PCR) in the semen of HBV-infected patients (Qian et al. 2005). A population-based analysis revealed an increased incidence and risk of infertility among men with HBV infection compared with men without HBV (Su et al. 2014). The spermatozoa of HBV patients are less motile than spermatozoa of healthy individuals (Moretti et al. 2008a). In a clinical case-control study, couples in which the male was infected with HBV had a lower fertilisation rate (15.6% vs 34.4%; P = 0.036) and a decreased number of embryos available for transfer compared with uninfected couples (Oger et al. 2011). These results indicate that HBV may have adverse effects on human spermatozoa. In a previous study, we showed that treatment of human spermatozoa with HBV surface protein (HBs) reduces mitochondrial membrane potential and sperm motility, resulting in detrimental effects on sperm function with consequences for fertilisation (Zhou et al. 2009). Furthermore, we demonstrated that HBs could affect the integrity of the sperm membrane and trigger apoptotic-like changes (Kang et al. 2012; Huang et al. 2013). However, how human spermatozoa respond to stress caused by HBs remains unknown.
Mitochondria are critical organelles involved in supplying energy for spermatozoa (Piomboni et al. 2012). Sperm motility is strongly related to mitochondrial status (Marchetti et al. 2002). Mitochondria also are the major sources of reactive oxygen species (ROS). ROS plays an important role in sperm physiology and pathology (de Lamirande et al. 1997; Morielli and O’Flaherty 2015). At normal physiological concentrations, ROS support sperm capacitation (Aitken and Curry 2011). However, if there is an increase in ROS levels that exceeds the antioxidant capacity of human spermatozoa, the cells will enter a state of oxidative stress and the excessive ROS will induce lipid peroxidation and loss of motility in spermatozoa (Aitken et al. 2012). However, the antioxidant defence mechanisms in spermatozoa in response to peroxides generated by HBs are unknown. The aim of the present study was to characterise the response of human spermatozoa to stress caused by HBs and the key molecules involved in this process. The results demonstrated that HBs affects the mRNA profile of human spermatozoa. Based on this knowledge, we further identified the key molecules mediating oxidative stress caused by HBs treatment of human spermatozoa.
Materials and methods
Ethics approval and experimental design
Human spermatozoa and mature female hamsters were used in this study. Human sperm samples were obtained from healthy male donors. The hamsters were maintained under standard laboratory conditions (12-h light : dark cycle). This work was approved by the Ethics Review Committee of Shantou University Medical College and conformed to the National Institutes of Health guidelines for humane animal care and use in research, as well as the ethical guidelines of the 1975 Declaration of Helsinki, as reflected in a priori approval by Shantou University Medical College’s Human Research Committee.
The study first investigated the effects of HBs on the formation of and gene expression in 2-cell embryos (formed from hamster oocytes and human spermatozoa). Next, peroxide generation in HBs-treated human spermatozoa was confirmed. Finally, the effects of HBs on mitochondria-related gene enrichment were investigated and the key molecules involved in the process were identified.
Preparation of human spermatozoa
Each semen sample was kept at 37°C under a humidified 5% CO2 atmosphere for 30 min to allow liquefaction. Motile spermatozoa were selected by the swim-up method as follows: in each test tube, 0.5 mL liquefied semen sample was gently layered under 2 mL Biggers–Whitten–Whittingham (BWW) medium containing 0.3% bovine serum albumin (BSA) and incubated for 1 h at 37°C under 5% CO2 in a humidified incubator. Supernatant collected from three to four tubes was centrifuged at 300g for 5 min at room temperature, and the pellet of motile spermatozoa was washed once with BWW medium with 0.3% BSA and then resuspended in the same medium to a concentration ranging between 1 × 106 and 1 × 107 spermatozoa mL–1 for use in the subsequent experiments described in this paper.
IVF using human spermatozoa and hamster oocytes
Mature female Syrian golden hamsters were induced to superovulate by intraperitoneal injection of 40 IU pregnant mare’s serum gonadotrophin (Ningbo Hormone Product) on Day 1 of their oestrous cycle, followed by administration of 40 IU human chorionic gonadotrophin (hCG; Ningbo Hormone Product) 72 h later. Superovulated oocytes were collected from the ampullar region of the oviducts 17 h after hCG injection and freed from cumulus cells by treatment with 0.1% hyaluronidase (Sigma-Aldrich). Cumulus–free oocytes were washed twice in BWW medium with 0.3% BSA, incubated in BWW medium with 0.3% BSA and 0.1% trypsin (Sigma-Aldrich) for 2 min at 37°C to remove the zona pellucida and then washed twice in BWW medium with 0.3% BSA.
Washed spermatozoa were suspended in 5 mL BWW medium containing 10 μM calcium ionophore (A23187; Sigma-Aldrich) for 8 min to induce the acrosome reaction, and were then washed twice with BWW (with 0.3% BSA) and divided into two groups: Group 1 was treated with HBs (50 μg mL–1) during capacitation, and Group 2 was the untreated control. Spermatozoa in both groups were suspended in BWW with 3.0% BSA and incubated in a CO2 incubator (37°C, 5% CO2) for 4 h to allow capacitation. Oocytes were inseminated by placing them in sperm suspension (3.5 × 106 mL–1) for 20–40 min at 37°C, after which they were washed twice with embryo culture medium (as described in Table S1, available as Supplementary Material to this paper) and incubated in the same medium under mineral oil (Sigma-Aldrich) for another 24 h in a CO2 incubator (37°C, 5% CO2). The formation of 2-cell embryos was determined under a microscope and calculated as a percentage of the total number of oocytes.
RNA sequencing of 2-cell embryos
A sperm sample was collected from one healthy male volunteer and the sample was divided into two equal aliquots after sperm selection using the swim-up method described above. One of aliquots was treated with 50 μg mL–1 HBs for 3 h at 37°C, as described previously (Zhou et al. 2009), and the other aliquot was used as a control. These spermatozoa were used for IVF with golden hamster oocytes using the procedures described above. Approximately 20 2-cell embryos were collected in each group to prepare cDNA samples for RNA sequencing (RNA-seq) using a REPLI-g WTA single cell kit (Catalogue no. 150063; Qiagen). Human genes were subjected to Kyoto Encyclopedia of Genes and Genomes (KEGG) pathway analysis.
Determination of ROS and nitric oxide by flow cytometry
Human spermatozoa (1 × 106 spermatozoa mL–1), selected using the swim-up method, were incubated with 0, 25, 50 or 100 μg mL–1 HBs (HPLC purity >99%; NCPC GeneTech Biotechnology) for 3 h at 37°C and then washed three times with phosphate-buffered saline (PBS), pH 7.4 (Zhou et al. 2009). The sperm concentration was adjusted to 1 × 107 spermatozoa mL–1 before detection of ROS and nitric oxide (NO). ROS in human spermatozoa was detected using a Reactive Oxygen Species Assay kit (Beyotime) with 2,7-dichlorodihydrofluorescein diacetate (DCFH-DA); NO was detected by 4-amino-5 methylamino-2′,7′-difluorofluorescien diacetate (DAF-FM DA; Catalogue no. S0019; Beyotime). The green fluorescence signals of dichlorofluorescein (DCF) or DAF-FM were detected through the FL1 channel. All fluorescence-activated cell sorting (FACS) analyses were performed using a FACSCanto II Flow-Cytometer (BD Biosciences) equipped with an argon laser at 488 nm, forward scatter (FSC) detection at 0° relative to the laser, side scatter (SSC) detection at 90° and a green fluorescence detector at a mean (±s.d.) wavelength of 533 ± 15 nm. FSC and SSC were gated on the major population of normal-sized cells. A minimum of 10 000 cells per sample was analysed. Each sample was evaluated in triplicate. The relative proportion of cells within different areas of the fluorescence profiles was quantified using LYSYS II software (Becton-Dickinson). Data were acquired using FlowJo™ software (Becton-Dickinson) and collected on two plots using logarithmic scales (cell count vs green fluorescence channel). Results are expressed as mean fluorescence intensity (FI).
Switching Mechanism at 5′ end of RNA Template PCR and DNA array
Sperm samples were collected from eight healthy male volunteers, and each sample was divided into two equal aliquots after sperm selection using by the swim-up method described above. One aliquot was treated with 50 μg mL–1 HBs for 3 h at 37°C (Zhou et al. 2009), whereas the other was used as a control. Samples subjected to the same treatment were combined and RNA was extracted using TRIzol reagent (Invitrogen) and resuspended in 30 μL nuclease-free water (Ambion). The RNA template was used for reverse transcription and amplification using a Switching Mechanism at 5′ end of RNA Template (SMART) Pico PCR cDNA synthesis kit (Clontech) according to the manufacturer’s instructions. The resulting double-stranded cDNA was purified and assayed using a DNA microarray (NimbleGen). Mitochondria-related genes exhibiting twofold changes in the HBs-treated group relative to the control were identified and analysed using ArrayStar software (DNASTAR), KEGG pathway analysis and eXpression2Kinases (X2K) analysis (Clarke et al. 2018).
Real-time quantitative PCR
New sperm samples from three healthy male volunteers for real-time quantitative (q) PCR were prepared. Each sample was divided into two equal aliquots after being selected by the swim-up method described above. One of the aliquots was treated with 50 μg mL–1 HBs for 3 h at 37°C, and the other was used as a control. Total RNA was extracted using TRIzol (Invitrogen). Total RNA was reverse transcribed into cDNA with an RT-PCR Kit (FSQ-101; Toyobo) and qPCR was performed in triplicate for each sample using 2× Power SYBR Green Master Mix (Applied Biosystems) in an ABI 7500 PCR system (Thermo Fisher Scientific Inc.). Glyceraldehyde-3-phosphate dehydrogenase (GAPDH) was used for normalisation of input RNA. The real-time qPCR data were analysed using the 2–ΔΔCt method (Livak and Schmittgen 2001). The sequences of the primers used were as follows: glutathione peroxidase 4 (GPX4), 5′-ACCCGCTGTGGAAGTGGAT-3′ (forward) and 5′-CACGCAGCCGTTCTTGTCG-3′ (reverse); peroxiredoxin 5 (PRDX5), 5′-GCAAGACGGTGCAGTGAAG-3′ (forward) and 5′-ATGGCATCTCCCACCTTGATT-3′ (reverse); GAPDH, 5′-CACCATCTTCCAGGAGCGA-3′ (forward) and 5′-TCAGCAGAGGGGGCAGAGA-3′ (reverse). The concentration of all primers used was 250 nM. The qPCR program consisted of 5 min at 95°C, followed by 40 cycles of 95°C for 10 s and 60°C for 30 s. Samples pooled from different volunteers were analysed at least in triplicate.
Detection of cytochrome c released from sperm mitochondria
Human spermatozoa from three healthy male volunteers that had been selected using the swim-up method were incubated with HBs (0, 25, 50 or 100 μg mL–1) for 3 h at 37°C under a humidified 5% CO2 atmosphere, after which they were washed with 10 mL ice-cold PBS and collected by centrifugation at 600g for 5 min at room temperature. Sperm samples were pooled, and mitochondria and cytosol were extracted using a mitochondria/cytosol fractionation kit (BioVision) according to the manufacturer’s instructions. Briefly, the collected spermatozoa were resuspended in cytosol extraction buffer, placed on ice for 10 min and then homogenised using a Dura-Grind Dounce tissue grinder (Wheaton Instruments). The homogenised samples were transferred to 1.5-mL Eppendorf tubes and centrifuged at 700g for 10 min at 4°C. The supernatant was collected for cytosol proteins. The pellet was resuspended in mitochondria extraction buffer to obtain mitochondria proteins. The resulting protein samples were stored at –80°C before being used in the cytochrome c assay. Cytochrome c was detected by ELISA (Quantikine; R&D Systems) according to the manufacturer’s instructions. The samples were analysed in triplicate.
Western blotting
Each sample of motile spermatozoa (samples from eight healthy male volunteers) was divided into two equal aliquots after being selected by the swim-up method described above. One of aliquots was treated with 50 μg mL–1 HBs for 3 h at 37°C, whereas the other was used as a control. Sperm samples were collected by centrifugation at 600g for 5 min at 4°C and pooled together. Whole cell lysates were prepared using 300 μL RIPA buffer (150 mM NaCl, 1 mM EDTA, 100 mM Tris-HCl, 1% Triton X-100, 1% sodium deoxycholate, 0.1% sodium dodecyl sulfate, pH 7.4, supplemented with 1 : 500 protease inhibitor; Sigma-Aldrich) at 4°C for 1 h. The lysates were then centrifuged at 14 000g for 10 min at 4°C; the resulting supernatant was transferred to a new Eppendorf tube and the protein concentration was determined using a Pierce BCA protein assay kit (ThermoFisher). Proteins were separated by 10% sodium dodecyl sulfate–polyacrylamide gel electrophoresis and transferred to nitrocellulose membranes (Catalogue no. HAHY00010; Millipore) using the wet blot method. Membranes were then blocked with non-fat milk in Tris-buffered saline with 0.1% Tween-20 and incubated overnight at 4°C with a primary antibody against either: (1) β-actin (1 : 1000 dilution; Catalogue no. ZM-0001; ZSGB-Bio), washed with TBST for 10 min on a rocker incubator (repeated three times), followed by incubation with an infrared dye (IR800)-labelled goat anti-mouse IgG secondary antibody (LI-COR); or (2) active caspase-3 (1 : 1000 dilution; Catalogue no. AC033; Beyotime), washed with TBST for 10 min on a rocker incubator (repeated three times), followed by incubation with an infrared dye (IR700)-labelled goat anti-rabbit IgG secondary antibody (LI-COR). Images were obtained and quantified using Odyssey software (LI-COR).
GPX4 and PRDX5 assays
Human spermatozoa from three healthy male volunteers, selected using the swim-up method, were incubated with PBS (pH 7.4; control), 50 μg mL–1 HBs or 50 µg mL–1 HBs plus 50 µg mL–1 anti-HBs monoclonal antibody (mAb) for 3 h at 37°C under a humidified 5% CO2 atmosphere and then collected by centrifugation at 600g for 5 min at 37°C. The collected spermatozoa were washed twice with PBS (pH 7.4), centrifuged at 600g for 5 min at 37°C, pooled across volunteers and whole cell lysates prepared as described above. The lysates were centrifuged at 14 000g for 10 min at 4°C. The supernatant was collected, dithiothreitol (DTT) was added to a final concentration of 100 mM (O’Flaherty and de Souza 2011) and the samples were then used to determine GPX4 and PRDX5 levels using an ELISA kit (Shanghai Jimian) according to the manufacturer’s instructions. The samples were analysed at least in triplicate.
Statistical analysis
Data are presented as the mean ± s.d. Data were analysed using SPSS 11.0 (SPSS Inc.). After checking for normal data distribution and homogeneity of variances using the Shapiro–Wilk and Levene tests respectively, the significance of differences between groups were analysed using two-tailed independent-samples t-tests. The significance of differences in the fertilisation rate and fertilisation indices was analysed using the χ2 test. Continuity correction was used. P < 0.05 was considered significant.
Results
Effects of HBs on the formation of 2-cell embryos
In a previous study we showed that HBs reduces the fertilising ability of human spermatozoa (Zhou et al. 2009). In the present study we analysed the formation of 2-cell embryos after IVF with human spermatozoa (treated with or without HBs) and golden hamster oocytes. As expected, the number of 2-cell embryos formed was lower for human spermatozoa treated with than without HBs (33.90% vs 58.44% respectively; P < 0.01; Table 1). Therefore, HBs-induced sperm damage not only affects fertilisation (Zhou et al. 2009), but also the formation of 2-cell embryos.
We further investigated the effects of HBs on human gene expression in 2-cell embryos after IVF using HBs-treated spermatozoa. As shown in Fig. S1, the pathways primarily affected in 2-cell embryos following HBs treatment of spermatozoa were transport and catabolism, cell growth and death, signal transduction and infectious diseases. These results indicate that HBs-caused sperm damage could affect the development of early embryos.
Effects of HBs on oxidative stress in human spermatozoa
The effects of HBs on oxidative stress in human spermatozoa were examined, specifically the effects of HBs on levels of ROS and NO, important intracellular signalling molecules required at physiological levels for sperm capacitation, motility and fertilisation (Zini et al. 1993, 1995). After incubation of human spermatozoa (1 × 106 mL–1) in BWW medium with 0, 25, 50, or 100 μg mL–1 HBs for 3 h at 37°C, dose-dependent increases in both ROS (mainly peroxides) and NO were seen in HBs-treated compared with untreated spermatozoa (Fig. 1).
Effects of HBs on mitochondria-related molecules in human spermatozoa
HBs increases levels of peroxides and NO in human spermatozoa and decreases mitochondrial membrane potential and sperm motility (Zhou et al. 2009). However, the molecular changes induced by HBs in sperm mitochondria have yet to be characterised. Therefore, in this study RNA was extracted from spermatozoa from eight healthy donors that had been either treated or not with 50 μg mL–1 HBs. Gene expression arrays were used to analyse cDNA. In all 114 genes related to mitochondria were found to be differentially expressed (i.e. expression at least twofold higher or lower relative to control) in HBs-treated spermatozoa (Table S2). Using KEGG pathway analysis, eight genes were found to be involved in oxidative phosphorylation (Fig. 2). Twenty genes with large fold-change are indicated. Among these twenty genes, the expression of GPX4 and PRDX5 was 2.6- and 2.55-fold higher respectively in HBs-treated spermatozoa relative to control (Fig. 3). This indicates that the oxidative phosphorylation pathway in human spermatozoa is one of the major pathways affected by HBs treatment.
![]() |
![]() |
In addition, X2K analysis of the 114 mitochondria-related genes revealed that casein kinase 2α (CK2α), cyclin dependent kinase 1 (CDK1) and cyclin dependent kinase 4 (CDK4), three members of serine/threonine protein kinase family, could regulate these mitochondria-related molecules in human spermatozoa treated with HBs (Fig. 4).
Effects of HBs on caspase-3 in human spermatozoa
Protein levels of caspase-3 (active) were increased in human spermatozoa treated with 50 µg mL–1 HBs for 3 h compared with control (Fig. 5a; Fig. S2). Treatment of spermatozoa with 25, 50 and 100 µg mL–1 decreased cytochrome c concentrations in the mitochondria compared with those in the control group (27.47 ± 0.71 (P = 0.014), 25.06 ± 0.08 (P < 0.001) and 25.62 ± 0.04 (P < 0.001) vs 29.35 ± 0.30 nmol g–1 respectively), but dose-dependently increased cytochrome c concentrations in the cytoplasm (P < 0.01; Fig. 5b). These results indicate that HBs treatment induces a pre-apoptotic state in human spermatozoa.
![]() |
Antioxidant defences (GPX4 and PRDX5) in human spermatozoa
Antioxidant defences were induced in human spermatozoa in response to the oxidative stress caused by HBs treatment. This defence response involved increased peroxidase activity, including that of GPX4 and PRDX5. GPX4 and PRDX5 mRNA levels were higher in human spermatozoa treated with HBs than in the control group (Fig. 6a). Incubation of spermatozoa with 50 µg mL–1 HBs for 3 h resulted in higher GPX4 (approximately fivefold; P < 0.01) and PRDX5 (approximately twofold; P < 0.01) activity compared with control. Incubation of spermatozoa with 50 µg mL–1 HBs mAb in addition to 50 µg mL–1 HBs for 3 h, ameliorated the increase in GPX4 (P < 0.5) and PRDX5 (P > 0.5) levels (Fig. 6b, c).
Discussion
HBV infection has adverse effects on sperm quality (Huang et al. 2003; Vicari et al. 2006; Moretti et al. 2008b; Lorusso et al. 2010). HBV infection is negatively associated with the fertilisation rate of clinical IVF (Shi et al. 2014). Impaired sperm quality may be one reason for the lower fertilisation rate and higher rate of two pronuclei formation in couples in which the male is infected with HBV (Shi et al. 2014). In a previous study, we showed that HBs reduces sperm motility in a dose- and time-dependent manner and decreases sperm mitochondrial membrane potential (Zhou et al. 2009). It is of interest to determine the strategies human spermatozoa use to fight oxidative stress caused by HBs.
In this study we showed that HBs affects the expression of mitochondria-related genes in human spermatozoa. HBs causes oxidative stress in human spermatozoa and decreases the formation of 2-cell embryos. Generally, mammalian cells implement a series of stress responses, particularly mRNA turnover, after exposure to harmful stimuli (e.g. toxicants, oxidants or extreme temperature). mRNA turnover in cells involves the modulation of mRNA stabilisation and destabilisation by post-transcriptional regulation in addition to transcription (Friedel et al. 2009; Elkon et al. 2010; Rabani et al. 2011; Horvathova et al. 2017). In one study, mRNA stabilisation and destabilisation significantly affected the expression of more than half the stress-regulated genes (Fan et al. 2002).
Major histones are replaced by sperm-specific protamines in spermatozoa, but some histones are still retained at specific promoter regions (Yoshida et al. 2018). The nucleus of human spermatozoa also carries diverse RNA populations, including mRNAs (4000–5000 different mRNAs), antisense RNAs and microRNAs (miRNAs; Ostermeier et al. 2002; Dadoune 2009; Zhao et al. 2006; Sendler et al. 2013). Some RNAs play an important role in capacitation, fertilisation and embryo development (Bourc’his and Voinnet 2010). Importantly, PIWI-interacting RNA (piRNA) in germ cells also mediates the degradation of mRNA transcripts through miRNA- or short interference RNA-like mechanisms (Watanabe et al. 2015; Vourekas et al. 2016). Very recently, piRNA was demonstrated to have a dual function, eliminating mRNA and activating mRNA translation in mouse spermatids (Dai et al. 2019). It was widely believed that sperm were translationally silent. However, translation of sperm transcripts (RNA packed into spermatozoa) does occur in spermatozoa, but is mediated by mitochondrial-type ribosomes. In addition, sperm transcripts are substrates for sperm protein production (Gur and Breitbart 2006; Zhao et al. 2009). Recently, HBV gene transcription was confirmed in human spermatozoa and cytosine–phosphorous–guanine (CpG) methylation participates in the regulation of HBV gene expression in both host spermatozoa and sperm-derived embryos (Zhong et al. 2017, 2018).
A large-scale investigation of the human sperm proteome has revealed many signalling pathways, including oxidative phosphorylation and oxidative stress pathways (Wang et al. 2013). The results of the present study indicate that oxidative phosphorylation pathways are greatly involved in the oxidative stress response of human spermatozoa, which is consistent with the findings described above. In addition, X2K analysis of the 114 mitochondria-related molecules in HBs-treated spermatozoa indicated that serine/threonine protein kinases may mediate the sperm response to HBs-induced ROS. Modulation of piRNA machinery may be one of the possible mechanisms involved in the modulation of mRNA levels in human spermatozoa.
Generally, ROS are major contributors to oxidative stress. Excessive ROS damage the mitochondria and initiate an intrinsic apoptotic cascade in human spermatozoa, leading to a loss of sperm function. Physiological levels of ROS, such as superoxide (·O2–), hydrogen peroxide (H2O2) and nitric oxide (NO·), are essential for normal sperm capacitation (Aitken et al. 2012). The antioxidant defence in human spermatozoa comprises antioxidant enzymes, such as superoxide dismutase, catalase, GPX and PRDX. Members of the GPX family can remove H2O2 and other hydroperoxides by using reduced glutathione. GPX4 protects mitochondrial ATP generation against oxidative damage (Liang et al. 2007). Deletion of the selenoprotein GPX4 in spermatocytes results in male infertility in mice (Imai et al. 2009). Recently, it was shown that overexpression of GPX4 inhibits peroxide-induced cell death in diffuse large B cell lymphoma (Kinowaki et al. 2018). Members of the PRDX family can reduce a variety of ROS, including H2O2, organic hydroperoxides and ONOO– (O’Flaherty 2014a). PRDX5 is distributed in the plasma membrane, acrosome, nucleus, equatorial segment and midpiece of human spermatozoa, and its formation is dose-dependently modified by H2O2 (O’Flaherty and de Souza 2011; O’Flaherty 2014b).
We propose a model for the response of human spermatozoa to HBs-induced ROS generation. Normal sperm maintain ROS at physiological levels. However, in the presence of HBs, ROS levels increase in human spermatozoa, leading to oxidative stress. Human spermatozoa initiate an antioxidant defence response that involves increased levels of GPX4 and PRDX5, which restore ROS levels to physiological levels. In the absence of such a response, the constant supraphysiological levels of ROS would damage the mitochondria and decrease mitochondrial membrane potential, resulting in a pre-apoptotic state and abnormal spermatozoa.
In conclusion, this study shows that exposure of spermatozoa to HBs elicits both oxidative stress and defence responses. GPX4 and PRDX5 are key molecules involved in the antioxidant defence response of human spermatozoa.
Conflict of interest
The authors declare no conflicts of interest.
Acknowledgements
This work was supported by research grants from the National Nature Science Foundation of China (Grant no. 81870432, 81570567 and 81571994), the Scientific Research Foundation for the Returned Overseas Chinese Scholars, State Education Ministry and the 2020 Li Ka Shing Foundation Cross-Disciplinary Research Grant (No. L1111 2008). The authors thank Stanley Lin for help revising this manuscript.
References
Aitken, R. J., and Curry, B. J. (2011). Redox regulation of human sperm function: from the physiological control of sperm capacitation to the etiology of infertility and DNA damage in the germ line. Antioxid. Redox Signal. 14, 367–381.| Redox regulation of human sperm function: from the physiological control of sperm capacitation to the etiology of infertility and DNA damage in the germ line.Crossref | GoogleScholarGoogle Scholar | 20522002PubMed |
Aitken, R. J., Jones, K. T., and Robertson, S. A. (2012). Reactive oxygen species and sperm function – in sickness and in health. J. Androl. 33, 1096–1106.
| Reactive oxygen species and sperm function – in sickness and in health.Crossref | GoogleScholarGoogle Scholar | 22879525PubMed |
Bourc’his, D., and Voinnet, O. (2010). A small-RNA perspective on gametogenesis, fertilization, and early zygotic development. Science 330, 617–622.
| A small-RNA perspective on gametogenesis, fertilization, and early zygotic development.Crossref | GoogleScholarGoogle Scholar | 21030645PubMed |
Clarke, D. J. B., Kuleshov, M. V., Schilder, B. M., Torre, D., Duffy, M. E., Keenan, A. B., Lachmann, A., Feldmann, A. S., Gundersen, G. W., Silverstein, M. C., Wang, Z., and Ma’ayan, A. (2018). eXpression2Kinases (X2K) web: linking expression signatures to upstream cell signaling networks. Nucleic Acids Res 46, W171–W179.
| eXpression2Kinases (X2K) web: linking expression signatures to upstream cell signaling networks.Crossref | GoogleScholarGoogle Scholar |
Dadoune, J. P. (2009). Spermatozoal RNAs: what about their functions? Microsc. Res. Tech. 72, 536–551.
| Spermatozoal RNAs: what about their functions?Crossref | GoogleScholarGoogle Scholar | 19283828PubMed |
Dai, P., Wang, X., Gou, L. T., Li, Z. T., Wen, Z., Chen, Z. G., Hua, M. M., Zhong, A., Wang, L., Su, H., Wan, H., Qian, K., Liao, L., Li, J., Tian, B., Li, D., Fu, X. D., Shi, H. J., Zhou, Y., and Liu, M. F. (2019). A translation-activating function of MIWI/piRNA during mouse spermiogenesis. Cell 179, 1566–1581.e16.
| A translation-activating function of MIWI/piRNA during mouse spermiogenesis.Crossref | GoogleScholarGoogle Scholar | 31835033PubMed |
de Lamirande, E., Jiang, H., Zini, A., Kodama, H., and Gagnon, C. (1997). Reactive oxygen species and sperm physiology. Rev. Reprod. 2, 48–54.
| Reactive oxygen species and sperm physiology.Crossref | GoogleScholarGoogle Scholar | 9414465PubMed |
Elkon, R., Zlotorynski, E., Zeller, K. I., and Agami, R. (2010). Major role for mRNA stability in shaping the kinetics of gene induction. BMC Genomics 11, 259.
| Major role for mRNA stability in shaping the kinetics of gene induction.Crossref | GoogleScholarGoogle Scholar | 20409322PubMed |
Fan, J., Yang, X., Wang, W., Wood, W. H., Becker, K. G., and Gorospe, M. (2002). Global analysis of stress-regulated mRNA turnover by using cDNA arrays. Proc. Natl Acad. Sci. USA 99, 10611–10616.
| Global analysis of stress-regulated mRNA turnover by using cDNA arrays.Crossref | GoogleScholarGoogle Scholar | 12149460PubMed |
Friedel, C. C., Dolken, L., Ruzsics, Z., Koszinowski, U. H., and Zimmer, R. (2009). Conserved principles of mammalian transcriptional regulation revealed by RNA half-life. Nucleic Acids Res. 37, e115.
| Conserved principles of mammalian transcriptional regulation revealed by RNA half-life.Crossref | GoogleScholarGoogle Scholar | 19561200PubMed |
Gur, Y., and Breitbart, H. (2006). Mammalian sperm translate nuclear-encoded proteins by mitochondrial-type ribosomes. Genes Dev. 20, 411–416.
| Mammalian sperm translate nuclear-encoded proteins by mitochondrial-type ribosomes.Crossref | GoogleScholarGoogle Scholar | 16449571PubMed |
Hadchouel, M., Scotto, J., Huret, J. L., Molinie, C., Villa, E., Degos, F., and Brechot, C. (1985). Presence of HBV DNA in spermatozoa: a possible vertical transmission of HBV via the germ line. J. Med. Virol. 16, 61–66.
| Presence of HBV DNA in spermatozoa: a possible vertical transmission of HBV via the germ line.Crossref | GoogleScholarGoogle Scholar | 3840197PubMed |
Horvathova, I., Voigt, F., Kotrys, A. V., Zhan, Y., Artus-Revel, C. G., Eglinger, J., Stadler, M. B., Giorgetti, L., and Chao, J. A. (2017). The dynamics of mRNA turnover revealed by single-molecule imaging in single cells. Mol. Cell 68, 615–625.e9.
| The dynamics of mRNA turnover revealed by single-molecule imaging in single cells.Crossref | GoogleScholarGoogle Scholar | 29056324PubMed |
Huang, J. M., Huang, T. H., Qiu, H. Y., Fang, X. W., Zhuang, T. G., and Qiu, J. W. (2002). Studies on the integration of hepatitis B virus DNA sequence in human sperm chromosomes. Asian J. Androl. 4, 209–212.
| 12364978PubMed |
Huang, J. M., Huang, T. H., Qiu, H. Y., Fang, X. W., Zhuang, T. G., Liu, H. X., Wang, Y. H., Deng, L. Z., and Qiu, J. W. (2003). Effects of hepatitis B virus infection on human sperm chromosomes. World J. Gastroenterol. 9, 736–740.
| Effects of hepatitis B virus infection on human sperm chromosomes.Crossref | GoogleScholarGoogle Scholar | 12679922PubMed |
Huang, J., Zhong, Y., Fang, X., Xie, Q., Kang, X., Wu, R., Li, F., Xu, X., Lu, H., Xu, L., and Huang, T. (2013). Hepatitis B virus s protein enhances sperm apoptosis and reduces sperm fertilizing capacity in vitro. PLoS One 8, e68688.
| Hepatitis B virus s protein enhances sperm apoptosis and reduces sperm fertilizing capacity in vitro.Crossref | GoogleScholarGoogle Scholar | 24391762PubMed |
Imai, H., Hakkaku, N., Iwamoto, R., Suzuki, J., Suzuki, T., Tajima, Y., Konishi, K., Minami, S., Ichinose, S., Ishizaka, K., Shioda, S., Arata, S., Nishimura, M., Naito, S., and Nakagawa, Y. (2009). Depletion of selenoprotein GPx4 in spermatocytes causes male infertility in mice. J. Biol. Chem. 284, 32522–32532.
| Depletion of selenoprotein GPx4 in spermatocytes causes male infertility in mice.Crossref | GoogleScholarGoogle Scholar | 19783653PubMed |
Kang, X., Xie, Q., Zhou, X., Li, F., Huang, J., Liu, D., and Huang, T. (2012). Effects of hepatitis B virus s protein exposure on sperm membrane integrity and functions. PLoS One 7, e33471.
| Effects of hepatitis B virus s protein exposure on sperm membrane integrity and functions.Crossref | GoogleScholarGoogle Scholar | 23272113PubMed |
Kinowaki, Y., Kurata, M., Ishibashi, S., Ikeda, M., Tatsuzawa, A., Yamamoto, M., Miura, O., Kitagawa, M., and Yamamoto, K. (2018). Glutathione peroxidase 4 overexpression inhibits ROS-induced cell death in diffuse large B-cell lymphoma. Lab. Invest. 98, 609–619.
| Glutathione peroxidase 4 overexpression inhibits ROS-induced cell death in diffuse large B-cell lymphoma.Crossref | GoogleScholarGoogle Scholar | 29463878PubMed |
Liang, H., Van Remmen, H., Frohlich, V., Lechleiter, J., Richardson, A., and Ran, Q. (2007). Gpx4 protects mitochondrial ATP generation against oxidative damage. Biochem. Biophys. Res. Commun. 356, 893–898.
| Gpx4 protects mitochondrial ATP generation against oxidative damage.Crossref | GoogleScholarGoogle Scholar | 17395155PubMed |
Liaw, Y. F., and Chu, C. M. (2009). Hepatitis B virus infection. Lancet 373, 582–592.
| Hepatitis B virus infection.Crossref | GoogleScholarGoogle Scholar | 19217993PubMed |
Livak, K. J., and Schmittgen, T. D. (2001). Analysis of relative gene expression data using real-time quantitative PCR and the 2(–Delta Delta C(T)) method. Methods 25, 402–408.
| Analysis of relative gene expression data using real-time quantitative PCR and the 2(–Delta Delta C(T)) method.Crossref | GoogleScholarGoogle Scholar | 11846609PubMed |
Lorusso, F., Palmisano, M., Chironna, M., Vacca, M., Masciandaro, P., Bassi, E., Selvaggi Luigi, L., and Depalo, R. (2010). Impact of chronic viral diseases on semen parameters. Andrologia 42, 121–126.
| Impact of chronic viral diseases on semen parameters.Crossref | GoogleScholarGoogle Scholar | 20384803PubMed |
Marchetti, C., Obert, G., Deffosez, A., Formstecher, P., and Marchetti, P. (2002). Study of mitochondrial membrane potential, reactive oxygen species, DNA fragmentation and cell viability by flow cytometry in human sperm. Hum Reprod 17, 1257–1265.
| Study of mitochondrial membrane potential, reactive oxygen species, DNA fragmentation and cell viability by flow cytometry in human sperm.Crossref | GoogleScholarGoogle Scholar | 11980749PubMed |
Moretti, E., Federico, M. G., Giannerini, V., and Collodel, G. (2008a). Sperm ultrastructure and meiotic segregation in a group of patients with chronic hepatitis B and C. Andrologia 40, 173–178.
| Sperm ultrastructure and meiotic segregation in a group of patients with chronic hepatitis B and C.Crossref | GoogleScholarGoogle Scholar | 18477204PubMed |
Moretti, E., Federico, M. G., Giannerini, V., and Collodel, G. (2008b). Sperm ultrastructure and meiotic segregation in a group of patients with chronic hepatitis B and C. Andrologia 40, 286–291.
| Sperm ultrastructure and meiotic segregation in a group of patients with chronic hepatitis B and C.Crossref | GoogleScholarGoogle Scholar | 18811918PubMed |
Morielli, T., and O’Flaherty, C. (2015). Oxidative stress impairs function and increases redox protein modifications in human spermatozoa. Reproduction 149, 113–123.
| Oxidative stress impairs function and increases redox protein modifications in human spermatozoa.Crossref | GoogleScholarGoogle Scholar | 25385721PubMed |
O’Flaherty, C. (2014a). The enzymatic antioxidant system of human spermatozoa. Adv. Androl. 2014, 1–15.
| The enzymatic antioxidant system of human spermatozoa.Crossref | GoogleScholarGoogle Scholar |
O’Flaherty, C. (2014b). Peroxiredoxins: hidden players in the antioxidant defence of human spermatozoa. Basic Clin. Androl. 24, 4.
| Peroxiredoxins: hidden players in the antioxidant defence of human spermatozoa.Crossref | GoogleScholarGoogle Scholar | 25780579PubMed |
O’Flaherty, C., and de Souza, A. R. (2011). Hydrogen peroxide modifies human sperm peroxiredoxins in a dose-dependent manner. Biol. Reprod. 84, 238–247.
| Hydrogen peroxide modifies human sperm peroxiredoxins in a dose-dependent manner.Crossref | GoogleScholarGoogle Scholar | 20864641PubMed |
Oger, P., Yazbeck, C., Gervais, A., Dorphin, B., Gout, C., Jacquesson, L., Ayel, J. P., Kahn, V., and Rougier, N. (2011). Adverse effects of hepatitis B virus on sperm motility and fertilization ability during IVF. Reprod. Biomed. Online 23, 207–212.
| Adverse effects of hepatitis B virus on sperm motility and fertilization ability during IVF.Crossref | GoogleScholarGoogle Scholar | 21665545PubMed |
Ostermeier, G. C., Dix, D. J., Miller, D., Khatri, P., and Krawetz, S. A. (2002). Spermatozoal RNA profiles of normal fertile men. Lancet 360, 772–777.
| Spermatozoal RNA profiles of normal fertile men.Crossref | GoogleScholarGoogle Scholar | 12241836PubMed |
Piomboni, P., Focarelli, R., Stendardi, A., Ferramosca, A., and Zara, V. (2012). The role of mitochondria in energy production for human sperm motility. Int. J. Androl. 35, 109–124.
| The role of mitochondria in energy production for human sperm motility.Crossref | GoogleScholarGoogle Scholar | 21950496PubMed |
Qian, W. P., Tan, Y. Q., Chen, Y., Peng, Y., Li, Z., Lu, G. X., Lin, M. C., Kung, H. F., He, M. L., and Shing, L. K. (2005). Rapid quantification of semen hepatitis B virus DNA by real-time polymerase chain reaction. World J. Gastroenterol. 11, 5385–5389.
| Rapid quantification of semen hepatitis B virus DNA by real-time polymerase chain reaction.Crossref | GoogleScholarGoogle Scholar | 16149152PubMed |
Rabani, M., Levin, J. Z., Fan, L., Adiconis, X., Raychowdhury, R., Garber, M., Gnirke, A., Nusbaum, C., Hacohen, N., Friedman, N., Amit, I., and Regev, A. (2011). Metabolic labeling of RNA uncovers principles of RNA production and degradation dynamics in mammalian cells. Nat. Biotechnol. 29, 436–442.
| Metabolic labeling of RNA uncovers principles of RNA production and degradation dynamics in mammalian cells.Crossref | GoogleScholarGoogle Scholar | 21516085PubMed |
Schweitzer, A., Horn, J., Mikolajczyk, R. T., Krause, G., and Ott, J. J. (2015). Estimations of worldwide prevalence of chronic hepatitis B virus infection: a systematic review of data published between 1965 and 2013. Lancet 386, 1546–1555.
| Estimations of worldwide prevalence of chronic hepatitis B virus infection: a systematic review of data published between 1965 and 2013.Crossref | GoogleScholarGoogle Scholar | 26231459PubMed |
Scott, R. M., Snitbhan, R., Bancroft, W. H., Alter, H. J., and Tingpalapong, M. (1980). Experimental transmission of hepatitis B virus by semen and saliva. J. Infect. Dis. 142, 67–71.
| Experimental transmission of hepatitis B virus by semen and saliva.Crossref | GoogleScholarGoogle Scholar | 7400629PubMed |
Sendler, E., Johnson, G. D., Mao, S., Goodrich, R. J., Diamond, M. P., Hauser, R., and Krawetz, S. A. (2013). Stability, delivery and functions of human sperm RNAs at fertilization. Nucleic Acids Res. 41, 4104–4117.
| Stability, delivery and functions of human sperm RNAs at fertilization.Crossref | GoogleScholarGoogle Scholar | 23471003PubMed |
Shi, L., Liu, S., Zhao, W., Zhou, H., Ren, W., and Shi, J. (2014). Hepatitis B virus infection reduces fertilization ability during in vitro fertilization and embryo transfer. J. Med. Virol. 86, 1099–1104.
| Hepatitis B virus infection reduces fertilization ability during in vitro fertilization and embryo transfer.Crossref | GoogleScholarGoogle Scholar | 24760595PubMed |
Su, F. H., Chang, S. N., Sung, F. C., Su, C. T., Shieh, Y. H., Lin, C. C., and Yeh, C. C. (2014). Hepatitis B virus infection and the risk of male infertility: a population-based analysis. Fertil. Steril. 102, 1677–1684.
| Hepatitis B virus infection and the risk of male infertility: a population-based analysis.Crossref | GoogleScholarGoogle Scholar | 25439807PubMed |
Vicari, E., Arcoria, D., Di Mauro, C., Noto, R., Noto, Z., and La Vignera, S. (2006). Sperm output in patients with primary infertility and hepatitis B or C virus; negative influence of HBV infection during concomitant varicocele. Minerva Med. 97, 65–77.
| 16565700PubMed |
Vourekas, A., Alexiou, P., Vrettos, N., Maragkakis, M., and Mourelatos, Z. (2016). Sequence-dependent but not sequence-specific piRNA adhesion traps mRNAs to the germ plasm. Nature 531, 390–394.
| Sequence-dependent but not sequence-specific piRNA adhesion traps mRNAs to the germ plasm.Crossref | GoogleScholarGoogle Scholar | 26950602PubMed |
Wang, G., Guo, Y., Zhou, T., Shi, X., Yu, J., Yang, Y., Wu, Y., Wang, J., Liu, M., Chen, X., Tu, W., Zeng, Y., Jiang, M., Li, S., Zhang, P., Zhou, Q., Zheng, B., Yu, C., Zhou, Z., Guo, X., and Sha, J. (2013). In-depth proteomic analysis of the human sperm reveals complex protein compositions. J. Proteomics 79, 114–122.
| In-depth proteomic analysis of the human sperm reveals complex protein compositions.Crossref | GoogleScholarGoogle Scholar | 23268119PubMed |
Watanabe, T., Cheng, E. C., Zhong, M., and Lin, H. (2015). Retrotransposons and pseudogenes regulate mRNAs and lncRNAs via the piRNA pathway in the germline. Genome Res. 25, 368–380.
| Retrotransposons and pseudogenes regulate mRNAs and lncRNAs via the piRNA pathway in the germline.Crossref | GoogleScholarGoogle Scholar | 25480952PubMed |
Yoshida, K., Muratani, M., Araki, H., Miura, F., Suzuki, T., Dohmae, N., Katou, Y., Shirahige, K., Ito, T., and Ishii, S. (2018). Mapping of histone-binding sites in histone replacement-completed spermatozoa. Nat. Commun. 9, 3885.
| Mapping of histone-binding sites in histone replacement-completed spermatozoa.Crossref | GoogleScholarGoogle Scholar | 30250204PubMed |
Zhao, Y., Li, Q., Yao, C., Wang, Z., Zhou, Y., Wang, Y., Liu, L., Wang, L., and Qiao, Z. (2006). Characterization and quantification of mRNA transcripts in ejaculated spermatozoa of fertile men by serial analysis of gene expression. Hum. Reprod. 21, 1583–1590.
| Characterization and quantification of mRNA transcripts in ejaculated spermatozoa of fertile men by serial analysis of gene expression.Crossref | GoogleScholarGoogle Scholar | 16501037PubMed |
Zhao, C., Guo, X. J., Shi, Z. H., Wang, F. Q., Huang, X. Y., Huo, R., Zhu, H., Wang, X. R., Liu, J. Y., Zhou, Z. M., and Sha, J. H. (2009). Role of translation by mitochondrial-type ribosomes during sperm capacitation: an analysis based on a proteomic approach. Proteomics 9, 1385–1399.
| Role of translation by mitochondrial-type ribosomes during sperm capacitation: an analysis based on a proteomic approach.Crossref | GoogleScholarGoogle Scholar | 19253287PubMed |
Zhong, C., Lu, H., Han, T., Tan, X., Li, P., Huang, J., Xie, Q., Hou, Z., Qu, T., Jiang, Y., Wang, S., Xu, L., Zhong, Y., and Huang, T. (2017). CpG methylation participates in regulation of hepatitis B virus gene expression in host sperm and sperm-derived embryos. Epigenomics 9, 123–125.
| CpG methylation participates in regulation of hepatitis B virus gene expression in host sperm and sperm-derived embryos.Crossref | GoogleScholarGoogle Scholar | 27919171PubMed |
Zhong, Y., Liu, D. L., Ahmed, M. M. M., Li, P. H., Zhou, X. L., Xie, Q. D., Xu, X. Q., Han, T. T., Hou, Z. W., Huang, J. H., Xu, L., and Huang, T. H. (2018). Transcription and regulation of hepatitis B virus genes in host sperm cells. Asian J. Androl. 20, 284–289.
| Transcription and regulation of hepatitis B virus genes in host sperm cells.Crossref | GoogleScholarGoogle Scholar | 29111540PubMed |
Zhou, X. L., Sun, P. N., Huang, T. H., Xie, Q. D., Kang, X. J., and Liu, L. M. (2009). Effects of hepatitis B virus S protein on human sperm function. Hum. Reprod. 24, 1575–1583.
| Effects of hepatitis B virus S protein on human sperm function.Crossref | GoogleScholarGoogle Scholar | 19279032PubMed |
Zini, A., de Lamirande, E., and Gagnon, C. (1993). Reactive oxygen species in semen of infertile patients: levels of superoxide dismutase- and catalase-like activities in seminal plasma and spermatozoa. Int J Androl 16, 183–188.
| Reactive oxygen species in semen of infertile patients: levels of superoxide dismutase- and catalase-like activities in seminal plasma and spermatozoa.Crossref | GoogleScholarGoogle Scholar | 8359932PubMed |
Zini, A., De Lamirande, E., and Gagnon, C. (1995). Low levels of nitric oxide promote human sperm capacitation in vitro. J. Androl. 16, 424–431.
| 8575982PubMed |
* These authors contributed equally to this work.