Maternal periconceptional and first trimester protein restriction in beef heifers: effects on maternal performance and early fetal growth
Katrina J. Copping

A University of Adelaide, Robinson Research Institute, School of Medicine, North Terrace, Adelaide, SA 5005, Australia.
B South East Vets, 314 Commercial Street, Mount Gambier, SA 5290, Australia.
C The Chancellery, University of Newcastle, Callaghan, NSW 2308, Australia.
D Animal and Veterinary Sciences, University of Maine, Orono, ME 04469, USA.
E Corresponding author. Email: viv.perry@adelaide.edu.au
Reproduction, Fertility and Development 32(9) 835-850 https://doi.org/10.1071/RD19149
Submitted: 30 April 2019 Accepted: 10 January 2020 Published: 15 May 2020
Journal Compilation © CSIRO 2020 Open Access CC BY-NC-ND
Abstract
This study evaluated the effect of protein restriction during the periconception (PERI) and first trimester (POST) periods on maternal performance, physiology and early fetal growth. Yearling nulliparous heifers (n = 360) were individually fed a diet high or low in protein (HPeri and LPeri respectively) beginning 60 days before conception. From 24 to 98 days post-conception (dpc), half of each treatment group changed to the alternative post-conception high- or low-protein diet (HPost and LPost respectively), yielding four groups in a 2 × 2 factorial design with a common diet until parturition. Protein restriction was associated with lower bodyweight subsequent to reduced (but positive) average daily weight gain (ADG) during the PERI and POST periods. During the POST period, ADG was greater in LPeri than HPeri heifers and tended to be greater in LPost than HPost heifers during the second and third trimester. Bodyweight was similar at term. The pregnancy rate did not differ, but embryo loss between 23 and 36 dpc tended to be greater in LPeri than HPeri heifers. Overall, a greater proportion of male fetuses was detected (at 60 dpc 63.3% male vs 36.7% female). Protein restriction altered maternal plasma urea, non-esterified fatty acids, progesterone, leptin and insulin-like growth factor 1 at critical stages of fetal development. However, profiles varied depending on the sex of the conceptus.
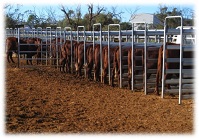
Additional keywords: conception, fetal programming, progesterone.
Introduction
Maternal nutrition has been reported to affect maternal reproductive performance, fetal development and postnatal performance of the offspring in ruminants (Edwards and McMillen 2002; MacLaughlin et al. 2005; Micke et al. 2011). Wide variations in natural feed resources in extensive farming systems are common. In the northern Australian rangelands, protein, rather than energy, is the major limiting nutrient (Norman 1963), with protein supplementation of replacement heifers consuming low-quality forage a common management practice (Burns et al. 2010). Embryo mortality in early gestation (from fertilisation to 45 days post-conception (dpc)) accounts for the majority of reproductive inefficiency in beef herds between conception and weaning (Burns et al. 2010). Consequently, investigations into the effects of protein supplementation on embryo survival, subsequent fetal development and postnatal progeny performance are important to the beef industry.
Development of ovarian follicles, oocytes and embryos, along with growth of the fetus and the placenta, are dependent on the hormonal and metabolic status of the cow. Multiple interrelated factors mediate the effects of nutrition on reproductive function and fetal development (Dupont et al. 2014); these factors include reproductive and/or metabolic hormone and endocrine signals (i.e. insulin growth factor (IGF; Sullivan et al. 2009a; Sferruzzi-Perri et al. 2011), glucocorticoids and leptin (Fowden and Forhead 2009)) and metabolic factors (i.e. urea and non-esterified fatty acids (NEFAs); Sullivan et al. 2009b).
Previously, we reported that periconception restriction of dietary protein in heifers reduces fetal growth in a sex-specific manner as early as 36 dpc in heifers (Copping et al. 2014; Micke et al. 2015). Conversely, excess dietary crude protein (CP) has been associated with decreased conception rates in dairy cattle (Butler et al. 1996), but the effects of either excess or restricted CP intake in beef cattle on reproductive function and early fetal development are less clear (Gunn et al. 2014; Amundson et al. 2016). Therefore, the aim of the present study was to evaluate the effects of protein restriction on the physiology of the developing yearling heifer, subsequent conception rates and early in utero fetal development. We hypothesised that low maternal dietary protein during the periconception period and early gestation would alter the metabolic and endocrine profiles of the dams, increase early embryo loss and reduce fetal development in a sex-dependent manner.
Materials and methods
Ethics approval
The use of animals and the procedures performed in this study complied with the Australian Code for the Care and Use of Animals for Scientific Purposes (National Health and Medical Research Council (NHMRC) 2004), and were approved by the University of South Australia IMVS Animal Ethics Committee (Approval no. 18/11) and The University of Adelaide, Australia (Approval no. S2012-249).
Experimental design and animal management
The aim of this study was to evaluate the effect of dietary protein restriction during the periconception (PERI; –60 to 23 dpc (implantation being 18–22 dpc; Wathes and Wooding 1980; Spencer et al. 2007)) and first trimester (POST; 24–98 dpc) periods on maternal performance and fetal development. The study was a 2 × 2 factorial design (see Fig. S1, available as Supplementary Material to this paper). The animals used in the study were the heifers and their progeny that have been described previously in brief (Copping et al. 2014) with dietary treatments reported in detail by Copping et al. (2017). In all, 360 nulliparous Santa Gertrudis (Bos taurus × Bos indicus) heifers were selected from S. Kidman & Co herds located in south-western Queensland, Australia. Heifers were transported to ‘Tungali’ (Sedan, SA, Australia, Australia; 34°29′S, 139°18′E) and underwent a 60-day acclimation period before commencement of the study. Heifers that did not acclimatise to feeding in individual stalls were not included in the study (n = 6).
At 12 months of age, 60 days before AI, heifers were randomly assigned to two PERI treatment groups (n = 177 in each): high and low protein (HPeri and LPeri respectively; from –60 to 23 dpc). Heifers in the HPeri group were fed a high-protein diet (71 MJ metabolisable energy (ME), 1.18 kg CP heifer–1 day–1), whereas those in the LPeri group were fed a low-protein diet (63 MJ ME, 0.62 kg CP heifer–1 day–1; Table S1), consisting of a measured pelleted diet fed individually in stalls each day. Straw (5% CP) was available ad libitum and intake was recorded on a group basis. The levels of dietary protein provided were formulated to reflect northern Australian rangeland pasture conditions without (low) and with (high) protein supplementation (Norman 1963). Both groups received similar energy intake, whereas protein intake was restricted during both the PERI and POST periods in the low group (Copping et al. 2017).
Heifers underwent synchronisation of oestrus using a progesterone-based program (Hernandez-Medrano et al. 2015) and were inseminated with frozen–thawed semen from one Santa Gertrudis bull on Day 0. At 23 dpc, half of each nutritional treatment group was swapped to the alternative POST treatment (from 24 to 98 dpc; Table S1), high (HPost; 102 MJ ME, 1.49 kg CP heifer–1 day–1) or low (LPost; 98 MJ ME, 0.88 kg CP heifer–1 day–1), giving rise to four groups: HPeri–HPost (HH), HPeri–LPost (HL), LPeri–HPost (LH) and LPeri–LPost (LL). Pregnancy was confirmed at 36 dpc. The sex of the fetus was determined at 60 dpc by rectal ultrasound. Fetuses were measured using transrectal ultrasonography (Sonosite M-Turbo) at 36, 60 and 95 dpc as reported previously (Copping et al. 2014). Briefly, measures taken at each respective time point were dependent on the stage of development and included crown–rump length (CRL), abdominal diameter (AD), umbilical cord diameter (UD), biparietal diameter (BPD), crown–nose length (CNL) and eye socket diameter (ED), as defined previously (Micke et al. 2010). Heifers that were identified by ultrasound as carrying twins (n = 3) and heifers with late embryo loss (after 36 dpcp; n = 15) were removed from the trial.
A subset of heifers (n = 46) was humanely slaughtered in a commercial abattoir at 98 dpc (Copping et al. 2014) and fetuses of both sexes were collected (singleton pregnancies; HH, 6 male, 6 female; HL, 10 male, 5 female; LH, 5 male, 5 female; LL, 4 male, 5 female). Fetal organ weights, fetal morphology and gross placenta parameters are reported in an accompanying paper (Copping et al. 2020). From the end of the first trimester of gestation (98 dpc), the remaining heifers (n = 64) were fed a common diet (Table S1), which was formulated to provide additional growth of 0.5 kg heifer–1 day–1 until parturition (from 99 dpc to term; 79 MJ ME, 0.92 kg CP heifer–1 day–1; Table 1). Heifers continued to receive the measured pellet portion of their diet individually on a daily basis with straw (5% CP) provided ad libitum until parturition (63 heifers, singletons: HH, 10 male, 8 female; HL, 14 male, 4 female; LH, 11 male, 4 female; LL, 9 male, 3 female). An additional heifer produced twins and is excluded from the analyses reported herein (LL, 2 male).
Heifers were weighed at approximately monthly intervals and immediately after birth. Average daily gain (ADG) was calculated by dividing the difference in bodyweight (BW) between the start and end of each diet period (PERI, POST, second and third trimesters) by the number of days after the start of that period.
Maternal blood sampling
Maternal blood samples were collected by tail venepuncture at approximately monthly intervals beginning 1 month before conception. Samples of whole blood were collected directly into 10-mL Vacutainer tubes containing 17 IU mL–1 lithium–heparin (Becton Dickinson). Tubes were gently inverted by hand for 5–10 s and placed on ice before centrifugation at 3000g for 10 min at 4°C (Eppendorf 5702R; Eppendorf Zentrifugen). Plasma was harvested and stored at –80°C until analysis.
Maternal metabolite and hormone assays
Bovine pregnancy-associated glycoproteins
A monoclonal-based bovine pregnancy-associated glycoprotein (bPAG) ELISA modified from Green et al. (2005) and as described by Pohler et al. (2016) was used to measure bPAG in plasma at 36, 60, 95, 128, 190 and 256 dpc and at birth. The assay sensitivity was 0.28 ng mL–1. The intra- and interassay CVs were 10.5% and 14.5% respectively.
Bovine placental lactogen
Plasma bovine placental lactogen (bPL) concentrations at 128, 190 and 256 dpc and at birth were determined in duplicate samples by radioimmunoassay (RIA; Wallace 1993). The assay sensitivity was 0.05 ng mL–1. The intra- and interassay CVs were 9.7% and 10.0% respectively.
Cortisol
Plasma cortisol concentrations at –19, 23, 36, 60, 95, 128, 190 and 256 dpc and at birth were determined using a commercial RIA kit (Clinical Assays, GammaCoat, Cortisol 125I RIA Kit; DiaSorin). Samples were assayed in duplicate 50-µL aliquots. The limit of detection of the assay was 1.0 ng mL–1. The intra-assay CVs for quality control samples containing 8.4 and 32.9 ng mL–1 were 5.0% and 7.1% respectively.
Leptin
Plasma leptin concentrations at –19, 23, 36, 60, 95, 128, 190 and 256 dpc and at birth were measured in duplicate in a single assay by a double-antibody RIA (Blache et al. 2000). The limit of detection of the assay was 0.05 ng mL–1. The assay included six replicates of three control samples containing 0.37, 0.99 and 1.73 ng mL–1, which were used to estimate intra-assay CVs of 2.54%, 6.05% and 6.34% respectively.
Insulin-like growth factor 1
Plasma IGF1 at 36, 60, 95, 128, 190 and 256 dpc and at birth was measured in duplicate in a single assay by double-antibody RIA with human recombinant IGF1 (ARM4050; Amersham-Pharmacia Biotech) and anti-human IGF1 antiserum (AFP4892898; National Hormone and Pituitary Program of the National Institute of Diabetes and Digestive and Kidney Diseases) after acid–ethanol extraction and cryoprecipitation (Breier et al. 1991). The limit of detection of this assay was 0.05 ng mL–1 and the intra-assay CVs for quality control samples containing 0.20 and 1.79 ng mL–1 were 7.2% and 3.9% respectively.
Insulin-like growth factor 2
Plasma concentrations of IGF2 at 36 dpc were measured in duplicate in a single assay as described previously (Forhead et al. 2011). Briefly, interference by binding proteins was minimised by the acid–ethanol cryoprecipitation method validated for ruminants (Breier et al. 1991). The reagents used were a highly purified human (h) IGF2 (Catalogue no. 031-30; Phoenix Pharmaceuticals), a rabbit antiserum to hIGF2 (PAC1; GroPep; 1 : 5000 dilution) and a 1 : 5 mixture of secondary antibody (donkey anti-rabbit; G4004; Jackson ImmunoResearch; 1 : 60 dilution) and normal rabbit serum (1 : 500 dilution). The assay was validated by checking for parallelism using a serial dilution of pooled plasma samples. The limit of detection was 0.05 ng mL–1 and the intra-assay CVs for quality control samples containing 1.23 and 2.58 ng mL–1 were 4.6% and 8.1% respectively.
Progesterone
Plasma progesterone concentrations at –19, 23, 36, 60, 95, 128, 190 and 256 dpc and at birth were determined by RIA using anti-progesterone antibody-coated tubes (RIA Progesterone #IM1188; Beckman Coulter Australia). The sensitivity of the assay was 0.10 ng mL–1 and the intra-assay CV for quality control samples containing 1.11 ng mL–1 was 1.29%; the interassay CV was 4.78%.
Metabolites
Metabolites, namely plasma urea and NEFA, were measured in plasma at –19, 23, 36, 60, 95, 128, 190 and 256 dpc and at birth. Samples were measured by enzymatic colourimetric analysis on an Olympus AU400 Auto analyser using commercially available kits (urea: UREA/BUN (Beckman-Coulter); NEFA: Wako NEFA-C (Novachem)). Samples were measured in six assays. The interassay CVs for quality control samples containing 6.46 and 21.93 mM urea were 2.18% and 2.54% respectively; for quality control samples containing 0.871 and 1.129 mEq L–1 NEFA, the interassay CVs were 2.43% and 2.06% respectively.
Statistical analysis
Statistical analyses were performed using STATA 13.1 (StataCorp). Data were checked for normality and transformed before analysis as required. Fisher’s exact χ2 test was used to analyse data regarding conception and embryo loss. Data for maternal BW and ADG for each of the PERI, POST and second and third trimester periods were analysed using two-way analysis of variance (ANOVA) to determine the effects of PERI and POST maternal diet and their interaction. Multifactorial ANOVA was used to interpret the effects of maternal diet during PERI and POST, fetal sex and their interaction terms on ultrasonography measures of fetal body dimensions at 36, 60 and 95 dpc in singleton progeny. In addition, multifactorial ANOVA were used to test the effects of PERI and POST maternal diet, fetal sex and their interactions on cortisol, leptin, progesterone, urea and NEFA at individual periods of pregnancy (–19, 23, 36, 60, 95, 128, 190 and 256 dpc and at birth), as well as on IGF1 (36, 60, 95, 128, 190 and 256 dpc and at birth), IGF2 (36 dpc), bPAG (36, 60, 95, 128, 190 and 256 dpc and at birth) and bPL (128, 190 and 256 dpc and at birth). Separate analyses were applied at each time point instead of a repeated-measures approach because maternal diet treatment and number of animals differed over time (i.e. –19 and 23 dpc had only PERI diet treatments and the remainder PERI and POST diet treatments with a subset of heifers (n = 46) killed at 98 dpc). Significant interactions were explored with one-way ANOVA and the Tukey–Kramer post hoc test as required. Interactions were not significant unless expressly stated in the results. For clarity, in the absence of significant interaction terms the data are presented according to the main effects of PERI and POST maternal diet. Ultrasonography measures and maternal hormone and metabolite data for male and female conceptuses were analysed together and separately. All data are presented as unadjusted mean ± s.e.m. unless stated otherwise. A P < 0.05 was considered significant.
Results
Maternal BW and ADG
Heifers were stratified according to BW and randomly allocated into maternal dietary treatment groups. Mean maternal BW at the start and end of each diet (PERI and POST) treatment period, along with ADG, is given in Table 1. Neither BW nor ADG was affected by fetal sex throughout any period of gestation (from –60 to 23 dpc, from 24 to 98 dpc, from 99 to 256 dpc and immediately after calving; all P > 0.10).
At the commencement of the experiment, the BW of heifers allocated to the LPeri and HPeri groups was similar (P > 0.10). However, at the end of the PERI diet period (from –60 to 23 dpc), BW was lower in LPeri than HPeri heifers (P = 0.04), consequent to the moderately reduced ADG in LPeri heifers during the PERI diet period (P = 0.0001).
At the start of the POST diet period (24–98 dpc), the BW of LPost and HPost heifers was similar (P = 0.69), reflecting the reallocation of heifers from the LPeri and HPeri diets as part of the crossover experimental design. At the end of the POST period, BW was lower in LPost than HPost heifers (P = 0.02). There was no interaction effect of the PERI and POST diets (P = 0.30) at the end of the POST diet period. ADG during the POST diet period was reduced in LPost heifers (P = 0.0003). There was no diet interaction effect for ADG (P = 0.82); however, heifers that had been fed the LPeri diet had a greater ADG during the POST diet period (24–98 dpc) than HPeri heifers (0.38 ± 0.03 vs 0.13 ± 0.02 kg head–1 day–1; P < 0.0001).
From the end of the POST diet period to term, the remaining heifers received the same individually fed diet. At 256 dpc, BW did not differ due to dietary treatment (LPeri vs HPeri: 506.4 ± 5.8 vs 502.7 ± 5.2 kg respectively (P = 0.69); LPost vs HPost: 505.8 ± 5.2 vs 502.8 ± 5.7 kg respectively (P = 0.92)). ADG between 99 and 256 dpc tended to be greater in heifers fed the LPost than HPost diet (0.65 ± 0.02 vs 0.60 ± 0.02 kg head–1 day–1 respectively; P = 0.05). ADG did not differ due to PERI diet (0.62 ± 0.02 vs 0.62 ± 0.02 kg head–1 day–1 in the LPeri and HPeri groups respectively; P = 0.77), nor was there a diet interaction (P = 0.23). Similarly, immediately after calving BW did not differ due to dietary treatment (LPeri vs HPeri: 466.5 ± 6.9 vs 456.0 ± 5.2 kg respectively (P = 0.28); LPost vs HPost: 458.8 ± 5.2 vs 462.2 ± 6.6 kg respectively (P = 0.55)).
Pregnancy rates and progeny sex ratio
The overall pregnancy rate, defined as the number of heifers with a fetus at 36 dpc (singletons, n = 124 heifers; twins, n = 4 heifers) as identified by ultrasonography, was 36.3%. There was no difference in conception rates for heifers receiving the LPeri versus HPeri diet during the periconception period (from –60 to 23 dpc; 33.0% vs 39.6% respectively; P = 0.22). From the laboratory assay used, 7 ng mL–1 progesterone in maternal plasma was considered indicative of pregnancy at this point (Starbuck et al. 2004). By this measure, 26 heifers lost the embryo between 23 and 36 dpc; 19 of these received the LPeri diet during the PERI period (P = 0.088).
Heifers with singleton fetuses that were either necropsied at 98 dpc (n = 46) or calved at term (n = 63) had a greater proportion of male fetuses than females overall (63.3% vs 36.7%; P = 0.009). However, the proportion of males was similar between the two PERI diet groups (63.0% vs 63.5% in the LPeri and HPeri groups respectively; P > 0.10).
Maternal hormones and metabolites
Progesterone
Overall, heifers fed the LPeri diet had reduced circulating maternal plasma progesterone concentrations at 23 and 36 dpc compared with heifers fed the HPeri diet (Fig. 1; P < 0.05). At 95 dpc, progesterone tended to be higher in heifers fed the LPeri diet (P = 0.09) and in those fed the HPost diet (P = 0.08). At 128 dpc, progesterone varied with an interaction between POST diet and fetal sex (P = 0.04), and tended to vary with an interaction between PERI diet and fetal sex (P = 0.06). These apparently discordant effects were contingent upon fetal sex, as described below.
When analysed separately, within heifers with female fetuses (Fig. 2a, c), progesterone concentrations were reduced at 23 dpc in LPeri versus HPeri heifers (P < 0.05). At 128 and 190 dpc, progesterone was elevated in LPost versus heifers HPost (P < 0.05).
![]() |
Within heifers carrying male fetuses (Fig. 2b, d), progesterone was reduced at 36 dpc in LPeri versus HPeri heifers (P = 0.02). By 95 dpc, POST diet affected progesterone, with concentrations reduced in LPost versus HPost heifers (P = 0.004). At 128 dpc, a carryover effect of the PERI diet was observed, with progesterone tending to be higher (P = 0.06) in LPeri than HPeri heifers.
Leptin
Overall, at 19 days before conception, at 23 dpc and at 95 dpc maternal plasma leptin concentrations varied with an interaction between PERI maternal diet and subsequent fetal sex (P < 0.05), as described below. Also at 95 dpc, leptin concentrations were lower LPost than HPost heifers (P = 0.013). At 128 and 190 dpc, leptin tended to be reduced overall in LPost versus HPost heifers (both P < 0.10).
When sexes were analysed separately (Fig. 3), leptin concentrations in those heifers subsequently determined to be carrying females were lower at 19 days before conception in LPeri than HPeri heifers (P < 0.05), but were similar at 23 dpc (P = 0.11). By 95 dpc, the POST diet had affected leptin, with reduced concentrations in LPost than HPost heifers (P = 0.04). At 128 dpc, leptin tended to be lower in LPost heifers (P = 0.06).
![]() |
Within heifers pregnant with male fetuses, leptin concentrations did not differ due to the PERI diet at either –19 or 23 dpc. At 95 dpc, leptin concentrations were lower in LPost than HPost heifers, and there was a carryover effect of the PERI diet, with leptin concentrations greater in LPeri than HPeri heifers (P < 0.05). This effect was still observed later in pregnancy, with leptin concentrations at both 128 dpc and birth tending to be greater in LPeri than HPeri heifers (both P < 0.10).
Insulin-like growth factor 1
Overall, at 36 dpc maternal plasma IGF1 concentrations tended to be increased in the LPeri versus HPeri group (P = 0.09). At 60 dpc, the LPost diet increased plasma IGF1 (P = 0.04). Within heifers with male (Fig. 4b, d) but not female (Fig. 4a, c) fetuses, plasma IGF1concentrations at 36 dpc were elevated by both the LPeri (P = 0.01) and LPost (P = 0.04) diets and tended to be greater in LPost than HPost heifers at 60 dpc (P = 0.09).
![]() |
Insulin-like growth factor 2
Maternal plasma IGF2 concentration at 36 dpc varied with an interaction between PERI diet and fetal sex (P = 0.02). When analysed separately, in heifers with female fetuses (Fig. 5), IGF2 concentrations were lower in LPeri than HPeri heifers (P = 0.02), but did not differ according to the POST diet (P > 0.10). In heifers with male fetuses (Fig. 5), IGF2 concentrations were not altered by maternal diet (P > 0.10). Overall, heifers carrying male fetuses tended to have reduced IGF2 concentrations at 36 dpc compared with those carrying with female fetuses (60.7 ± 1.6 vs 66.7 ± 2.1 ng mL–1 respectively; P = 0.07).
Cortisol, bPL and bPAG
Maternal diet and fetal sex did not affect maternal plasma cortisol, bPAG or bPL concentrations when analysed overall with sexes combined or separately (Figs S2–S4; P > 0.10), except within heifers carrying male fetuses at 190 dpc, with bPL tending to be reduced in LPost versus HPost heifers (P = 0.06), and in heifers carrying females, in which cortisol concentrations tended to be elevated at birth in LPeri versus HPeri heifers (P = 0.06).
Non-esterified fatty acids
Overall, at 19 days before conception, maternal plasma NEFA concentrations were lower in LPeri than HPeri heifers (P = 0.006). At 36 dpc, NEFA tended to be lower in LPost than HPost heifers (P = 0.06). At 95 dpc, this pattern was reversed, with NEFA tending to be greater in LPost heifers (P = 0.06). At 23 dpc, NEFA was less overall in heifers carrying male fetuses compared with those carrying female fetuses (0.11 ± 0.01 vs 0.15 ± 0.02 nM respectively; P = 0.04). Maternal diet and fetal sex did not affect NEFA concentrations at any other point (all P > 0.10).
When analysed separately, within heifers carrying female fetuses, maternal diet did not effect NEFA concentrations at any point (Fig. 6a, c; P > 0.10). Within heifers carrying male fetuses, at 19 days before conception maternal NEFA concentrations were reduced in LPeri compared with HPeri heifers (Fig. 6b, d; P = 0.02). At 23 dpc, NEFA concentrations tended to be greater in LPeri heifers (P = 0.09).
Plasma urea
Circulating maternal plasma urea concentrations reflected the protein level of the maternal diet throughout the PERI and POST periods (Fig. 7). Overall, urea concentrations were reduced in the LPeri compared with HPeri group at –19, 23 and 36 dpc (all P < 0.05). At 36 dpc, the POST diet also affected plasma urea concentrations, which were reduced in LPost compared with HPost heifers (P < 0.0001) following the start of the POST diet at 23 dpc. At 60 dpc, following the diet change at 23 dpc, urea concentrations varied with an interaction between the PERI and POST diets (Fig. 8; P = 0.0007). Heifers fed a low protein diet in both the PERI and POST periods (LL) had reduced plasma urea concentrations compared with heifers that changed diet from HPeri to LPost (HL). Both LL and HL groups had lower plasma urea concentrations (P < 0.05) than heifers fed the HPost diet (the LH and HH groups), which did not differ (P > 1.0). At 95 dpc, the PERI diet no longer affected plasma urea concentrations (P > 0.10), but plasma urea concentrations were lower in LPost than HPost heifers (P < 0.05). Overall, plasma urea concentrations did not differ between heifers carrying male and female fetuses at any point (P < 0.05).
![]() |
![]() |
When analysed separately, within heifers carrying females (Fig. 7a, c), urea concentrations were reduced in LPeri versus HPeri heifers at 19 days before conception and at 23 dpc (P < 0.05), and tended to be reduced at 60 dpc (P = 0.052). Urea was reduced in the LPost group at 36, 60 and 95 dpc (all P < 0.05). In heifers carrying males (Fig. 7b, d), maternal plasma urea concentrations were reduced in LPeri compared with HPeri heifers at 19 days before conception and at 23 and 36 dpc (all P < 0.05), and tended to be reduced at 60 dpc (P = 0.10). After the diet change at 23 dpc, the LPost diet was associated with reduced plasma urea concentrations at 36, 60 and 95 dpc (all P < 0.05), a tendency for reduced urea concentrations at 190 dpc (P = 0.08) and reduced urea concentrations at 256 dpc (P = 0.02) compared with the HPost diet.
Fetal ultrasound measures
Ultrasound measures of fetal development (AD, CNL, ED and UD) at 36, 60 and 95 dpc are presented in Table 2. Maternal diet affected ultrasonography measures of CRL at 36 dpc and BPD at 60 dpc in a sex-dependant manner, as reported previously (Copping et al. 2014). Due to increasing fetal size, ultrasonography measures of BPD at 95 dpc and CRL at 60 and 95 dpc could not be obtained.
At 60 dpc, AD and CNL did not differ according to maternal diet overall and within each sex (P > 0.10). Male fetuses had greater AD than females overall (1.73 ± 0.01 vs 1.68 ± 0.02 cm respectively; P = 0.048) and tended to have a larger CNL than females (P = 0.07). At 95 dpc, maternal diet and fetal sex did not affect ultrasound measures of CNL, ED or UD, either overall or within each sex (all P > 0.10).
Discussion
This paper reports conception results, maternal metabolic and endocrine patterns and early in utero fetal measures in a study exploring the effects of maternal protein diet during the periconception and first trimester period in beef heifers on fetoplacental development. Briefly, varying levels of maternal dietary protein during the periconception period (–60 to 23 dpc) and first trimester (24 to 98 dpc) of gestation in adolescent beef cattle resulted in maternal hormonal and metabolite adaptations associated with altered fetal development in the first trimester (Copping et al. 2014; Hernandez-Medrano et al. 2015). Intriguingly, many of these changes were sex specific, as reported previously in the bovine (Micke et al. 2015) and ovine (Jaquiery et al. 2012).
Heifer performance
The nutritional treatments altered heifer BW and ADG, with protein restriction during the PERI period resulting in small, but significant, differences in BW and ADG by 23 dpc. A similar pattern was observed in heifers fed the low-protein diet during the POST period. The effect of the low-protein diet extended beyond the PERI and POST diet periods, with increased ADG observed subsequent to ceasing nutritional treatments. This suggests that compensatory gain occurred when levels of protein in the diet met or exceeded requirements. This contrasts with the results of Sullivan et al. (2009b), who reported that depression of ADG continued until term following protein restriction during the first and second trimester. The study of Sullivan et al. (2009b) was undertaken in 2-year-old heifers, as distinct from the adolescent yearling heifers reported herein; furthermore, the dietary treatments used by Sullivan et al. (2009b) were not isocaloric and were applied at different stages of gestation (0–180 dpc). This may explain, in part, the difference in effects on ADG, because nutritional effects on maternal BW are affected by dam age (Hennessy et al. 2002). In addition, although maternal BW was affected by nutritional treatment, it was not associated with weight loss, ADG being positive throughout. This contrasts with many studies on maternal nutrient restriction in both sheep and cattle, where more severe dietary treatments are often associated with periods of maternal weight loss followed by realimentation (Long et al. 2009; Mossa et al. 2013). In the present study, heifers were fed individually throughout until term, as in the study of Sullivan et al. (2009b), to enable observation of effects in animals with limited ability for realimentation until term, a scenario that may commonly occur under range conditions.
Hormones and metabolites
It is well established that nutrition before joining may affect oocyte quality, fertilisation rates and subsequent embryo development in cattle (Dunne et al. 1999; Adamiak et al. 2005, 2006). Furthermore, changes in BW and nutritional status in beef heifers immediately following AI have been demonstrated to affect embryo quality and development as early as 6 days after insemination (Kruse et al. 2017) and to negatively affect pregnancy success to AI (Perry et al. 2016). Moreover, studies have reported that maternal nutrition during the periconception period has the potential to affect the critical window of DNA methylation, mediating changes in fetal development and postnatal progeny performance (Long et al. 2010; Micke et al. 2010; Taylor et al. 2018); however, the mechanisms are not fully defined. Given the effects of many metabolites and hormones on both embryo and fetoplacental development (Dupont et al. 2014), it is likely that diet-induced changes in maternal physiological and metabolic processes may contribute to these effects.
Overall, the pregnancy rate observed in the present study was lower than anticipated but similar to that reported following fixed-time AI (FTAI) programs in nulliparous heifers of B. indicus type that have undergone treatment to synchronise ovulation (36.3% vs 31–40%; Cavalieri et al. 2002; Butler et al. 2011a; Edwards et al. 2015). This relatively poor conception rate following FTAI has been reported to be associated with a high level of ovarian dysfunction (Butler et al. 2011b). Reduced oocyte developmental competence in adolescent heifers (as used in the present study) has also been reported in many animal species (Armstrong et al. 2001) and may be a contributing factor to the low conception rate. In the present study, the dietary protein levels were associated with maternal hormone and metabolite adaptions. Protein levels contained in the diet are positively associated with circulating plasma urea concentrations in ruminant species (Anthony et al. 1986; Butler et al. 1996; Swanson et al. 2015). The increased plasma urea concentrations in heifers fed the high-protein diet (HPeri and HPost) were therefore expected (Huntington and Archibeque 2000). The concentrations, although within an acceptable physiological range (2.8–8.8 mM; Gath et al. 2012), reached levels previously reported as associated with impaired fertility in dairy cows (Canfield et al. 1990; Elrod and Butler 1993; Butler et al. 1996; Larson et al. 1997). Although ovarian and/or uterine parameters and early embryo development were not directly measured in the present study, prior studies in beef heifers have shown that supplementation with dietary protein elevates plasma urea at the time of conception and in early gestation but has no effect on embryo survival (Kenny et al. 2002; Gath et al. 2012) or conception rate (Amundson et al. 2016). Kenny et al. (2002) concluded that the previously reported adverse effects of urea on early oocyte development in lactating dairy cattle may result from the interaction of negative energy balance and excess dietary protein intake rather than a direct outcome of elevated blood urea concentrations, whereas Gath et al. (2012) hypothesised that the negative effects of urea may occur earlier during oocyte development in the ovary rather than during fertilisation or after fertilisation. Similarly, in the present study, the elevated urea concentration in heifers receiving the high-protein diet was not associated with reduced conception rate or embryo survival.
In contrast, the effect of low maternal plasma urea concentrations on embryo survival is less clear, but has also been linked to poor fertility in dairy cattle (Wathes et al. 2007). Embryo loss between 23 and 36 dpc in the LPeri heifers tended to be increased, with plasma urea concentrations in this group below 4.5 mM, a physiological level previously defined as low by Wathes et al. (2007). The low plasma urea concentration may be due to the protein-deficient diet providing fewer precursors for nitrogen supply (Moore and Varga 1996). This decrease in plasma urea concentration occurred in combination with reduced progesterone, the effect of which is discussed below.
Elevated plasma NEFA concentrations in cattle are often associated with negative energy balance and are inversely related to ADG and nutrient intake (Konigsson et al. 2008). Based on their ADG, the heifers in the present study were in a positive energy status regardless of treatment. Therefore, the reduced NEFA concentrations at –19 dpc and the tendency for reduced NEFA concentrations at 36 dpc in heifers receiving the low-protein diet were unexpected because NEFA concentrations in beef cattle have been reported to be similar when managed to achieve different, but positive, ADG (Ellenberger et al. 1989; Bossis et al. 2000). There is an energetic cost associated with protein metabolism of diets with increased CP levels (Hales et al. 2013), and this may have mildly altered the energy balance in the heifers receiving the high-protein diet. However, the NEFA concentrations reported, although different at several points during the periods of maternal dietary treatment, were relatively low and within the normal physiological range (<0.5 mM; Wathes et al. 2007). The subsequent overall pattern of elevation of NEFA concentrations observed in late gestation in the heifers taken to term was as expected (Adewuyi et al. 2005).
Leptin concentrations are modulated by body fatness and nutrient intake (Houseknecht et al. 1988). The reduction in plasma leptin concentrations observed in the heifers receiving the low-protein diet was therefore anticipated. The increase in leptin concentrations at 95 dpc in male-carrying LPeri heifers was unexpected, but may be an artefact of the higher ADG also seen in the LPeri heifers during the POST maternal diet period. The previously reported sex-specific effect of low dietary protein lowering maternal leptin concentrations at preterm in heifers carrying male fetuses was not observed (Micke et al. 2015); however, heifers in that study exhibited a carryover effect of maternal diet, with depressed ADG in the third trimester after dietary protein restriction that was not a characteristic of the present study. Collectively, the measures of circulating urea and NEFA, as well as leptin, indicate that the experimental design was sufficient to alter the metabolic status of the heifers during critical periods of the dietary intervention despite the heifers being in positive weight gain throughout.
Hormones modulate maternal and fetal metabolism and nutrient transport in the placenta (Fowden and Forhead 2009; Sullivan et al. 2009b). Indeed, the placenta acts as an endocrine organ, regulating fetal growth and maternal metabolism (Sullivan et al. 2009a; Tarrade et al. 2015). Reduced maternal dietary protein in the periconception period and first trimester (LPeri and LPost) did not affect placental function, as indicated by bPL and bPAG concentrations (Breukelman et al. 2005). This is contrary to previous studies (Sullivan et al. 2009b; Micke et al. 2015) that showed that bPAG was increased in heifers fed low dietary protein in the first trimester, with these authors ascribing this to hormonal signalling by the fetus for enhanced nutrient requirements. This may reflect the difference in the timing of the nutritional treatments between studies.
Progesterone has a pivotal role in the establishment and maintenance of pregnancy (Lonergan 2011; Lonergan and Forde 2015) through modulation of the luteolytic signal, regulation of conceptus growth and interferon-τ production (Chagas e Silva and Lopes da Costa 2005). Circulating progesterone concentrations depend on the quality of the corpus luteum and may be affected by dietary intake and energy balance (O’Callaghan and Boland 1999). Inadequate circulating progesterone has been associated with increased levels of early embryo loss (Lonergan 2011; Lonergan et al. 2016). In the present study, protein restriction during the periconception period was associated with lower circulating progesterone concentrations at several critical time points. In heifers carrying a female fetus, progesterone measures at 23 dpc (following implantation; Wathes and Wooding 1980) were reduced by the LPeri diet. This may have contributed to the trend for increased embryo losses in the LPeri cohort at 36 dpc. However, the sampling regimen used did not allow us to fully explore the relationship between maternal progesterone concentrations and embryo loss.
Previous studies showed that the maternal IGF system has a role in modulating placental growth and development (Sferruzzi-Perri et al. 2006, 2011) and is positively associated with conception rate in cattle (Butler et al. 2006). Moreover, the maternal IGF system itself has been reported to be modulated by factors including endocrine signals (Guilbault et al. 1988; Bertolini et al. 2006; Weber et al. 2007), energy intake and dietary protein (Perry et al. 2002; Sullivan et al. 2009a). The decline in maternal circulating IGF1 concentrations throughout pregnancy is generally in agreement with previously reported profiles (Hossner et al. 1997; Sullivan et al. 2009a). The association of maternal dietary protein restriction with increases in maternal IGF1 concentrations in the first trimester is contrary to our previous study (Sullivan et al. 2009a), which showed a consistent positive circulating IGF1 response to increased maternal dietary protein. However, the diet in that study was not isocaloric and the response may have been modulated, in part, by the energy level of the diet (Elsasser et al. 1989). The response in the present study is small and not consistent, and limited to heifers carrying male fetuses; the sex of the fetus was not explored individually in analyses reported by previous studies.
A reduction in maternal IGF2 at 36 dpc in the LPeri cohort carrying female, but not male, fetuses may indicate a reduced rate of growth in these embryos, as evidenced by their reduced CRL (Copping et al. 2014), because exogenous IGF2 is known to induce enhanced fetal growth in the guinea pig (Sferruzzi-Perri et al. 2007). The maternal IGF2 response to protein restriction is in agreement with the results of Perry et al. (2002), who reported that maternal IGF2 in the second trimester was reduced by maternal dietary protein restriction in B. taurus yearling heifers, whereas others have reported that IGF2 is not sensitive to nutrition in cattle (McGuire et al. 1992).
Sex differences
It has been established that differences exist in growth rate, metabolism and gene expression levels between female and male embryos (Gutiérrez-Adán et al. 2006). Consequently, it has been suggested that environmental conditions may affect embryos differently depending on their sex (Bermejo-Alvarez et al. 2011; Tríbulo et al. 2018) and that bovine male blastocysts may be more responsive before implantation than female blastocysts under stress conditions (Gutiérrez-Adán et al. 2006). Similarly, the placenta may differ between sexes in its ability to respond and adapt to adverse environmental conditions (Rosenfeld 2015). When metabolic, hormonal and fetal development parameters were explored separately by fetal sex in the present study, some of the nutritional treatment effects were dependent upon the sex of the conceptus. In the LPeri cohort, heifers carrying female fetuses had reduced progesterone concentrations at 23 dpc. This period is immediately after embryo elongation, when adequate progesterone concentrations are essential (Clemente et al. 2009), with full adhesion of the embryo occurring by 35 dpc (Senger 2005). This effect of diet on progesterone concentrations was not observed in the heifers carrying male fetuses. In addition, despite no sex-related differences in circulating plasma urea and NEFA, leptin concentrations were reduced only in the cohort carrying female, but not male, fetuses at this point in pregnancy. Interestingly, at 36 dpc as previously reported CRL was decreased by the LPeri diet (Copping et al. 2014). When each sex was considered separately, this was observed only in the female fetuses, concomitant with the reduction in maternal IGF2 concentrations. Conversely, by 60 dpc as previously reported there was a sex-specific growth effect, with male fetuses showing a greater reduction in BPD measures from the LPeri diet than female fetuses (Copping et al. 2014). Furthermore, a significant correlation between bPL and IGF1 at 128 (r = 0.54) and 190 dpc (r = 0.62) occurred only in heifers carrying a female fetus, and may suggest a mechanism whereby the female fetus was signalling enhanced requirement for growth (Bertolini et al. 2006) after ceasing dietary restriction. Collectively, these findings suggest that the differential patterns in endocrine and metabolite status observed in these heifers in response to dietary treatments may have occurred in association with modifications in the early growth of their fetus in a sex-specific manner.
Conclusion
Prior studies in cattle have shown that nutrient restriction in early to mid pregnancy alters fetal growth in young cows, occurring both with (Long et al. 2009; Taylor et al. 2018) and without (Micke et al. 2010) maternal bodyweight loss. This study demonstrates that moderate protein restriction, typical of that observed in northern Australian rangeland pastures, during the periconception period (from –60 to 23 dpc) and first trimester (24–98 dpc) in growing adolescent beef cattle (with a positive ADG) is also detrimental to early fetal growth and may contribute to early embryo loss, the periconception dietary intervention being earlier than in previous studies. Maternal metabolic and endocrine hormone concentrations were responsive to protein restriction at critical developmental time points in a sex-specific manner. This study therefore builds on previously published work examining the mechanisms underlying fetal growth in cattle. Further, this study emphasises the difference between sexes in their ability to respond to maternal dietary perturbation at different time points during gestation. In extensive pasture-based production systems, where variation in feed quality through breeding and gestation commonly occur, quantification of the effects of nutrition on long-term productivity of the progeny remains an important area of study.
Conflict of interest
The authors declare that there is no conflict of interest that could be perceived as prejudicing the impartiality of the research reported.
Acknowledgements
This research was funded by Australian Research Council linkage grant (Project ID: LP110100649) with S. Kidman & Co and Ridley AgriProducts to V. E. A. Perry, R. J. Rodgers and I. C. McMillen. K. J. Copping was funded by an Australian Postgraduate award. R. J. Rodgers is funded by the National Health and Medical Research Council of Australia. The authors acknowledge the excellent technical assistance provided by Katja Hummitzsch, Wendy Bonner and Nicholas Hatzirodos, along with the statistical support provided by Data Management and Analysis Centre, The University of Adelaide. The authors are grateful for the assistance of Ray Cranney, Mark Irrgang (deceased), Maddie Jonas and staff at Tungali Feedlot in all animal husbandry procedures. The authors also gratefully acknowledge the assistance of Dominique Blache and Margaret Blackberry (The University of Western Australia) and Jon Green (University of Missouri) for performing hormone analyses.
References
Adamiak, S. J., Mackie, K., Watt, R. G., Webb, R., and Sinclair, K. D. (2005). Impact of nutrition on oocyte quality: cumulative effects of body composition and diet leading to hyperinsulinemia in cattle. Biol. Reprod. 73, 918–926.| Impact of nutrition on oocyte quality: cumulative effects of body composition and diet leading to hyperinsulinemia in cattle.Crossref | GoogleScholarGoogle Scholar | 15972884PubMed |
Adamiak, S. J., Powell, K., Rooke, J. A., Webb, R., and Sinclair, K. D. (2006). Body composition, dietary carbohydrates and fatty acids determine post-fertilisation development of bovine oocytes in vitro. Reproduction 131, 247–258.
| Body composition, dietary carbohydrates and fatty acids determine post-fertilisation development of bovine oocytes in vitro.Crossref | GoogleScholarGoogle Scholar | 16452718PubMed |
Adewuyi, A. A., Gruys, E., and van Eerdenburg, F. J. (2005). Non esterified fatty acids (NEFA) in dairy cattle. A review. Vet. Q. 27, 117–126.
| Non esterified fatty acids (NEFA) in dairy cattle. A review.Crossref | GoogleScholarGoogle Scholar | 16238111PubMed |
Amundson, O. L., Larimore, E. L., McNeel, A. K., Chase, C. C., Cushman, R. A., Freetly, H. C., and Perry, G. A. (2016). Uterine environment and pregnancy rate of heifers with elevated plasma urea nitrogen. Anim. Reprod. Sci. 173, 56–62.
| Uterine environment and pregnancy rate of heifers with elevated plasma urea nitrogen.Crossref | GoogleScholarGoogle Scholar | 27609357PubMed |
Anthony, R. V., Bellows, R. A., Short, R. E., Staigmiller, R. B., Kaltenbach, C. C., and Dunn, T. G. (1986). Fetal growth of beef calves. I. Effect of prepartum dietary crude protein on birth weight, blood metabolites and steroid hormone concentrations. J. Anim. Sci. 62, 1363–1374.
| Fetal growth of beef calves. I. Effect of prepartum dietary crude protein on birth weight, blood metabolites and steroid hormone concentrations.Crossref | GoogleScholarGoogle Scholar | 3722022PubMed |
Armstrong, D. G., McEvoy, T. G., Baxter, G., Robinson, J. J., Hogg, C. O., Woad, K. J., Webb, R., and Sinclair, K. D. (2001). Effect of dietary energy and protein on bovine follicular dynamics and embryo production in vitro: associations with the ovarian insulin-like growth factor system. Biol. Reprod. 64, 1624–1632.
| Effect of dietary energy and protein on bovine follicular dynamics and embryo production in vitro: associations with the ovarian insulin-like growth factor system.Crossref | GoogleScholarGoogle Scholar | 11369588PubMed |
Bermejo-Alvarez, P., Rizos, D., Lonergan, P., and Gutiérrez-Adán, A. (2011). Transcriptional sexual dimorphism in elongating bovine embryos: implications for XCI and sex determination genes. Reproduction 141, 801–808.
| Transcriptional sexual dimorphism in elongating bovine embryos: implications for XCI and sex determination genes.Crossref | GoogleScholarGoogle Scholar | 21411694PubMed |
Bertolini, M., Wallace, C. R., and Anderson, G. B. (2006). Expression profile and protein levels of placental products as indirect measures of placental function in in vitro-derived bovine pregnancies. Reproduction 131, 163–173.
| Expression profile and protein levels of placental products as indirect measures of placental function in in vitro-derived bovine pregnancies.Crossref | GoogleScholarGoogle Scholar | 16388019PubMed |
Blache, D., Tellam, R. L., Chagas, L. M., Blackberry, M. A., Vercoe, P. E., and Martin, G. B. (2000). Level of nutrition affects leptin concentrations in plasma and cerebrospinal fluid in sheep. J. Endocrinol. 165, 625–637.
| Level of nutrition affects leptin concentrations in plasma and cerebrospinal fluid in sheep.Crossref | GoogleScholarGoogle Scholar | 10828846PubMed |
Bossis, I., Wettemann, R. P., Welty, S. D., Vizcarra, J., and Spicer, L. J. (2000). Nutritionally induced anovulation in beef Heifers: ovarian and endocrine function during realimentation and resumption of ovulation. Biol. Reprod. 62, 1436–1444.
| Nutritionally induced anovulation in beef Heifers: ovarian and endocrine function during realimentation and resumption of ovulation.Crossref | GoogleScholarGoogle Scholar | 10775198PubMed |
Breier, B. H., Gallaher, B. W., and Gluckman, P. D. (1991). Radioimmunoassay for insulin-like growth factor-1: solutions to some potential problems and pitfalls. J. Endocrinol. 128, 347–357.
| Radioimmunoassay for insulin-like growth factor-1: solutions to some potential problems and pitfalls.Crossref | GoogleScholarGoogle Scholar | 1707433PubMed |
Breukelman, S. P., Perenyi, Z., Ruigh, L., van Wagtendonk-de Leeuw, A. M., Jonker, F. H., Vernooij, J. C., Beckers, J. F., van der Weijden, G. C., Vos, P. L., Dieleman, S. J., and Taverne, M. A. (2005). Plasma concentrations of bovine pregnancy-associated glycoprotein (bPAG) do not differ during the first 119 days between ongoing pregnancies derived by transfer of in vivo and in vitro produced embryos. Theriogenology 63, 1378–1389.
| Plasma concentrations of bovine pregnancy-associated glycoprotein (bPAG) do not differ during the first 119 days between ongoing pregnancies derived by transfer of in vivo and in vitro produced embryos.Crossref | GoogleScholarGoogle Scholar | 15725445PubMed |
Burns, B. M., Fordyce, G., and Holroyd, R. G. (2010). A review of factors that impact on the capacity of beef cattle females to conceive, maintain a pregnancy and wean a calf – implications for reproductive efficiency in northern Australia. Anim. Reprod. Sci. 122, 1–22.
| A review of factors that impact on the capacity of beef cattle females to conceive, maintain a pregnancy and wean a calf – implications for reproductive efficiency in northern Australia.Crossref | GoogleScholarGoogle Scholar | 20447780PubMed |
Butler, W. R., Calaman, J. J., and Beam, S. W. (1996). Plasma and milk urea nitrogen in relation to pregnancy rate in lactating dairy cattle. J. Anim. Sci. 74, 858–865.
| Plasma and milk urea nitrogen in relation to pregnancy rate in lactating dairy cattle.Crossref | GoogleScholarGoogle Scholar | 8728008PubMed |
Butler, S. T., Pelton, S. H., and Butler, W. R. (2006). Energy balance, metabolic status, and the first postpartum ovarian follicle wave in cows administered propylene glycol. J. Dairy Sci. 89, 2938–2951.
| Energy balance, metabolic status, and the first postpartum ovarian follicle wave in cows administered propylene glycol.Crossref | GoogleScholarGoogle Scholar | 16840609PubMed |
Butler, S. A., Atkinson, P. C., Boe-Hansen, G. B., Burns, B. M., Dawson, K., Bo, G. A., and McGowan, M. R. (2011a). Pregnancy rates after fixed-time artificial insemination of Brahman heifers treated to synchronize ovulation with low-dose intravaginal progesterone releasing devices, with or without eCG. Theriogenology 76, 1416–1423.
| Pregnancy rates after fixed-time artificial insemination of Brahman heifers treated to synchronize ovulation with low-dose intravaginal progesterone releasing devices, with or without eCG.Crossref | GoogleScholarGoogle Scholar | 21798584PubMed |
Butler, S. A., Phillips, N. J., Boe-Hansen, G. B., Bo, G. A., Burns, B. M., Dawson, K., and McGowan, M. R. (2011b). Ovarian responses in Bos indicus heifers treated to synchronise ovulation with intravaginal progesterone releasing devices, oestradiol benzoate, prostaglandin F(2alpha) and equine chorionic gonadotrophin. Anim Reprod Sci 129, 118–126.
| Ovarian responses in Bos indicus heifers treated to synchronise ovulation with intravaginal progesterone releasing devices, oestradiol benzoate, prostaglandin F(2alpha) and equine chorionic gonadotrophin.Crossref | GoogleScholarGoogle Scholar | 22136725PubMed |
Canfield, R. W., Sniffen, C. J., and Butler, W. R. (1990). Effects of excess degradable protein on postpartum reproduction and energy balance in dairy cattle. J. Dairy Sci. 73, 2342–2349.
| Effects of excess degradable protein on postpartum reproduction and energy balance in dairy cattle.Crossref | GoogleScholarGoogle Scholar | 2258483PubMed |
Cavalieri, J., Coleman, C., Rodrigues, H., Macmillan, K. L., and Fitzpatrick, L. A. (2002). The effect of timing of administration of oestradiol benzoate on characteristics of oestrus, timing of ovulation and fertility in Bos indicus heifers synchronised with a progesterone releasing intravaginal insert. Aust. Vet. J. 80, 217–223.
| The effect of timing of administration of oestradiol benzoate on characteristics of oestrus, timing of ovulation and fertility in Bos indicus heifers synchronised with a progesterone releasing intravaginal insert.Crossref | GoogleScholarGoogle Scholar | 12054285PubMed |
Chagas e Silva, J., and Lopes da Costa, L. (2005). Luteotrophic influence of early bovine embryos and the relationship between plasma progesterone concentrations and embryo survival. Theriogenology 64, 49–60.
| Luteotrophic influence of early bovine embryos and the relationship between plasma progesterone concentrations and embryo survival.Crossref | GoogleScholarGoogle Scholar | 15935842PubMed |
Clemente, M., de La Fuente, J., Fair, T., Al Naib, A., Gutiérrez-Adán, A., Roche, J. F., Rizos, D., and Lonergan, P. (2009). Progesterone and conceptus elongation in cattle: a direct effect on the embryo or an indirect effect via the endometrium? Reproduction 138, 507–517.
| Progesterone and conceptus elongation in cattle: a direct effect on the embryo or an indirect effect via the endometrium?Crossref | GoogleScholarGoogle Scholar | 19556439PubMed |
Copping, K. J., Hoare, A., Callaghan, M., McMillen, I. C., Rodgers, R. J., and Perry, V. E. A. (2014). Fetal programming in 2-year-old calving heifers: peri-conception and first trimester protein restriction alters fetal growth in a gender-specific manner. Anim. Prod. Sci. 54, 1333–1337.
| Fetal programming in 2-year-old calving heifers: peri-conception and first trimester protein restriction alters fetal growth in a gender-specific manner.Crossref | GoogleScholarGoogle Scholar |
Copping, K. J., Ruiz-Diaz, M. D., Rutland, C. S., Mongan, N. P., Callaghan, M. J., McMillen, I. C., Rodgers, R. J., and Perry, V. E. A. (2017). Peri-conception and first trimester diet modifies reproductive development in bulls. Reprod. Fertil. Dev. 30, 703–720.
| Peri-conception and first trimester diet modifies reproductive development in bulls.Crossref | GoogleScholarGoogle Scholar |
Copping, K. J., Hernandez-Medrano, J., Hoare, A., Hummitzsch, K., McMillen, I. C., Morrison, J. L., Rodgers, R. J., and Perry, V. E. A. (2020). Maternal periconceptional and first trimester protein restriction in beef heifers: effects on placental parameters and fetal and neonatal calf development. Reprod. Fertil. Dev. 32, 495–507.
| Maternal periconceptional and first trimester protein restriction in beef heifers: effects on placental parameters and fetal and neonatal calf development.Crossref | GoogleScholarGoogle Scholar | 32029064PubMed |
Dunne, L. D., Diskin, M. G., Boland, M. P., O’Farrell, K. J., and Sreenan, J. M. (1999). The effect of pre- and post-insemination plane of nutrition on embryo survival in beef heifers. Anim. Sci. 69, 411–417.
| The effect of pre- and post-insemination plane of nutrition on embryo survival in beef heifers.Crossref | GoogleScholarGoogle Scholar |
Dupont, J., Scaramuzzi, R. J., and Reverchon, M. (2014). The effect of nutrition and metabolic status on the development of follicles, oocytes and embryos in ruminants. Animal 8, 1031–1044.
| The effect of nutrition and metabolic status on the development of follicles, oocytes and embryos in ruminants.Crossref | GoogleScholarGoogle Scholar | 24774511PubMed |
Edwards, L. J., and McMillen, I. C. (2002). Impact of maternal undernutrition during the periconceptional period, fetal number, and fetal sex on the development of the hypothalamo–pituitary adrenal axis in sheep during late gestation. Biol. Reprod. 66, 1562–1569.
| Impact of maternal undernutrition during the periconceptional period, fetal number, and fetal sex on the development of the hypothalamo–pituitary adrenal axis in sheep during late gestation.Crossref | GoogleScholarGoogle Scholar | 11967224PubMed |
Edwards, S. A., Bo, G. A., Chandra, K. A., Atkinson, P. C., and McGowan, M. R. (2015). Comparison of the pregnancy rates and costs per calf born after fixed-time artificial insemination or artificial insemination after estrus detection in Bos indicus heifers. Theriogenology 83, 114–120.
| Comparison of the pregnancy rates and costs per calf born after fixed-time artificial insemination or artificial insemination after estrus detection in Bos indicus heifers.Crossref | GoogleScholarGoogle Scholar | 25284281PubMed |
Ellenberger, M. A., Johnson, D. E., Carstens, G. E., Hossner, K. L., Holland, M. D., Nett, T. M., and Nockels, C. F. (1989). Endocrine and metabolic changes during altered growth rates in beef cattle. J. Anim. Sci. 67, 1446–1454.
| Endocrine and metabolic changes during altered growth rates in beef cattle.Crossref | GoogleScholarGoogle Scholar | 2670867PubMed |
Elrod, C. C., and Butler, W. R. (1993). Reduction of fertility and alteration of uterine pH in heifers fed excess ruminally degradable protein. J. Anim. Sci. 71, 694–701.
| Reduction of fertility and alteration of uterine pH in heifers fed excess ruminally degradable protein.Crossref | GoogleScholarGoogle Scholar | 8463156PubMed |
Elsasser, T. H., Rumsey, T. S., and Hammond, A. C. (1989). Influence of diet on basal and growth hormone-stimulated plasma concentrations of IGF-I in beef cattle. J. Anim. Sci. 67, 128–141.
| Influence of diet on basal and growth hormone-stimulated plasma concentrations of IGF-I in beef cattle.Crossref | GoogleScholarGoogle Scholar | 2925537PubMed |
Forhead, A. J., Jellyman, J. K., Gillham, K., Ward, J. W., Blache, D., and Fowden, A. L. (2011). Renal growth retardation following angiotensin II type 1 (AT(1)) receptor antagonism is associated with increased AT(2) receptor protein in fetal sheep. J. Endocrinol. 208, 137–145.
| Renal growth retardation following angiotensin II type 1 (AT(1)) receptor antagonism is associated with increased AT(2) receptor protein in fetal sheep.Crossref | GoogleScholarGoogle Scholar | 21097994PubMed |
Fowden, A. L., and Forhead, A. J. (2009). Hormones as epigenetic signals in developmental programming. Exp. Physiol. 94, 607–625.
| Hormones as epigenetic signals in developmental programming.Crossref | GoogleScholarGoogle Scholar | 19251980PubMed |
Gath, V. P., Crowe, M. A., O’Callaghan, D., Boland, M. P., Duffy, P., Lonergan, P., and Mulligan, F. J. (2012). Effects of diet type on establishment of pregnancy and embryo development in beef heifers. Anim. Reprod. Sci. 133, 139–145.
| Effects of diet type on establishment of pregnancy and embryo development in beef heifers.Crossref | GoogleScholarGoogle Scholar | 22818782PubMed |
Green, J. A., Parks, T. E., Avalle, M. P., Telugu, B. P., McLain, A. L., Peterson, A. J., McMillan, W., Mathialagan, N., Hook, R. R., Xie, S., and Roberts, R. M. (2005). The establishment of an ELISA for the detection of pregnancy-associated glycoproteins (PAGs) in the serum of pregnant cows and heifers. Theriogenology 63, 1481–1503.
| The establishment of an ELISA for the detection of pregnancy-associated glycoproteins (PAGs) in the serum of pregnant cows and heifers.Crossref | GoogleScholarGoogle Scholar | 15725453PubMed |
Guilbault, L. A., Beckers, J. F., Roy, G. L., and Grasso, F. (1988). Plasma concentrations of bovine placental lactogen, prolactin and prostaglandins during the periparturient period in Ayrshire heifers bearing different breeds of fetus. Theriogenology 29, 255.
| Plasma concentrations of bovine placental lactogen, prolactin and prostaglandins during the periparturient period in Ayrshire heifers bearing different breeds of fetus.Crossref | GoogleScholarGoogle Scholar |
Gunn, P. J., Schoonmaker, J. P., Lemenager, R. P., and Bridges, G. A. (2014). Feeding excess crude protein to gestating and lactating beef heifers: impact on parturition, milk composition, ovarian function, reproductive efficiency and pre-weaning progeny growth. Livest. Sci. 167, 435–448.
| Feeding excess crude protein to gestating and lactating beef heifers: impact on parturition, milk composition, ovarian function, reproductive efficiency and pre-weaning progeny growth.Crossref | GoogleScholarGoogle Scholar |
Gutiérrez-Adán, A., Perez-Crespo, M., Fernandez-Gonzalez, R., Ramirez, M. A., Moreira, P., Pintado, B., Lonergan, P., and Rizos, D. (2006). Developmental consequences of sexual dimorphism during pre-implantation embryonic development. Reprod. Domest. Anim. 41, 54–62.
| Developmental consequences of sexual dimorphism during pre-implantation embryonic development.Crossref | GoogleScholarGoogle Scholar | 16984469PubMed |
Hales, K. E., Cole, N. A., and MacDonald, J. C. (2013). Effects of increasing concentrations of wet distillers grains with solubles in steam-flaked, corn-based diets on energy metabolism, carbon-nitrogen balance, and methane emissions of cattle. J. Anim. Sci. 91, 819–828.
| Effects of increasing concentrations of wet distillers grains with solubles in steam-flaked, corn-based diets on energy metabolism, carbon-nitrogen balance, and methane emissions of cattle.Crossref | GoogleScholarGoogle Scholar | 23148244PubMed |
Hennessy, D. W., Hearnshaw, H., Greenwood, P. L., Harper, G. S., and Morris, S. G. (2002). The effects of low or high quality pastures on the live weight of cows at calving and on birth weight of calves sired by Wagyu or Piedmontese. Animal Production in Australia 24, 311.
Hernandez-Medrano, J. H., Copping, K. J., Hoare, A., Wapanaar, W., Grivell, R., Kuchel, T., Miguel-Pacheco, G., McMillen, I. C., Rodgers, R. J., and Perry, V. E. (2015). Gestational dietary protein is associated with sex specific decrease in blood flow, fetal heart growth and post-natal blood pressure of progeny. PLoS One 10, e0125694.
| Gestational dietary protein is associated with sex specific decrease in blood flow, fetal heart growth and post-natal blood pressure of progeny.Crossref | GoogleScholarGoogle Scholar | 25915506PubMed |
Hossner, K. L., Holland, M. D., Williams, S. E., Wallace, C. R., Niswender, G. D., and Odde, K. G. (1997). Serum concentrations of insulin-like growth factors and placental lactogen during gestation in cattle. II. Maternal profiles. Domest. Anim. Endocrinol. 14, 316–324.
| Serum concentrations of insulin-like growth factors and placental lactogen during gestation in cattle. II. Maternal profiles.Crossref | GoogleScholarGoogle Scholar | 9347252PubMed |
Houseknecht, K. L., Boggs, D., Campion, D., Sartin, J., Kiser, T., Rampacek, G., and Amos, H. (1988). Effect of dietary energy source and level on serum growth hormone, insulin-like growth factor-I, growth and body composition in beef heifers. J. Anim. Sci. 66, 2916–2923.
| Effect of dietary energy source and level on serum growth hormone, insulin-like growth factor-I, growth and body composition in beef heifers.Crossref | GoogleScholarGoogle Scholar | 2852186PubMed |
Huntington, G. B., and Archibeque, S. L. (2000). Practical aspects of urea and ammonia metabolism in ruminants. Journal of Animal Science 77, 1–11.
| Practical aspects of urea and ammonia metabolism in ruminants.Crossref | GoogleScholarGoogle Scholar |
Jaquiery, A. L., Oliver, M. H., Honeyfield-Ross, M., Harding, J. E., and Bloomfield, F. H. (2012). Periconceptional undernutrition in sheep affects adult phenotype only in males. J. Nutr. Metab. 2012, 123610.
| Periconceptional undernutrition in sheep affects adult phenotype only in males.Crossref | GoogleScholarGoogle Scholar | 23091706PubMed |
Kenny, D. A., Boland, M. P., Diskin, M. G., and Sreenan, J. M. (2002). Effect of rumen degradable protein with or without fermentable carbohydrate supplementation on blood metabolites and embryo survival in cattle. Anim. Sci. 74, 529–537.
| Effect of rumen degradable protein with or without fermentable carbohydrate supplementation on blood metabolites and embryo survival in cattle.Crossref | GoogleScholarGoogle Scholar |
Konigsson, K., Savoini, G., Govoni, N., Invernizzi, G., Prandi, A., Kindahl, H., and Veronesi, M. C. (2008). Energy balance, leptin, NEFA and IGF-I plasma concentrations and resumption of post partum ovarian activity in Swedish Red and White breed cows. Acta Vet. Scand. 50, 3.
| Energy balance, leptin, NEFA and IGF-I plasma concentrations and resumption of post partum ovarian activity in Swedish Red and White breed cows.Crossref | GoogleScholarGoogle Scholar | 18184427PubMed |
Kruse, S. G., Bridges, G. A., Funnell, B. J., Bird, S. L., Lake, S. L., Arias, R. P., Amundson, O. L., Larimore, E. L., Keisler, D. H., and Perry, G. A. (2017). Influence of post-insemination nutrition on embryonic development in beef heifers. Theriogenology 90, 185–190.
| Influence of post-insemination nutrition on embryonic development in beef heifers.Crossref | GoogleScholarGoogle Scholar | 28166966PubMed |
Larson, S. F., Butler, W. R., and Currie, W. B. (1997). Reduced fertility associated with low progesterone postbreeding and increased milk urea nitrogen in lactating cows. J. Dairy Sci. 80, 1288–1295.
| Reduced fertility associated with low progesterone postbreeding and increased milk urea nitrogen in lactating cows.Crossref | GoogleScholarGoogle Scholar | 9241591PubMed |
Lonergan, P. (2011). Influence of progesterone on oocyte quality and embryo development in cows. Theriogenology 76, 1594–1601.
| Influence of progesterone on oocyte quality and embryo development in cows.Crossref | GoogleScholarGoogle Scholar | 21855985PubMed |
Lonergan, P., and Forde, N. (2015). The role of progesterone in maternal recognition of pregnancy in domestic ruminants. Adv. Anat. Embryol. Cell Biol. 216, 87–104.
| 26450496PubMed |
Lonergan, P., Forde, N., and Spencer, T. (2016). Role of progesterone in embryo development in cattle. Reprod. Fertil. Dev. 28, 66–74.
| Role of progesterone in embryo development in cattle.Crossref | GoogleScholarGoogle Scholar | 27062875PubMed |
Long, N. M., Vonnahme, K. A., Hess, B. W., Nathanielsz, P. W., and Ford, S. P. (2009). Effects of early gestational undernutrition on fetal growth, organ development, and placentomal composition in the bovine. J. Anim. Sci. 87, 1950–1959.
| Effects of early gestational undernutrition on fetal growth, organ development, and placentomal composition in the bovine.Crossref | GoogleScholarGoogle Scholar | 19213703PubMed |
Long, N. M., Prado-Cooper, M. J., Krehbiel, C. R., DeSilva, U., and Wettemann, R. P. (2010). Effects of nutrient restriction of bovine dams during early gestation on postnatal growth, carcass and organ characteristics, and gene expression in adipose tissue and muscle. J. Anim. Sci. 88, 3251–3261.
| Effects of nutrient restriction of bovine dams during early gestation on postnatal growth, carcass and organ characteristics, and gene expression in adipose tissue and muscle.Crossref | GoogleScholarGoogle Scholar | 20525929PubMed |
MacLaughlin, S. M., Walker, S. K., Roberts, C. T., Kleemann, D. O., and McMillen, I. C. (2005). Periconceptional nutrition and the relationship between maternal body weight changes in the periconceptional period and feto-placental growth in the sheep. J. Physiol. 565, 111–124.
| Periconceptional nutrition and the relationship between maternal body weight changes in the periconceptional period and feto-placental growth in the sheep.Crossref | GoogleScholarGoogle Scholar | 15774513PubMed |
McGuire, M. A., Vicini, J. L., Bauman, D. E., and Veenhuizen, J. J. (1992). Insulin-like growth factors and binding proteins in ruminants and their nutritional regulation. J. Anim. Sci. 70, 2901–2910.
| Insulin-like growth factors and binding proteins in ruminants and their nutritional regulation.Crossref | GoogleScholarGoogle Scholar | 1383181PubMed |
Micke, G. C., Sullivan, T. M., Soares Magalhaes, R. J., Rolls, P. J., Norman, S. T., and Perry, V. E. (2010). Heifer nutrition during early- and mid-pregnancy alters fetal growth trajectory and birth weight. Anim. Reprod. Sci. 117, 1–10.
| Heifer nutrition during early- and mid-pregnancy alters fetal growth trajectory and birth weight.Crossref | GoogleScholarGoogle Scholar | 19394770PubMed |
Micke, G. C., Sullivan, T. M., McMillen, I. C., Gentili, S., and Perry, V. E. (2011). Protein intake during gestation affects postnatal bovine skeletal muscle growth and relative expression of IGF1, IGF1R, IGF2 and IGF2R. Mol. Cell. Endocrinol. 332, 234–241.
| Protein intake during gestation affects postnatal bovine skeletal muscle growth and relative expression of IGF1, IGF1R, IGF2 and IGF2R.Crossref | GoogleScholarGoogle Scholar | 21056085PubMed |
Micke, G. C., Sullivan, T. M., Kennaway, D. J., Hernandez-Medrano, J., and Perry, V. E. (2015). Maternal endocrine adaptation throughout pregnancy to nutrient manipulation: consequences for sexually dimorphic programming of thyroid hormones and development of their progeny. Theriogenology 83, 604–615.
| Maternal endocrine adaptation throughout pregnancy to nutrient manipulation: consequences for sexually dimorphic programming of thyroid hormones and development of their progeny.Crossref | GoogleScholarGoogle Scholar | 25492373PubMed |
Moore, D. A., and Varga, G. (1996). BUN and MUN: urea nitrogen testing in dairy cattle. Compend. Contin. Educ. Vet. 18, 712–720.
Mossa, F., Carter, F., Walsh, S. W., Kenny, D. A., Smith, G. W., Ireland, J. L., Hildebrandt, T. B., Lonergan, P., Ireland, J. J., and Evans, A. C. (2013). Maternal undernutrition in cows impairs ovarian and cardiovascular systems in their offspring. Biol. Reprod. 88, 92.
| Maternal undernutrition in cows impairs ovarian and cardiovascular systems in their offspring.Crossref | GoogleScholarGoogle Scholar | 23426432PubMed |
National Health and Medical Research Council (NHMRC) (2004). ‘Australian Code for the Care and Use of Animals for Scientific Purposes.’ 7th edn. (NHMRC: Canberra.)
Norman, M. (1963). Dry season protein and energy supplements for beef cattle on native pastures at Katherine, N.T. Aust. J. Exp. Agric. 3, 280–283.
| Dry season protein and energy supplements for beef cattle on native pastures at Katherine, N.T.Crossref | GoogleScholarGoogle Scholar |
O’Callaghan, D., and Boland, M. P. (1999). Nutritional effects on ovulation, embryo development and the establishment of pregnancy in ruminants. Anim. Sci. 68, 299–314.
| Nutritional effects on ovulation, embryo development and the establishment of pregnancy in ruminants.Crossref | GoogleScholarGoogle Scholar |
Perry, V. E., Norman, S. T., Daniel, R. C., Owens, P. C., Grant, P., and Doogan, V. J. (2002). Insulin-like growth factor levels during pregnancy in the cow are affected by protein supplementation in the maternal diet. Anim. Reprod. Sci. 72, 1–10.
| Insulin-like growth factor levels during pregnancy in the cow are affected by protein supplementation in the maternal diet.Crossref | GoogleScholarGoogle Scholar | 12106961PubMed |
Perry, G. A., Perry, B. L., and Walker, J. A. (2016). Postinsemination diet change on reproductive performance in beef heifers. Prof. Anim. Sci. 32, 316–321.
| Postinsemination diet change on reproductive performance in beef heifers.Crossref | GoogleScholarGoogle Scholar |
Pohler, K. G., Pereira, M. H., Lopes, F. R., Lawrence, J. C., Keisler, D. H., Smith, M. F., Vasconcelos, J. L., and Green, J. A. (2016). Circulating concentrations of bovine pregnancy-associated glycoproteins and late embryonic mortality in lactating dairy herds. J. Dairy Sci. 99, 1584–1594.
| Circulating concentrations of bovine pregnancy-associated glycoproteins and late embryonic mortality in lactating dairy herds.Crossref | GoogleScholarGoogle Scholar | 26709163PubMed |
Rosenfeld, C. S. (2015). Sex-specific placental responses in fetal development. Endocrinology 156, 3422–3434.
| Sex-specific placental responses in fetal development.Crossref | GoogleScholarGoogle Scholar | 26241064PubMed |
Senger, P. L. (2005). ‘Pathways to Pregnancy and Parturition.’ 2nd edn. (Current Conceptions: Pullman.)
Sferruzzi-Perri, A. N., Owens, J. A., Pringle, K. G., Robinson, J. S., and Roberts, C. T. (2006). Maternal insulin-like growth factors-I and -II act via different pathways to promote fetal growth. Endocrinology 147, 3344–3355.
| Maternal insulin-like growth factors-I and -II act via different pathways to promote fetal growth.Crossref | GoogleScholarGoogle Scholar | 16556757PubMed |
Sferruzzi-Perri, A. N., Owens, J. A., Standen, P., Taylor, R. L., Heinemann, G. K., Robinson, J. S., and Roberts, C. T. (2007). Early treatment of the pregnant guinea pig with IGFs promotes placental transport and nutrient partitioning near term. Am. J. Physiol. Endocrinol. Metab. 292, E668–E676.
| Early treatment of the pregnant guinea pig with IGFs promotes placental transport and nutrient partitioning near term.Crossref | GoogleScholarGoogle Scholar | 17062842PubMed |
Sferruzzi-Perri, A. N., Owens, J. A., Pringle, K. G., and Roberts, C. T. (2011). The neglected role of insulin-like growth factors in the maternal circulation regulating fetal growth. J. Physiol. 589, 7–20.
| The neglected role of insulin-like growth factors in the maternal circulation regulating fetal growth.Crossref | GoogleScholarGoogle Scholar | 20921199PubMed |
Spencer, T. E., Johnson, G. A., Bazer, F. W., and Burghardt, R. C. (2007). Fetal–maternal interactions during the establishment of pregnancy in ruminants. Soc. Reprod. Fertil. Suppl. 64, 379–396.
| Fetal–maternal interactions during the establishment of pregnancy in ruminants.Crossref | GoogleScholarGoogle Scholar | 17491160PubMed |
Starbuck, M. J., Dailey, R. A., and Inskeep, E. K. (2004). Factors affecting retention of early pregnancy in dairy cattle. Anim. Reprod. Sci. 84, 27–39.
| Factors affecting retention of early pregnancy in dairy cattle.Crossref | GoogleScholarGoogle Scholar | 15302385PubMed |
Sullivan, T. M., Micke, G., Perkins, N., Martin, G., Wallace, C., Gatford, K., Owens, J., and Perry, V. (2009a). Dietary protein during gestation affects maternal IGF, IGFBP, leptin concentrations, and fetal growth in heifers. J. Anim. Sci. 87, 3304–3316.
| Dietary protein during gestation affects maternal IGF, IGFBP, leptin concentrations, and fetal growth in heifers.Crossref | GoogleScholarGoogle Scholar | 19617516PubMed |
Sullivan, T. M., Micke, G. C., Magalhaes, R. S., Martin, G. B., Wallace, C. R., Green, J. A., and Perry, V. E. (2009b). Dietary protein during gestation affects circulating indicators of placental function and fetal development in heifers. Placenta 30, 348–354.
| Dietary protein during gestation affects circulating indicators of placental function and fetal development in heifers.Crossref | GoogleScholarGoogle Scholar | 19233467PubMed |
Swanson, T. J., Lekatz, L. A., Van Emon, M. L., Perry, G. A., Schauer, C. S., Maddock Carlin, K. R., Hammer, C. J., and Vonnahme, K. A. (2015). Supplementation of metabolizable protein during late gestation and fetal number impact ewe organ mass, maternal serum hormone and metabolite concentrations, and conceptus measurements. Domest. Anim. Endocrinol. 58, 113–125.el.
| Supplementation of metabolizable protein during late gestation and fetal number impact ewe organ mass, maternal serum hormone and metabolite concentrations, and conceptus measurements.Crossref | GoogleScholarGoogle Scholar | 26416263PubMed |
Tarrade, A., Panchenko, P., Junien, C., and Gabory, A. (2015). Placental contribution to nutritional programming of health and diseases: epigenetics and sexual dimorphism. J. Exp. Biol. 218, 50–58.
| Placental contribution to nutritional programming of health and diseases: epigenetics and sexual dimorphism.Crossref | GoogleScholarGoogle Scholar | 25568451PubMed |
Taylor, R. K., LeMaster, C. T., Mangrum, K. S., Ricks, R. E., and Long, N. M. (2018). Effects of maternal nutrient restriction during early or mid-gestation without realimentation on maternal physiology and foetal growth and development in beef cattle. Animal 12, 312–321.
| Effects of maternal nutrient restriction during early or mid-gestation without realimentation on maternal physiology and foetal growth and development in beef cattle.Crossref | GoogleScholarGoogle Scholar | 28697817PubMed |
Tríbulo, P., Jumatayeva, G., Lehloenya, K., Moss, J. I., Negron-Perez, V. M., and Hansen, P. J. (2018). Effects of sex on response of the bovine preimplantation embryo to insulin-like growth factor 1, activin A, and WNT7A. BMC Dev. Biol. 18, 16.
| Effects of sex on response of the bovine preimplantation embryo to insulin-like growth factor 1, activin A, and WNT7A.Crossref | GoogleScholarGoogle Scholar | 30055575PubMed |
Wallace, C. R. (1993). Concentration of bovine placental lactogen in dairy and beef cows across gestation. Domest. Anim. Endocrinol. 10, 67–70.
| Concentration of bovine placental lactogen in dairy and beef cows across gestation.Crossref | GoogleScholarGoogle Scholar | 8467647PubMed |
Wathes, D. C., and Wooding, F. B. (1980). An electron microscopic study of implantation in the cow. Am. J. Anat. 159, 285–306.
| An electron microscopic study of implantation in the cow.Crossref | GoogleScholarGoogle Scholar | 7211711PubMed |
Wathes, D. C., Bourne, N., Cheng, Z., Mann, G. E., Taylor, V. J., and Coffey, M. P. (2007). Multiple correlation analyses of metabolic and endocrine profiles with fertility in primiparous and multiparous cows. J. Dairy Sci. 90, 1310–1325.
| Multiple correlation analyses of metabolic and endocrine profiles with fertility in primiparous and multiparous cows.Crossref | GoogleScholarGoogle Scholar | 17297107PubMed |
Weber, W. J., Wallace, C. R., Hansen, L. B., Chester-Jones, H., and Crooker, B. A. (2007). Effects of genetic selection for milk yield on somatotropin, insulin-like growth factor-I, and placental lactogen in Holstein cows. J. Dairy Sci. 90, 3314–3325.
| Effects of genetic selection for milk yield on somatotropin, insulin-like growth factor-I, and placental lactogen in Holstein cows.Crossref | GoogleScholarGoogle Scholar | 17582117PubMed |