A comprehensive review of South Australia’s Great Artesian Basin spring and discharge wetlands biota
P. G. Beasley-Hall
A
B
Abstract
The Great Artesian Basin (GAB) feeds thousands of springs in Australia’s arid centre, supporting relictual species not found elsewhere on Earth. Springs are considerably threatened by ongoing water abstraction by industry. Robust management plans are needed to prevent further extirpations of GAB taxa, but fundamental biodiversity knowledge is lacking.
We aimed to characterise major organismal groups in South Australian GAB springs and surrounding wetlands, their conservation and taxonomic status, and potential biodiversity hotspots and connectivity of spring ecosystems.
Focusing on South Australia as a case study, we conducted a comprehensive review of GAB spring biota based on the published scientific and grey literature.
Almost 500 taxa have been recorded from GAB springs, the majority being invertebrates. Community composition is highly heterogeneous among spring clusters and the true extent of spring biodiversity is far greater than currently known.
GAB springs have intrinsic value as refugia for both endemics and cosmopolitan taxa. GAB invertebrates are poorly conserved and largely lacking in taxonomic knowledge. We highlight several potential biodiversity hotspots that have been overlooked in the literature.
Fundamental biodiversity information on the GAB is crucial for decision making in conservation management, for industry, and for Traditional Custodians.
Keywords: aquifers, mound springs, biodiversity, biogeography, Great Artesian Basin, groundwater-dependent ecosystems, springs.
Introduction
The Great Artesian Basin (GAB) is Australia’s largest groundwater resource, spanning over one-fifth of the continent’s area or almost 2 × 106 km2 (Habermehl 2020). At the outer margins of the GAB where the confining layer is thin, pressurised water is often forced to the surface to form springs and associated wetlands. Thousands of such springs are found throughout Central Australia, with >5000 individual spring vents (discrete discharge points of water) in South Australia (hereafter SA) (Arabana Aboriginal Corporation 2021; WaterConnect, see https://www.waterconnect.sa.gov.au/Systems/GD/Pages/Default.aspx, accessed 20 April 2023), >2000 in Queensland (Queensland Department of Regional Development, Manufacturing and Water 2023) and >400 in New South Wales (New South Wales Department of Planning, Industry and Environment 2021). From an ecological and evolutionary standpoint, GAB-fed springs support wetlands representing ‘museums of biodiversity’ housing plant and animal species not found elsewhere on Earth (Murphy et al. 2015). As relicts of the continent’s mesic past, species endemic to GAB springs often have exceptionally small distributions. It is not uncommon for taxa to be restricted to a single cluster of springs, termed ‘ultra-short range endemics’ (e.g. Gotch et al. 2008; Guzik et al. 2012, 2019; Harvey 1989; King 2009; King and Leys 2014; Murphy et al. 2012, 2009).
The conservation and management of groundwater resources is lacking globally (Famiglietti 2014). Springs fed by the GAB are no exception and are considerably threatened by a range of industrial and pastoral practices (Mudd 2000; Fairfax and Fensham 2002; Lewis and Harris 2020). The sinking of more than 50,000 artificial boreholes and the direct abstraction of Basin water have led to substantial reductions in artesian pressure (hereafter ‘drawdown’) and spring flow (Mudd 2000; Gotch et al. 2016; Great Artesian Basin Coordinating Committee 2019; Beasley-Hall et al. 2023a). A complete cessation of flow has occurred for an estimated 800 springs across Australia (Andersen et al. 2016; Fensham et al. 2016), and declines of endemic fauna have been documented as a result of this degradation of spring habitats (Fensham et al. 2010). Springs and their biota also face threats in addition to drawdown, including grazing, trampling, and soiling of wetlands by livestock such as cattle (Fatchen 2000), overabundant pest species (Noack 1994; Kerezsy 2015), climate change (Ordens et al. 2020) and tourist activity (Witjira National Park Co-management Board 2022). Despite remediation efforts, drivers of spring flow remain poorly understood and net water flows in GAB springs appear to still be reducing in certain regions of the Basin (Green and Berens 2013).
The development of a biotic inventory has been identified as an urgent action to recover the community of native species dependent on GAB springs (Fensham et al. 2010). Publicly available data can help to inform future management plans and early warning systems that detect changes in these ecosystems of high biodiversity value (Vaughan et al. 2001; Brack et al. 2015; Obura et al. 2019) and so improving their accessibility is a clear priority. Further, industry stakeholders frequently rely on biotic inventories to ensure they are fulfilling their environmental obligations. This is particularly relevant for the GAB in the context of drawdown. Yet, basic taxonomic and biological information for these communities is still lacking, inaccessible or disparate, making it difficult to accurately assess and monitor all spring species in the face of rapid, human-driven change. The Queensland Government has published information on metrics such as water quality, chemistry, flow rate, biodiversity and spring condition (Queensland Government 2018), but such information is not public for SA and New South Wales. This lack of taxonomic information is particularly pronounced for endemic spring invertebrates (Rossini 2020). The relationship between environmental characteristics of springs and biodiversity is also not well understood (Harris 1992; Fensham et al. 2010; Green and Berens 2013; Rossini et al. 2018), meaning it is currently difficult to establish clear conservation priorities for GAB spring taxa in certain locations over others. The compilation of baseline information on these taxa in a centralised, accessible manner would represent a fundamental resource to facilitate future conservation work on this ecological community. Here, we conducted a literature review of species associated with springs in SA, selected due to its high number of GAB springs (>60% of all active springs) and the comparatively well-studied nature of its biodiversity (Zeidler and Ponder 1989; King 2009; Murphy et al. 2013, 2015). We specifically aimed to: (1) characterise major organismal groups in SA GAB springs and surrounding wetlands; (2) document the conservation and taxonomic status of these groups to highlight knowledge gaps; (3) identify hotspots of biodiversity in SA GAB springs; and (4) assess connectivity of SA GAB spring communities.
Materials and methods
Literature review and database construction
We undertook a non-systematic review of all available information relevant to the biodiversity of GAB springs in SA. A formal systematic searching strategy was not possible as a large proportion of information on springs is present in unpublished or grey literature such as government reports, internal publications from mining companies and museum records. We therefore relied heavily on repositories such as the Government of South Australia’s WaterConnect portal (see https://www.waterconnect.sa.gov.au, accessed 26 April 2023) and The Atlas of Living Australia (Belbin et al. 2021). We were specifically interested in data indicating the presence or absence of species in or around spring wetlands as well as their occurrence extents, if available, to gauge where taxa occurred and their degree of endemicity. The scope of this review spanned the three spring supergroups in SA: Kati Thanda–Lake Eyre, Munda–Lake Frome and Dalhousie (Fig. 1). ‘Supergroups’ are clusters of ‘spring complexes’, which themselves contain ‘spring groups’ composed of individual springs. Spring complexes share similar geomorphological characteristics and water chemistry, whereas groups are clusters of springs sourcing from the same fault or structure (Lewis et al. 2013). Individual springs are composed of permanent wetland vegetation with at least one ‘vent’, a discrete discharge point of water (Gotch 2013). Within these three supergroups, we focused on 23 spring complexes containing 170 spring groups (see Beasley-Hall et al. 2023b for raw research data). Whilst the number of these spring clusters, as well as their naming conventions, have previously not been standardised (Gotch et al. 2016), we selected these locations due to their widespread use in conservation management by the Australian Government (Lewis and White 2013; Department of the Environment 2022).
Springs fed by the Great Artesian Basin (GAB) in South Australia. Springs are classified hierarchically as supergroups, the broadest classification (a, Dalhousie; b, Kati Thanda–Lake Eyre; c, Munda–Lake Frome); complexes (labelled coloured circles); groups (coloured points); springs; and finally vents, discrete water discharge points. The approximate area of the GAB is shown in dark purple and the location of the SA GAB springs is indicated by the dashed square.
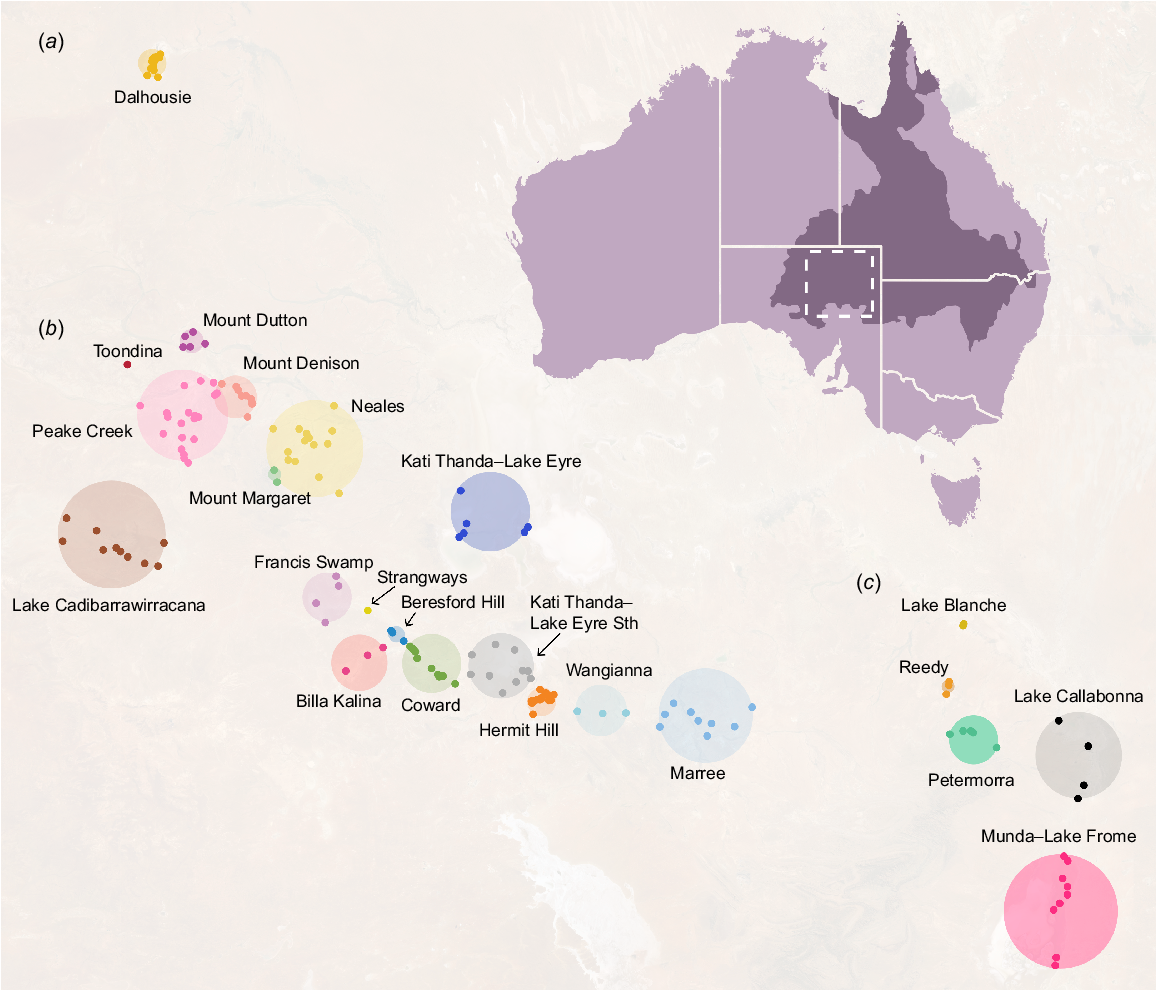
We retrieved Aboriginal names for spring groups from a key anthropological study (Hercus and Sutton 1985) to ensure locations were referred to by a dual naming convention whenever possible. The validity of these names was confirmed by The Arabana Aboriginal Corporation in a personal communication to the authors. For locations with dual names, but lacking ‘official’ formatting (e.g. not used by federal or state government legislation, or publications), we followed the Australian Government (2023) style manual. We chose not to standardise names across springs, groups or complexes as they refer to specific locations with distinct associated mythologies and histories (Hercus and Sutton 1985). Information below the spring group level, where present, was standardised to the group level due to the rarity of such records and the inconsistency of spring vent naming conventions. As GAB spring fauna are often morphologically cryptic yet genetically distinct, we recorded species as separate taxa if explicitly indicated by their species authorities based on genetic data following Rossini et al. (2018).
To supplement presence or absence records of spring taxa, we also gathered information related to taxonomic status, conservation status, common names, synonyms and endemicity where available. Taxonomic status was coded into three categories: taxa for which there is a corresponding formal taxonomic description (‘described’), taxa awaiting taxonomic description (‘undescribed’) or those for which species-level occurrence records were not available (e.g. a family-only record; ‘unidentified’). For taxa with species-level identifications, conservation status information was retrieved from the Species Profile and Threats Database to capture listings under the IUCN Red List of Threatened Species and Australian federal and state environmental legislation (Department of Climate Change, Energy, the Environment and Water 2023). Finally, for each endemic taxon we noted whether it occupied only one spring group, complex or supergroup. Except for undescribed species with well-established occurrence records, endemicity was not recorded for taxa without a species-level identification.
Biodiversity metrics
For each spring group, we first transformed presence or absence records to weight them by whether they represented resolution at the spring group level (hereafter ‘confident records’) or those which referred to a taxon’s presence within a certain complex but did not supply spring group information (hereafter ‘coarse records’). To assess the inclusion of uncertain occurrence information, we calculated metrics for our entire dataset and a subset of the data only considering confident records. As the dataset included only presence or absence records, we were limited in our choice of biodiversity metrics and focused on species richness and endemicity. We calculated species richness values (hereafter ‘taxon richness’) by the number of putative taxa in each spring. Spring groups were ranked by the degree of endemicity of their biota using a modification of the scoring system developed by Fensham and Price (2004). Originally applied to GAB flora in spring complexes in Queensland, the ranking has since been expanded to fauna across Australia (Rossini et al. 2018) and relies on the number of populations corresponding to the most widespread taxon in a spring dataset. The desert goby Chlamydogobius eremius (Zietz, 1896) is the most widespread SA GAB spring endemic, occurring across 24 known groups (Gotch et al. 2016; Rossini et al. 2018); we used this value as a proxy for the taxon’s number of populations, although we acknowledge this may be an underestimate as spring groups do not necessarily share permanent wetlands. Rankings for each endemic taxon were first calculated by dividing 24 by the number of groups the taxon occurred in such that C. eremius (24 ÷ 24) would receive the lowest ranking due to its comparably widespread distribution. As all endemic taxa assessed here do not occur beyond their respective supergroups, each taxon was then scored by whether it was further restricted to a single spring complex (+1) or group (+2). These scores, hereafter ‘endemicity rankings’, were summed for each spring group. We visualised these metrics using QGIS (ver. 3.30, QGIS Association, see https://qgis.org). Spring groups were first mapped using latitude and longitude information corresponding to vents retrieved from the Government of South Australia’s WaterConnect portal (see https://www.waterconnect.sa.gov.au/Systems/GD/Pages/Default.aspx); for groups containing multiple vents, centroids were calculated to approximate their location. Circles corresponding to the above metrics per group were scaled using the Flannery method. To assess differences in community composition among spring groups, we calculated pairwise Jaccard distances using a binary matrix of presence or absence records using the proxy package (ver. 0.4-27, D. Meyer and C. Buchta, see https://cran.r-project.org/package=proxy) in R (ver. 4.3.0, R Foundation for Statistical Computing, Vienna, Austria, see https://www.r-project.org/). Principal components analysis was performed using the native R package stats (ver. 4.4.0, see https://stat.ethz.ch/R-manual/R-devel/library/stats/html/stats-package.html) and visualised using ggplot2 (ver. 3.5.1, see https://CRAN.R-project.org/package=ggplot2; Wickham 2016). Springs without occurrence records were excluded from the analysis, as were taxa known to occur in SA GAB springs generally but without specific location information. Finally, we produced rarefaction curves using the R package iNEXT (ver. 3.0.1, see https://cran.r-project.org/package=iNEXT; Chao et al. 2014) from our entire dataset and a subset of the data considering only confident records. Rarefaction was performed using the rarefaction and extrapolation models for species richness (q = 0), 95% confidence intervals and 100 replications.
Results
Biodiversity of the SA GAB
The database we compiled based on our comprehensive literature review captured 3463 occurrence records corresponding to 495 distinct and putatively distinct taxa. Of these records, 2300 were considered coarse, namely corresponding to the supergroup or complex level, but not informing the presence of taxa at a specific spring group. Invertebrates were by far the most speciose group in the dataset (42%, 207 taxa) followed by vascular plants (22%, 111 taxa), vertebrates (21%, 102 taxa), algae (14%, 68 taxa; for the purposes of this paper, includes green, golden and blue-green algae), non-vascular plants (1%, 5 taxa) and fungi (0.4%, 2 taxa) (Fig. 2). In total, 65 taxa (13%) are known only from SA GAB springs, almost all of which are invertebrates. Despite the species diversity dominance of invertebrates, these organisms are also the most poorly known in GAB springs from a taxonomic standpoint. Just over one-third of invertebrate taxa have formal taxonomic names (37%), whereas the remainder of the fauna is either undescribed (14%) or has an unknown taxonomic status due to a lack of species-level occurrence records (49%). For other taxonomic groups, taxonomic description ranges were 50–100% (described), 0–1.5% (undescribed) and 0–50% (unidentified). Further, apart from the Gastropoda, no invertebrate taxon has had its conservation status assessed at the global, federal or state level (Fig. 2). In total, 58 of the 170 spring groups assessed in this study had no corresponding occurrence records in the literature. A rarefaction curve derived from the dataset suggests the artesian wetlands of SA have not been adequately surveyed, and if additional locations were examined with equal sampling intensity, dozens of additional taxa would likely be documented (Fig. 3).
Fauna, flora and funga associated with SA GAB spring wetlands, with major organismal groups indicated by grey boxes. Groups lacking species-level records have an unknown conservation status and are indicated with n.d. (no data). Rare and Extinct are respectively specific to South Australia–Tasmania and New South Wales legislation, and only refer to populations or taxa within those states. Silhouette credits Maxime Dahirel, Armelle Ansart, Mathieu Pélissié and Lafage (from PhyloPic).
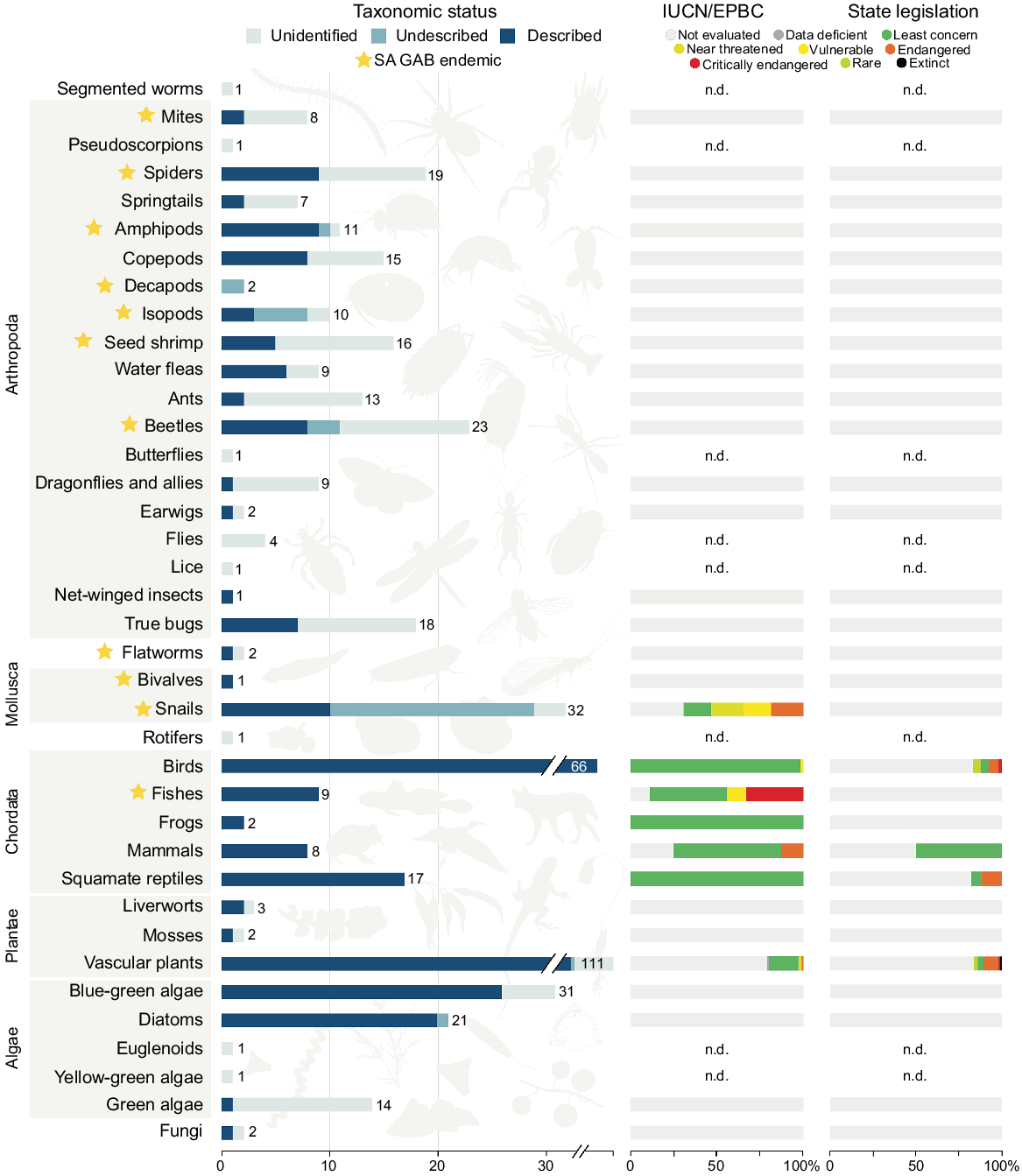
Extrapolation curves predicting an increase in taxon richness if additional spring groups were surveyed, calculated from all occurrence records in the dataset (a) and a subset of the dataset containing only confident records (b). As the curve does not flatten at the maximum number of known spring groups in the state (170), an increase in sampling effort within springs (as opposed to the sole sampling of additional springs) may also capture a more comprehensive representation of these communities. Dotted lines represent extrapolation beyond the taxon richness of our dataset (solid lines); shaded areas represent 95% confidence intervals.
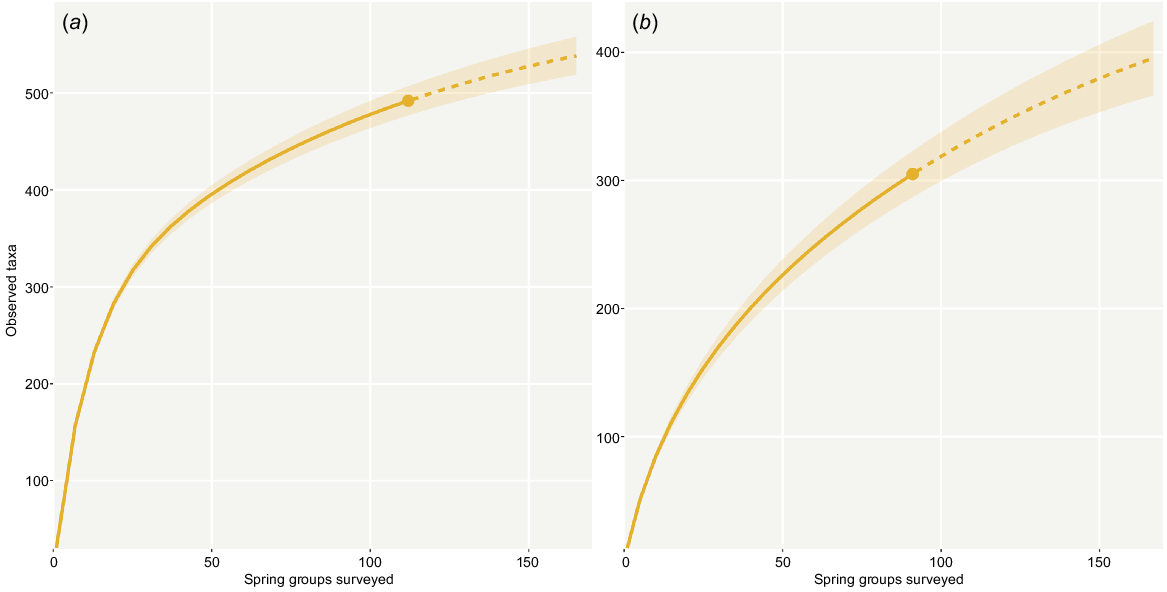
In addition to occurrence records in the dataset, we also identified several records of potential local extinctions in the literature (Table 1). In all, we retrieved absence records corresponding to one isopod taxon, which may represent multiple species (Phreatomerus latipes (Chilton, 1922) Central, North, South haplotypes) (Kinhill-Stearns 1984; Kinhill 1997; Fensham et al. 2010; Guzik et al. 2012), 1 ostracod (Ngarawa dirga De Deckker, 1979) (McLaren et al. 1986; Kinhill 1997; Fensham et al. 2010), 15 snail taxa (Fonscochlea accepta, members of F. aquatica, F. billakalina, F. variabilis, Trochidrobia punicea, T. smithi species complexes, all Ponder, Hershler & Jenkins, 1989; Sinumelon pedasum Iredale, 1937) (Zeidler and Ponder 1989; Ponder et al. 1995; Fensham et al. 2010; Rossini et al. 2018; Department of the Environment 2022) and 4 fishes (Dalhousie goby, Chlamydogobius gloveri Larson, 1995; Lake Eyre hardyhead, Craterocephalus eyresii (Steindachner, 1883); spangled perch, Leiopotherapon unicolor (Günther, 1859); Dalhousie gudgeon, Mogurnda thermophila Allen & Jenkins, 1999) (Zeidler and Ponder 1989; Kodric-Brown et al. 2007; Gotch et al. 2016; Rossini et al. 2018; Department of the Environment 2022; R. Froese and D. Pauly, FishBase, see www.fishbase.se). To the best of our knowledge, these records corresponded to likely local extinctions as opposed to complete species extinctions (i.e. relevant taxa were present in at least one additional location). These likely extirpations have occurred across 13 spring groups in the Dalhousie and Kati Thanda–Lake Eyre supergroups (Table 1). Given the patchy sampling of GAB springs during many surveys, we note that these data may be a result of stochastic sampling effort rather than true extirpations and is therefore an opportunity for future research. However, we note that for certain taxa this evidence is more robust than others; for example, the discovery of empty snail shells at springs (Ponder et al. 1989).
Taxon | Broad grouping | Spring group(s) | Spring complex(es) | |
---|---|---|---|---|
Phreatomerus latipes A, B | Isopod | Venable, Marrinha–Hergott | Hermit Hill, Marree | |
Ngarawa dirga B | Ostracod | Venable, Manda-wardunha–Mundowdna | Hermit Hill, Marree | |
Fonscochlea accepta B | Hydrobiid snail | Venable, Palura Pintjanha–Priscilla | Hermit Hill, KT–LE Sth | |
Fonscochlea aquatica A, B | Hydrobiid snail | Margaret | Francis Swamp | |
Fonscochlea billakalina B | Hydrobiid snail | Margaret | Francis Swamp | |
Fonscochlea variabilis A, B | Hydrobiid snail | Venable, Palura Pintjanha–Priscilla | Hermit Hill, KT–LE Sth | |
Fonscochlea zeidleri A, B | Hydrobiid snail | Venable, Palura Pintjanha–Priscilla, Centre Island | Hermit Hill, KT–LE Sth | |
Trochidrobia punicea A, B | Hydrobiid snail | Venable, Palura Pintjanha–Priscilla | Hermit Hill, KT–LE Sth | |
Trochidrobia smithi A, B | Hydrobiid snail | Margaret | Francis Swamp | |
Sinumelon pedasum | Camaenid snail | Irrwanjira–Errawanyera | Dalhousie | |
Chlamydogobius gloveri | Fish | Irrwanjira–Errawanyera, Frog Dreaming, Kirki–Dalhousie Proper, Cadni Dreaming | Dalhousie | |
Craterocephalus eyresii | Fish | Old Nilpinna, Thuntinha–Toondina | Peake Creek, Toondina | |
Leiopotherapon unicolor | Fish | Idnjundura–Kingfisher, Ilpikwa | Dalhousie | |
Mogurnda thermophila | Fish | Frog Dreaming | Dalhousie |
To our knowledge, there are no records of floral extinctions in the SA GAB. KT–LE Sth, Kati Thanda–Lake Eyre South.
Biodiversity metrics and community composition
Taxon richness was more heavily affected by the exclusion of coarse occurrence records – as most data in this category are not spring endemics – whereas endemicity rankings were essentially unchanged between spring locations. Complexes may also be taxon-rich without containing a large number of endemics (e.g. groups within the Francis Swamp complex; Fig. 4) and vice versa (e.g. Beresford Hill). Groups with high levels of richness values generally corresponded to the Coward, Dalhousie, Billa Kalina and Hermit Hill complexes and those containing large numbers of isolated endemics included Dalhousie, Francis Swamp, Mount Denison and Coward (Fig. 4; see Beasley-Hall et al. 2023c for raw research data).
Rankings of endemicity and overall biodiversity in SA GAB spring groups, with circle size indicating a greater value for a given metric. Larger circles indicate a higher proportion of endemics or more isolated endemic fauna (endemicity ranking, yellow) or a greater number of putative species (taxon richness, purple). Grey circles represent the approximate area of spring groups as shown in Fig. 1. This visualisation was calculated from both coarse and confident records; raw data, including metrics calculated from only ‘confident’ records, are available in Beasley-Hall et al. (2023c).
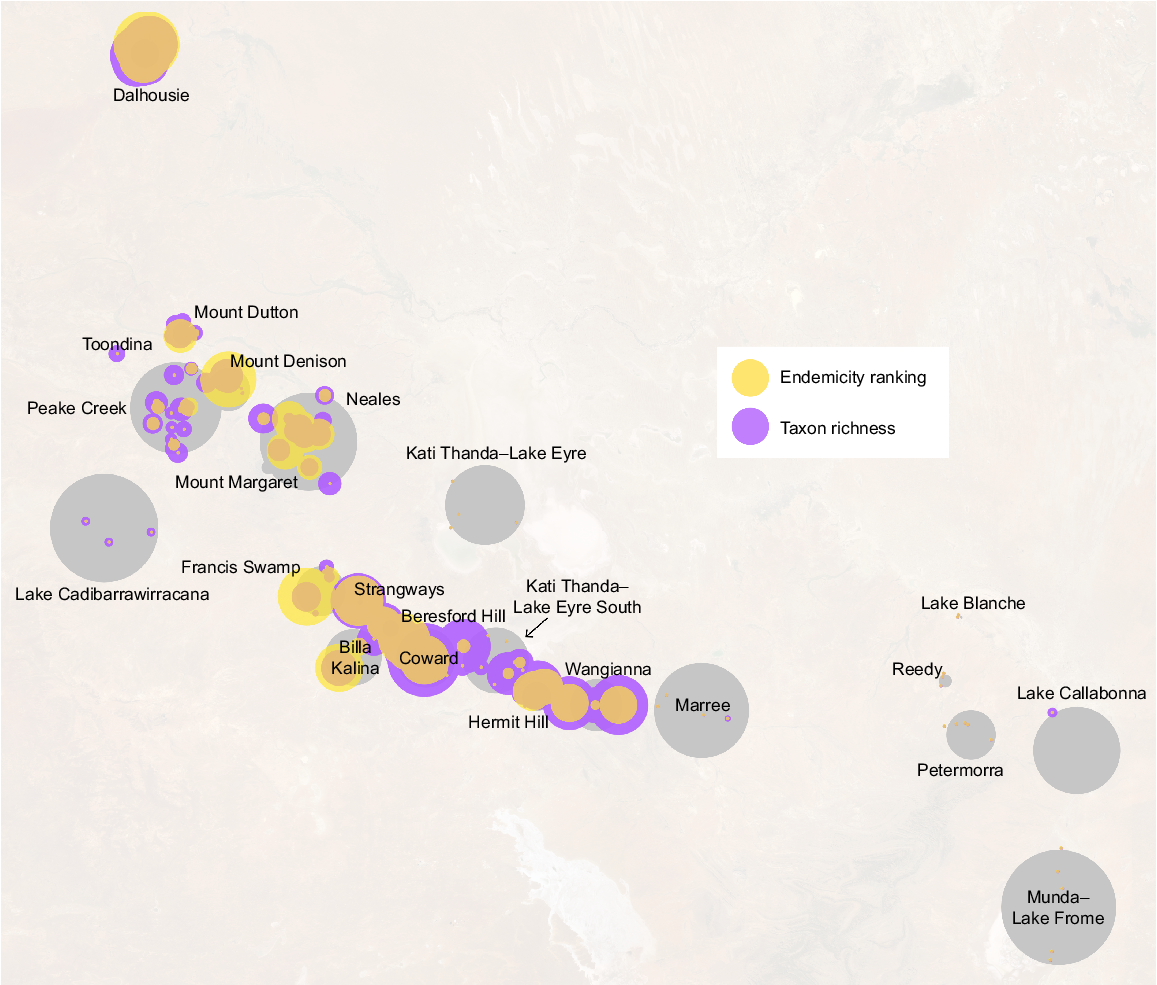
Although there is some degree of overlap regarding taxon composition of spring complexes, particularly in the Kati Thanda–Lake Eyre region (Fig. 5), locations with similar biodiversity metrics do not necessarily support the same biotic communities. The Peake Creek, Lake Cadibarrawirracanna, Neales River and Mount Dutton complexes of the Kati Thanda–Lake Eyre supergroup are generally non-overlapping and groups from within the Dalhousie complex–supergroup are easily differentiated from other complexes (Fig. 5). In contrast, groups from the Munda–Lake Frome complex are nested within those from the Kati Thanda–Lake Eyre supergroup. Groups belonging to the same complex are also generally more similar to one another than those in different complexes. This apparent lack of connectivity is perhaps unsurprising given the aridity of the surrounding landscape. Overall, spring groups within the Dalhousie supergroup–complex are the most distinct in the dataset in that they support taxa not known to be associated with other spring complexes, including highly isolated endemics (Fig. 4 and 5).
Principal components analysis (PCA) showing composition of faunal, floral and fungal communities reliant on SA GAB springs. Both coarse and confident occurrence records in the dataset were used to calculate Jaccard distances between spring groups. Spring groups lacking occurrence records are not shown here. Groups are coded by their respective spring complex (colour) and supergroup (shape). Axis labels refer to the amount of variability explained by each principal component (PC).
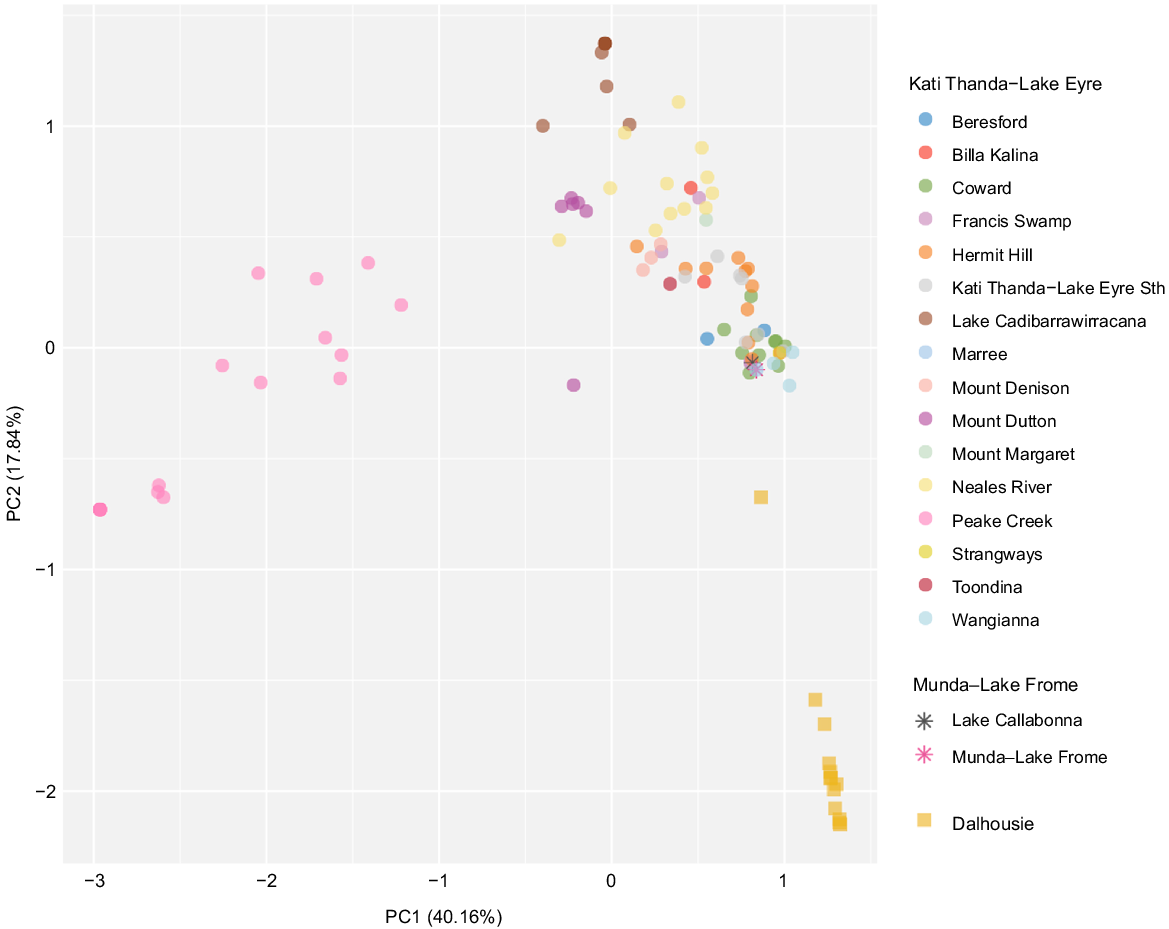
Discussion
Here, we present a robust literature review of the biota associated with artesian wetlands of SA. These data highlight several key trends: (1) invertebrates are a poorly known component of GAB biodiversity relative to the rest of the biota; (2) the composition of GAB biota differs considerably by location, challenging previous conceptions of relevant biodiversity hotspots; and (3) the true extent of GAB biodiversity is clearly far greater than is currently appreciated in the literature. We discuss each of these findings below, as well as conservation implications for GAB springs more broadly and how a centralised biodiversity resource for the GAB could be implemented in future.
Biodiversity of South Australia’s GAB springs
Almost 500 taxa are associated with GAB springs and their surrounding wetlands. Invertebrates represent the largest proportion of this biota and the largest group of endemics, with the majority being insects, crustaceans and arachnids (Fig. 2). Invertebrates are poorly documented from biodiversity, ecological and conservation standpoints relative to the remainder of GAB biota and are also overrepresented in extinction records (Table 1). These groups may therefore be the most at risk of decline due to difficulties in devising management strategies. By contrast, although vertebrates were the third-most speciose group associated with the system (N = 102), almost all were widespread species not of conservation concern (Fensham and Price 2004) (Fig. 2). However, we note here that vertebrates such as waterbirds are nonetheless dependent on the springs as breeding grounds (Badman 1985), and may make more extensive use of these habitats in non-flood years or as ‘stepping stone’ habitats to sustain migrations to nearby Kati Thanda–Lake Eyre. For example, the little grassbird Poodytes gramineus (Gould, 1845) is not restricted to GAB springs, but nonetheless has an obligate relationship with stands of the reed Phragmites australis in GAB wetlands (Read 1997).
Our data suggest a high degree of heterogeneity in the ecological communities of the SA GAB and challenge past assumptions regarding the system’s biodiversity hotspots. It has long been recognised that certain SA GAB springs are more speciose than others, but only recently has a quantitative view of candidate biodiversity hotspots been put forward (Rossini et al. 2018), and without consideration of non-endemic taxa. Springs at Dalhousie are one such hotspot proposed by Rossini et al. (2018), which we support in our review here. We also propose the Francis Swamp, Mount Denison and Coward complexes as hotspots due to their contribution to the remaining bulk of endemicity in the SA GAB. Indeed, these springs house a much more speciose and isolated endemic biota than initially recognised based on published records (Beasley-Hall et al. 2023a). Yet, we note that not all taxon-rich locations are necessarily rich in endemic species (Fig. 4). Overall, our findings indicate the disturbance or extinction of any given spring group could represent an enormous loss of biodiversity when both endemic and non-endemic taxa are considered together. The biodiversity of the SA GAB has not been adequately surveyed (Fig. 3) and over two-thirds of records we collated were coarse, meaning they lacked occurrence information at the spring group level. Although records are helpful in distinguishing spring group locations and suggest considerable structure in taxa occupying different spring complexes and supergroups (Fig. 5), they nonetheless highlight a lack of detailed past surveys and the sparseness of published information on GAB biodiversity.
The observed variability in the dataset may also be associated with sampling bias. Spring groups with the highest endemicity rankings, such as those within the Dalhousie and Coward complexes, are also those consistently associated with high sampling effort in previous surveys (Badman 1985; Greenslade 1985; Mitchell 1985; Sokol 1987; Zeidler and Ponder 1989; Noack 1994; Ponder et al. 1995; Kovac 2003). The Kati Thanda–Lake Eyre and Dalhousie supergroups corresponded to over 2000 occurrence records, whereas observations within the Munda–Lake Frome supergroup were restricted to a single, coarse occurrence record of the copepod Microcyclops dengizicus (Lepeschkin, 1900) (Zeidler and Ponder 1989). Munda–Lake Frome has clearly not been adequately sampled, although this does not necessarily imply the location lacks endemic taxa yet to be characterised (McLaren et al. 1986; Rossini 2020). Indeed, widespread taxonomic groups expected to be present in springs, such as the Hymenoptera and other cosmopolitan insect groups, were also poorly represented in the dataset due to a presumed bias in sampling methods and a lack of past taxonomic expertise. As such, we caution against the derivation of conservation priorities from locations with high measures of metrics such as species richness alone, but trends are nonetheless evident in this review regarding spring locations and taxonomic groups that have been undersampled.
Conservation implications for South Australia’s artesian springs
At least 14 taxa may have become locally extinct in the SA GAB springs (Table 1). Evidence for extirpations includes survey work conducted prior to the extinction of springs themselves or, conversely, the discovery of remains at extinct springs (e.g. snail shells). The bulk of these likely extirpations have occurred in spring groups that have ceased to flow because of drawdown, namely Venable, Palura Pintjanha–Priscilla, Marrinha–Hergott, Margaret and Manda-wardunha–Mundowdna (Fensham et al. 2010). The Venable and Palura Pintjanha–Priscilla spring groups became extinct around 1990 following predictions by industry that mining activities would lead to a partial, if not complete, reduction in artesian flow at certain GAB springs (Kinhill-Stearns 1982, 1983). Marrinha–Hergott ceased to flow in the mid-1980s as water was withdrawn to supply the nearby town of Marree (McLaren et al. 1986, p. 198). Other extirpations have been attributed to human modification of springs, the presence of overabundant invasive and native species, and potentially insufficient sampling effort. The apparent local extinction of the fish species, Lake Eyre hardyhead (Craterocephalus eyresii) at Toondina and Old Nilpinna, may have been caused by spring excavations and competition from the invasive mosquitofish Gambusia holbrooki Girard, 1859, respectively (Gotch et al. 2016). Extirpations of other fish species at Dalhousie have been attributed to the overgrowth of the native reed Phragmites as a result of decreased grazing pressure following the exclusion of livestock (Kodric-Brown et al. 2007). We also note that there are active springs at which only dead specimens of species have been collected, pointing to potential additional extirpations: for example, in certain spring groups within the Neales River and Francis Swamp complexes only empty shells of Fonscochlea zeidleri have been collected, with no evidence of live individuals (Ponder et al. 1989).
It is important to establish conservation priorities given the potential for further local, if not species-wide, extinctions in the SA GAB springs. Here, we ranked spring groups by the number of endemic species supported by each spring group and their degree of isolation. High-ranking locations mirror those proposed as conservation priorities in past studies, corroborating our findings. For example, McLaren et al. (1986) produced an inventory of fauna, flora, and funga to set out conservation priorities based on species diversity, the presence of rare species, ‘naturalness’ (i.e. extent of interference by humans and cattle grazing pressure) and perceived vulnerability to degradation. Spring groups were then ranked by conservation priority. High-ranking spring groups in this assessment include those found at Dalhousie and in the Coward and Mount Denison complexes of the Kati Thanda–Lake Eyre supergroup. Further, Rossini et al. (2018) used a ranking system developed by Fensham and Price (2004) (modified here to produce endemicity rankings) to conclude that groups within the Dalhousie, Strangways, Francis Swamp, Billa Kalina and Mount Denison complexes were of high conservation significance relative to other springs. The highest-ranking spring groups by endemicity in the current study presented here corresponded to all six of the above complexes, often down to the spring group level (Beasley-Hall et al. 2023c). We stress that these trends do not suggest certain spring groups are more important than others or that insignificant springs exist, as has been implied in past environmental impact statements (Kinhill-Stearns 1983; Keane 1997). This is especially the case for locations that could be perceived as having low biological importance, such as the extinct Papu-ngaljuru–Primrose spring group, but have outstanding cultural significance to Aboriginal peoples due to associated Dreaming stories and its use as a major occupation site (Hercus and Sutton 1985). Instead, our findings suggest that certain spring groups harbour high numbers of endemics relative to other locations (but remembering that insufficient sampling effort has occurred) and require targeted conservation efforts to preserve these short-range taxa.
Several conservation programs are currently in place which aim to directly improve the condition of SA GAB spring wetlands (Harris 1992). Preliminary evidence suggests several of these practices have had a positive effect on SA GAB spring endemics. Springs are threatened by the presence of large-bodied and hard-hooved livestock which can graze on or trample wetland vegetation, foul the water through their faeces, and potentially severely reduce invertebrate biodiversity if cattle stocking levels are high; almost all SA GAB springs are subject to such pressure, particularly from cattle (Hutchinson and King 1980; Kovac and Mackay 2009; Fensham et al. 2010; Gotch et al. 2016). A combination of fencing and destocking has been successful in restoring endemic biodiversity of SA GAB springs in some cases; for example, the endemic salt pipewort Eriocaulon carsonii subsp. carsonii F. Muell. in the Hermit Hill complex (Fatchen and Fatchen 1993) and aquatic invertebrates at springs that were heavily damaged by stock (Kinhill-Stearns 1984). The spring groups identified here as harbouring a large number of endemic species could represent future candidates for fencing or destocking initiatives.
As stated above, there is a large proportion of endemic taxa at several complexes within the SA GAB, namely those contained within the Dalhousie and Kati Thanda–Lake Eyre supergroups. The data presented here reiterate the need for targeted surveys in these locations to not only gather additional biodiversity data, but also to develop an understanding of population dynamics, habitat requirements and accidental human-mediated translocations of fauna, flora and funga per the National Recovery Plan (Fensham et al. 2010). The plan notably highlights the fact that the construction of a robust biodiversity inventory for GAB springs has been hindered by a lack of survey effort and taxonomic expertise. The utility of emerging environmental DNA techniques to capture a holistic picture of SA GAB spring biodiversity in a non-invasive manner would overcome several of these limitations (Vörös 2017; West et al. 2020; Saccò et al. 2022; Beasley-Hall et al. 2023a); in this regard, the database developed as part of this study would assist in the selection of fieldwork locations for a pilot study in this regard. Once taxa are established as occurring within the SA GAB, monitoring efforts should be undertaken on a regular basis to develop deeper understandings of ecological knowledge as opposed to presence or absence information.
Although taxa reliant on GAB-fed springs are protected as a single ecological community under the Environment Protection and Biodiversity Conservation Act 1999, species-level listings are also far overdue. This is particularly the case for invertebrates dependent on the GAB. The majority of GAB molluscs and crustaceans meet the criteria to be listed as Critically Endangered (Rossini 2020), but almost 90% of SA GAB invertebrates recorded here lack conservation listing at a global, federal or state level, the bulk of which are either new species awaiting formal description or those completely lacking species-level identifications. This large knowledge gap is only exacerbated by a lack of understanding of the relationship between spring characteristics and biodiversity metrics (Rossini et al. 2018; Fensham and Laffineur 2022) and indeed relationships among spring ecological and hydrogeological characteristics themselves (Mudd 2000; Green and Berens 2013; Love et al. 2013). We hope that resources like the database developed here will inform the prioritisation of certain spring locations and taxonomic groups, ultimately leading to the listing of invertebrates and other spring endemics under relevant legislation on a per-species basis.
Conclusion
Here, we have presented the first review of biota supported by the GAB in SA. This dataset is an important resource we hope will facilitate future studies on GAB spring endemics, investigations into their population dynamics, basic biology and taxonomy, and ultimately facilitate the listing of relevant taxa under state and federal environmental legislation. Such resources are essential given the multitude of threats currently facing springs and their biota, with potential extirpations of populations of at least 13 species in the SA GAB springs to date. We have also highlighted springs of particular conservation concern which may assist in determining future conservation priorities for this system. Our dataset has highlighted three major points about GAB spring biodiversity. Firstly, the majority of taxa reliant on these wetlands are invertebrates, and these animals are also the most poorly known and conserved in the GAB. Secondly, ecological communities reliant on artesian springs in SA are largely non-overlapping, irrespective of whether only endemic taxa, or endemics as well as ‘incidental’ species, are concerned. The extinction or considerable disturbance of any spring group is therefore likely to lead to a considerable loss of biodiversity or genetic diversity. Finally, the artesian springs of SA have not been adequately sampled by past survey efforts and a considerable proportion of taxa likely remain to be documented, particularly in understudied locations such as the Munda–Lake Frome supergroup. There also remains a dearth of occurrence records for certain taxa for which taxonomic expertise has been lacking in the past, such as the wasps and allies (Greenslade 1985). We recommend that datasets such as these are made publicly available with the capacity to be modified and updated on an ongoing basis, embodying the gold standard of digital asset storage.
Data availability
All raw data associated with this paper are available on FigShare (Beasley-Hall et al. 2023b, 2023c). A preprint version of this article has been deposited at bioRxiv (Beasley-Hall et al. 2024).
Conflicts of interest
P. G. Beasley-Hall and M. T. Guzik are independent researchers affiliated with The University of Adelaide and South Australian Museum, and received financial support from industry stakeholders when conducting this study. This funding did not influence the design, data collection, analysis or reporting of this study. Research findings and conclusions expressed in this publication are based solely on the analysis of the data and the scientific merit of the paper.
Declaration of funding
Funding for P. G. Beasley-Hall was provided by contract research from BHP Group Ltd. Funding for B. A. Hedges was provided by an Australian Government Research Training Program (RTP) Scholarship, the Roy and Marjory Edwards Scholarship as administered by Nature Foundation, and the Justin Costelloe Scholarship as administered by the Kati Thanda–Lake Eyre Basin Authority, Department of Agriculture, Water and the Environment. Funding for M. T. Guzik was provided by the Australian Research Council (grant LP190100555) in partnership with Curtin University, The University of Adelaide, BHP Group Ltd, Rio Tinto Ltd, Chevron Australia Pty Ltd, Western Australian Museum, South Australian Museum, the Department for Biodiversity, Conservation and Attractions (WA), and the Western Australian Biodiversity Science Institute, Department of Water and Environmental Regulation (WA).
Acknowledgements
The authors acknowledge the Arabana Aboriginal Corporation, Travis Gotch and Friends of Mound Springs for their guidance and feedback.
References
Andersen M, Barron O, Bond N, Burrows R, Eberhard S, Emelyanova I, Fensham R, Froend R, Kennard M, Marsh N, Pettit N, Rossini R, Rutlidge R, Valdez D, Ward D (2016) Research to inform the assessment of ecohydrological responses to coal seam gas extraction and coal mining. (Department of the Environment and Energy, Commonwealth of Australia) Available at https://www.dcceew.gov.au/sites/default/files/documents/assessment-ecohydrological-responses.pdf
Arabana Aboriginal Corporation (2021) Submission to the inquiry into the destruction of 46,000-year-old caves at the Juukan Gorge in the Pilbara Region of Western Australia. (Commonwealth of Australia: Canberra, ACT Australia) Available at https://www.aph.gov.au/DocumentStore.ashx?id=41e65957-4b2f-4245-bc55-f0198241c6ce&subId=690905
Australian Government (2023) Australian place names. In ‘Australian Government Style Manual’. (Commonwealth of Australia) Available at https://www.stylemanual.gov.au/grammar-punctuation-and-conventions/names-and-terms/australian-place-names
Badman FJ (1985) Birds of the mound springs and bores, south and west of Lake Eyre, with special reference to the Coward Spring area. In ‘South Australia’s mound springs’. (Eds J Greenslade, L Joseph, L Reeves) pp. 84–92. (Nature Conservation Society of South Australia Inc., Hyde Park Press: Plympton, SA, Australia)
Beasley-Hall PG, Murphy NP, King RA, White NE, Hedges BA, Cooper SJB, Austin AD, Guzik MT (2023a) Time capsules of biodiversity: future research directions for groundwater-dependent ecosystems of the Great Artesian Basin. Frontiers in Environmental Science 10, 1021987.
| Crossref | Google Scholar |
Beasley-Hall PG, Hedges BA, Cooper SJB, Austin AD, Guzik MT (2023b) Database collating information on the fauna, flora, and funga of South Australia’s Great Artesian Basin springs. Dataset. (The University of Adelaide) doi:10.25909/24457105
Beasley-Hall PG, Hedges BA, Cooper SJB, Austin AD, Guzik MT (2023c) Species richness and endemicity rankings for each South Australian spring group. Dataset. (The University of Adelaide) doi:10.25909/24457120
Beasley-Hall PG, Hedges BA, Cooper SJB, Austin AD, Guzik MT (2024) A comprehensive review of South Australia’s Great Artesian Basin spring and discharge wetlands biota. bioRxiv 2023.10.29.564639 [Preprint, posted 16 July 2024].
| Crossref | Google Scholar |
Belbin L, Wallis E, Hobern D, Zerger A (2021) The Atlas of living Australia: history, current state and future directions. Biodiversity Data Journal 9, e65023.
| Crossref | Google Scholar | PubMed |
Brack W, Altenburger R, Schüürmann G, Krauss M, López Herráez D, van Gils J, Slobodnik J, Munthe J, Gawlik BM, van Wezel A, Schriks M, Hollender J, Tollefsen KE, Mekenyan O, Dimitrov S, Bunke D, Cousins I, Posthuma L, van den Brink PJ, López de Alda M, Barceló D, Faust M, Kortenkamp A, Scrimshaw M, Ignatova S, Engelen G, Massmann G, Lemkine G, Teodorovic I, Walz K-H, Dulio V, Jonker MTO, Jäger F, Chipman K, Falciani F, Liska I, Rooke D, Zhang X, Hollert H, Vrana B, Hilscherova K, Kramer K, Neumann S, Hammerbacher R, Backhaus T, Mack J, Segner H, Escher B, de Aragão Umbuzeiro G (2015) The SOLUTIONS project: challenges and responses for present and future emerging pollutants in land and water resources management. Science of The Total Environment 503–504, 22-31.
| Crossref | Google Scholar | PubMed |
Chao A, Gotelli N, Hsieh T, Sander E, Ma K, Colwell R, Ellison A (2014) Rarefaction and extrapolation with Hill numbers: a framework for sampling and estimation in species diversity studies. Ecological Monographs 84, 45-67.
| Crossref | Google Scholar |
Department of Climate Change, Energy, the Environment and Water (2023) Species profile and threats database. Available at http://www.environment.gov.au/cgi-bin/sprat/public/sprat.pl [Verified 26 April 2023]
Department of the Environment (2022) Species Profile and Threats Database: the community of native species dependent on natural discharge of groundwater from the Great Artesian Basin. (Australian Government Department of the Environment: Canberra, ACT, Australia) Available at https://www.environment.gov.au/cgi-bin/sprat/public/publicshowcommunity.pl?id=26
Fairfax RJ, Fensham RJ (2002) In the footsteps of J. Alfred Griffiths: a cataclysmic history of Great Artesian Basin Springs in Queensland, Australia. Australian Geographical Studies 40, 210-230.
| Crossref | Google Scholar |
Famiglietti JS (2014) The global groundwater crisis. Nature Climate Change 4, 945-948.
| Crossref | Google Scholar |
Fatchen TJ (2000) Mound springs management planning: management issues, strategies, and prescriptions for mound springs in Far North South Australia. Report prepared for the South Australian Department of Environment and Heritage with the support of the Natural Heritage Trust. (Government of South Australia: Adelaide, SA, Australia) Available at https://data.environment.sa.gov.au/Content/Publications/MoundSpringsManagementPlanning-FarNorthSA_2000.pdf
Fensham RJ, Laffineur B (2022) Response of spring wetlands to restored aquifer pressure in the Great Artesian Basin, Australia. Journal of Hydrology 612, 128152.
| Crossref | Google Scholar |
Fensham RJ, Price RJ (2004) Ranking spring wetlands in the Great Artesian Basin of Australia using endemicity and isolation of plant species. Biological Conservation 119, 41-50.
| Crossref | Google Scholar |
Fensham RJ, Ponder WF, Fairfax RJ (2010) Recovery plan for the community of native species dependent on natural discharge of groundwater from the Great Artesian Basin. Report to Department of the Environment, Water, Heritage and the Arts, Canberra, ACT, Australia. (Queensland Department of Environment and Resource Management: Brisbane, Qld, Australia) Available at https://www.dcceew.gov.au/sites/default/files/documents/great-artesian-basin-ec.pdf
Fensham RJ, Silcock JL, Powell O, Habermehl MA (2016) In search of lost springs: a protocol for locating active and inactive springs. Groundwater 54, 374-383.
| Crossref | Google Scholar |
Gotch TB, Adams M, Murphy NP, Austin AD (2008) A molecular systematic overview of wolf spiders associated with Great Artesian Basin springs in South Australia: evolutionary affinities and an assessment of metapopulation structure in two species. Invertebrate Systematics 22, 151-165.
| Crossref | Google Scholar |
Gotch T, Keppel M, Fels K, McKenzie J (2016) Lake Eyre Basin Springs Assessment: ecohydrological conceptual models of springs in the western Lake Eyre Basin, South Australia. DEWNR Technical report 2016/02, Government of South Australia, through Department of Environment, Water and Natural Resources, Adelaide, SA, Australia.
Great Artesian Basin Coordinating Committee (2019) Great Artesian Basin Strategic Management Plan 2019. (Department of Climate Change, Energy, the Environment and Water) Available at https://www.dcceew.gov.au/sites/default/files/documents/strategic-management-plan.pdf
Green G, Berens V (2013) Relationship between aquifer pressure changes and spring discharge rates. In ‘Allocating Water and Maintaining Springs in the Great Artesian Basin, Volume III: groundwater discharge of the western Great Artesian Basin’. pp. 101–114. (National Water Commission: Canberra, ACT, Australia)
Guzik MT, Adams MA, Murphy NP, Cooper SJB, Austin AD (2012) Desert springs: deep phylogeographic structure in an ancient endemic crustacean (Phreatomerus latipes). PLoS ONE 7, e37642.
| Crossref | Google Scholar | PubMed |
Guzik MT, Stringer DN, Murphy NP, Cooper SJB, Taiti S, King RA, Humphreys WF, Austin AD (2019) Molecular phylogenetic analysis of Australian arid-zone oniscidean isopods (Crustacea: Haloniscus) reveals strong regional endemicity and new putative species. Invertebrate Systematics 33, 556-574.
| Crossref | Google Scholar |
Habermehl MA (2020) Review: the evolving understanding of the Great Artesian Basin (Australia), from discovery to current hydrogeological interpretations. Hydrogeology Journal 28, 13-36.
| Crossref | Google Scholar |
Harris CR (1992) Mound springs: South Australian conservation initiatives. The Rangeland Journal 14, 157-173.
| Crossref | Google Scholar |
Harvey MS (1989) A review of the water mite family Anisitsiellidae in Australia (Acarina). Invertebrate Systematics 3, 629-646.
| Crossref | Google Scholar |
Hutchinson KJ, King KL (1980) The effects of sheep stocking level on invertebrate abundance, biomass and energy utilization in a temperate, sown Grassland. Journal of Applied Ecology 17, 369-387.
| Crossref | Google Scholar |
Keane D (1997) The sustainability of use of groundwater from the southwestern edge of the Great Artesian Basin, with particular reference to the impact on the mound springs of the borefields of Western Mining Corporation. Environmental Engineering Design thesis, RMIT University. Available at https://nuclear.foe.org.au/wp-content/uploads/Keane-Mound-Springs-97.pdf
Kerezsy A (2015) Development of a technique for quarantining Great Artesian Basin springs from colonisation by the invasive fish Eastern Gambusia (Gambusia holbrooki). Ecological Management & Restoration 16, 229-232.
| Crossref | Google Scholar |
King R (2009) Two new genera and species of chiltoniid amphipods (Crustacea: Amphipoda: Talitroidea) from freshwater mound springs in South Australia. Zootaxa 2293, 35-52.
| Crossref | Google Scholar |
King RA, Leys R (2014) Molecular evidence for mid-Pleistocene divergence of populations of three freshwater amphipod species (Talitroidea: Chiltoniidae) on Kangaroo Island, South Australia, with a new spring-associated genus and species. Australian Journal of Zoology 62, 137-156.
| Crossref | Google Scholar |
Kodric-Brown A, Wilcox C, Bragg JG, Brown JH (2007) Dynamics of fish in Australian desert springs: role of large-mammal disturbance. Diversity and Distributions 13, 789-798.
| Crossref | Google Scholar |
Kovac K-J (2003) Bird surveys at Dalhousie Springs. In ‘Expedition Witjira: Interim Report, 12–26 July 2003’. p. 30. (Scientific Expedition Group with National Parks and Wildlife and Department of Environment and Heritage, Unley, SA, Australia) Available at https://data.environment.sa.gov.au/Content/Publications/ExpeditionWitjira12-26July2003-BiodiversitySurveyMoundSprings-INTREPORT_2006.pdf
Kovac K-J, Mackay DA (2009) An experimental study of the impacts of cattle on spider communities of artesian springs in South Australia. Journal of Insect Conservation 13, 57-65.
| Crossref | Google Scholar |
Lewis S, Harris C (2020) Improving conservation outcomes for Great Artesian Basin springs in South Australia. The Proceedings of the Royal Society of Queensland 126, 271-287.
| Crossref | Google Scholar |
Mudd GM (2000) Mound springs of the Great Artesian Basin in South Australia: a case study from Olympic Dam. Environmental Geology 39, 463-476.
| Crossref | Google Scholar |
Murphy NP, Adams M, Austin AD (2009) Independent colonization and extensive cryptic speciation of freshwater amphipods in the isolated groundwater springs of Australia’s Great Artesian Basin. Molecular Ecology 18, 109-122.
| Crossref | Google Scholar | PubMed |
Murphy NP, Breed MF, Guzik MT, Cooper SJB, Austin AD (2012) Trapped in desert springs: phylogeography of Australian desert spring snails. Journal of Biogeography 39, 1573-1582.
| Crossref | Google Scholar |
Murphy NP, Adams M, Guzik MT, Austin AD (2013) Extraordinary micro-endemism in Australian desert spring amphipods. Molecular Phylogenetics and Evolution 66, 645-653.
| Crossref | Google Scholar | PubMed |
Murphy NP, Guzik MT, Cooper SJB, Austin AD (2015) Desert spring refugia: museums of diversity or evolutionary cradles? Zoologica Scripta 44, 693-701.
| Crossref | Google Scholar |
Noack D (1994) Date palms and the exotic flora of Dalhousie Springs. South Australian Geographical Journal 93, 81-89.
| Google Scholar |
Obura DO, Aeby G, Amornthammarong N, Appeltans W, Bax N, Bishop J, Brainard RE, Chan S, Fletcher P, Gordon TAC, Gramer L, Gudka M, Halas J, Hendee J, Hodgson G, Huang D, Jankulak M, Jones A, Kimura T, Levy J, Miloslavich P, Chou LM, Muller-Karger F, Osuka K, Samoilys M, Simpson SD, Tun K, Wongbusarakum S (2019) Coral reef monitoring, reef assessment technologies, and ecosystem-based management. Frontiers in Marine Science 6, 580.
| Crossref | Google Scholar |
Ordens CM, McIntyre N, Underschultz JR, Ransley T, Moore C, Mallants D (2020) Preface: advances in hydrogeologic understanding of Australia’s Great Artesian Basin. Hydrogeology Journal 28, 1-11.
| Crossref | Google Scholar |
Ponder WF, Hershler R, Jenkins BJ (1989) An endemic radiation of hydrobiid snails from artesian springs in northern South Australia: their taxonomy, physiology, distribution and anatomy. Malacologia 31, 1-140.
| Google Scholar |
Ponder WF, Eggler P, Colgan DJ (1995) Genetic differentiation of aquatic snails (Gastropoda: Hydrobiidae) from artesian springs in arid Australia. Biological Journal of the Linnean Society 56, 553-596.
| Crossref | Google Scholar |
Queensland Department of Regional Development, Manufacturing and Water (2023) Queensland’s plan to protect our Great Artesian Basin. ArcGIS StoryMaps. Available at https://storymaps.arcgis.com/stories/259e2ef480944f799fb2465ba2821877 [Verified 20 April 2023]
Queensland Government (2018) Springs database. Open Data Portal. Available at https://www.data.qld.gov.au/dataset/springs [Verified 1 May 2023]
Read JL (1997) Stranded on desert islands? Factors shaping animal populations in Lake Eyre South. Global Ecology and Biogeography Letters 6, 431-438.
| Crossref | Google Scholar |
Rossini RA (2020) Current state and reassessment of threatened species status of invertebrates endemic to Great Artesian Basin Springs. The Proceedings of the Royal Society of Queensland 126, 225-248.
| Crossref | Google Scholar |
Rossini RA, Fensham RJ, Stewart-Koster B, Gotch T, Kennard MJ (2018) Biogeographical patterns of endemic diversity and its conservation in Australia’s artesian desert springs. Diversity and Distributions 24, 1199-1216.
| Crossref | Google Scholar |
Saccò M, Guzik MT, van der Heyde M, Nevill P, Cooper SJB, Austin AD, Coates PJ, Allentoft ME, White NE (2022) eDNA in subterranean ecosystems: applications, technical aspects, and future prospects. Science of The Total Environment 820, 153223.
| Crossref | Google Scholar |
Sokol A (1987) Yabbies at Dalhousie Springs, northern South Australia: morphological evidence for long-term isolation. Transactions of the Royal Society of South Australia, Incorporated 111, 207-210.
| Google Scholar |
Vaughan H, Brydges T, Fenech A, Lumb A (2001) Monitoring long-term ecological changes through the ecological monitoring and assessment network: science-based and policy relevant. Environmental Monitoring and Assessment 67, 3-28.
| Crossref | Google Scholar | PubMed |
Vörös J (2017) Hunting the olm with eDNA. Herpetological Review 48, 266.
| Google Scholar |
West KM, Richards ZT, Harvey ES, Susac R, Grealy A, Bunce M (2020) Under the karst: detecting hidden subterranean assemblages using eDNA metabarcoding in the caves of Christmas Island, Australia. Scientific Reports 10, 21479.
| Crossref | Google Scholar | PubMed |