The risky nightlife of undersized sea urchins
Jennifer E. Smith


A
Abstract
Longspined sea urchins (Centrostephanus rodgersii) form extensive urchin barrens in south-eastern Australia, threatening biodiversity and lucrative fishery stocks. Although large urchins are readily visible on reefs, small or ‘undersized’ urchins have often been considered non-emergent, cryptic, and largely inaccessible to predators, meaning smaller predators are considered not to contribute to top–down urchin control.
Here, we aim to investigate variation in nocturnal movement across urchin size classes and discuss the associated ecological implications.
Using timelapse footage we measured timing of movement, distance covered, and displacement of different sized sea urchins in various habitats.
Small urchins emerge from cryptic habitats and are active overnight on open reef areas. At dusk, smaller urchins emerge later than larger urchins, whereas at dawn, movement of all size classes of urchins decline at a similar rate.
The nocturnal emergence and movement of small urchins on open reef spaces makes them accessible to nocturnal predators, such as the southern rock lobster (Jasus edwardsii).
This time–space overlap of predator and prey implies that rock lobsters (including small lobsters) may be inflicting higher predatory pressure than previously considered on undersized sea urchins.
Keywords: activity, Centrostephanus, cryptic, movement, nocturnal, range-extension, sea urchins, size-based analysis, Tasmania, video analysis.
Introduction
Globally, sea urchins are known to overgraze kelp-dominated marine habitat with dramatic consequences for the wider ecosystem (Ling et al. 2015). Recovery of these habitats is possible but requires comprehensive understanding of the ecology of the sea urchins and interactions with local species (Filbee-Dexter and Scheibling 2014; Miller et al. 2022). In Tasmania, south-eastern Australia, the range-extending longspined sea urchin (Centrostephanus rodgersii) forms extensive urchin barrens by overgrazing algal habitats, threatening reef biodiversity and productivity (Johnson et al. 2005; Ling 2008). These urchins are an increasing threat to Tasmanian coastal ecosystems as well as reef-dependent fisheries. After being first reported in Tasmania in 1978, over 20 million C. rodgersii are now estimated to be on the east coast (Johnson et al. 2005; Ling and Keane 2018). This rapid expansion highlights the importance of investigating and documenting both the urchin’s ecology and the effectiveness of control attempts.
Spiny lobsters are known to predate on C. rodgersii, both in their native and extended range (Ling et al. 2009; Day 2020). In the extended range of C. rodgersii, their primary predator is the southern rock lobster (Jasus edwardsii) (Ling and Johnson 2012). Large lobsters (>140-mm carapace length, CL) are known to provide top-down predatory control in some areas where they are protected (Ling and Johnson 2012; Ling and Keane 2021). The southern rock lobster largely forages nocturnally (Williams and Dean 1989; MacDiarmid et al. 1991; Frusher et al. 2009), overlapping with the time during which C. rodgersii are most active (Flukes et al. 2012). Further, lobster tracking experiments in eastern Tasmania highlighted that nocturnal foraging is most pronounced in small lobsters of CL <140 mm (Frusher et al. 2009).
The size of individual lobsters and urchins plays an important role in their predator–prey interaction (Tegner and Levin 1983). It has been assumed that small C. rodgersii are largely cryptic until they reach a size of emergence (~50–70 mm), which means they are protected and hidden from many of their predators (Ling et al. 2009; Ling and Johnson 2012). Only larger lobsters are believed to be capable of predating on the post-emergent C. rodgersii due to their large spine canopy (SC; Ling et al. 2009). As such, small lobsters are not generally considered in management practises to provide predatory pressure, as they cannot attack post-emergent C. rodgersii and are largely unable to access small cryptic urchins (Department of Primary Industries, Parks, Water and Environment 2018). Despite this, C. rodgersii has been found to significantly contribute to the diet of lobsters of all sizes, including small lobsters, somewhat conflicting this hypothesis (Redd et al. 2008, 2014; Day 2020; Smith et al. 2023). Small lobsters have also been shown to be capable of predating on small C. rodgersii in captivity and tethered C. rodgersii in the wild away from cryptic refugia (Ling et al. 2009; Smith et al. 2022).
The aim of this study is to investigate variation in nocturnal movement across C. rodgersii size classes and link this to the associated ecological implications. We hypothesise that small C. rodgersii are nocturnally active and emerge from daytime cryptic refugia overlapping with nocturnal lobster foraging events, providing a window of opportunity for smaller lobsters to predate on small urchins. In this way, all size classes of lobster contribute to C. rodgersii predation and therefore should not be overlooked in the context of top-down ecosystem management.
Materials and methods
Time-lapse photography
Previously, 15 time-lapse videos filmed in eastern Tasmania during summer (November 2009–February 2010) were analysed for urchin movement as described in Flukes et al. (2012). Sites were specifically chosen to be spatially independent and represent one of three distinct habitat types: widespread urchin barrens (grazed areas >104 m2) composed of flat rock; widespread barrens composed of boulders; and incipient barrens (grazed patches 1–10 m2). Nikon D200 digital SLR and Pentax Optio W80 digital compact cameras equipped with red lighting were installed by SCUBA divers on adjustable tripods. Camera time-lapse photos were saved every 5 min and recording ran for 12 h each night, commencing prior to sunset (19:30 hours) and continued to after sunrise (07:30 hours). The measure tool in imageJ (ver. 1.52, W. S. Rasband, US National Institutes of Health, Bethesda, MD, USA, see https://imagej.nih.gov/ij/; Schneider et al. 2012) was used to measure the diameter of the SC of each urchin, recorded in pixels. For each individual urchin, SC was measured four times and the results averaged across replicate measurements to account for different positions of the urchins during the video. For distance travelled and displacement data, only the subset of measured urchins that remained within the field of view (FOV) for the entire night were included (n = 182). Urchins which emerged from and retreated to a crevice within the FOV during any point in the video were also included for distance and displacement measurements. For each of these urchins, visibility of the urchin at the beginning and the end of the video was manually sampled. The FOV photographed by the cameras varied from ~5 to 30 m2 depending on both the camera system used and the adjusted height of the tripod (0.8–3 m from the substratum, depending on topography). The position of each urchin in each frame was determined by x–y coordinates in pixels. Calibration of the time-lapse frame (mm per pixel) was obtained prior to this study, by measuring known distances between features in the FOV (Flukes et al. 2012).
Spine canopy – test diameter ratio
The test diameter (TD) and SC of 211 urchins collected from Fortescue Bay, Tasmania (incipient barren) in May–June 2023 were measured using digital callipers. A log-linear model was applied to determine the relationship between SC and TD and convert SC measurements of urchins in the overnight videos to TD.
Data analysis
The predation limit relationship between lobster size (CL) and sea urchin TD (TD = 0.6 × 5.12e0.023CL; Ling et al. 2009) was used to classify sea urchins into three size classes based on (a) the reported size of lobsters capable of urchin predation (140 mm CL) and (b) the lobster fishery size limit (110 mm CL), as used by Smith et al. (2022). Sea urchin size classes were ‘small’ (<39 mm TD, considered non-emergent and accessible to small lobsters <110 mm CL), ‘medium’ (39–77 mm TD, considered emerging and accessible to medium lobsters 110–140 mm CL), and ‘large’ (>77 mm TD, considered emergent and accessible only to large lobsters >140 mm CL). Movement rate was determined by the proportion of frames within which an urchin was scored as moving (i.e. the urchin was in a different x–y position from the previous frame, with a minimum step length of 10 mm as in Flukes et al. 2012). Movement rate was then averaged across the urchin size class for daylight period (daylight, dusk, night, dawn) as determined by times at sunrise and sunset at each deployment site. A linear mixed effects model (lmer) was used to determine the importance of size class, daylight period, and their interaction on urchin movement during the recording period. Individual urchin was included as a random factor to account for the individual urchins measured across multiple daylight periods. Habitat type, which is known to influence urchin movement (Flukes et al. 2012), was pooled for analysis following initial analysis which showed it did not interact with size to influence movement. For distance travelled, a linear model was used to determine the importance of size class in the total distance moved by each urchin. Net displacement of each urchin was calculated as the straight-line distance between its x–y position in the first frame and in the final frame. The movement model was fitted using the package lme4 (ver. 1.1-31, see https://cran.r-project.org/package=lme4; Bates et al. 2015) as lmer(movement ~ daylight-period × size-class, (1|Urchin-ID)), the distance travelled and displacement models were fit as lm(distance ~ size-class) and lm(displacement ~ size-class). ANOVA was used to determine each model’s predictiveness and post hoc multiple comparisons were used to compare between factors (Bonferroni corrected). Interactions were explored using t-tests and visualised using the package sjPlot (ver. 2.8.12, see https://cran.r-project.org/package=sjPlot) if significant. Data analysis was conducted with R (ver. 4.1.1, R Foundation for Statistical Computing, Vienna, Austria, see https://www.r-project.org/) in RStudio (Posit Software, PBC, Boston, MA, USA, see https://posit.co/products/open-source/rstudio/) using the packages tidyverse (ver. 2.0.0, see https://cran.r-project.org/package=tidyverse; Wickham et al. 2019), lubridate (ver. 1.9.2, see https://cran.r-project.org/package=lubridate; Grolemund and Wickham 2011) and multcomp (ver. 1.4-20, see https://cran.r-project.org/package=multcomp; Hothorn et al. 2008).
Results and discussion
Test diameter and spine canopy ratio
Centrostephanus rodgersii SC was positively correlated with TD and followed a log-linear relationship:
where y is SC and x is TD (R2 = 0.87, Fig. 1). The proportionally longer spines on smaller urchins is likely a predator avoidance adaptation, as long spines are known to confer greater resistance against predators (Ling and Johnson 2009). A gap in the data relating to urchins between 41 and 60 mm TD may be attributed to a low recruitment event at this site. Despite this gap in the measured TD-SC data, the high R2 value confirms the model fits well and is suitable to use.
Movement of urchins overnight
The time-lapse footage identified a total of 292 urchins ranging in size from 75 to 253 mm SC, which converted to 20–122 mm TD, this conversion facilitates association to the size at which urchins may be subject to predation by lobster (Ling et al. 2009). Using the upper limit of lobster–urchin predation size classes from Ling et al. (2009), urchins of all three size classes were identified in the time-lapse videos: ‘small’ (n = 32), ‘medium’ (n = 224) and ‘large’ (n = 36). Both medium and small urchin size classes (88% of urchins recorded) include those considered to be cryptic and inaccessible to predation. Previously, nocturnal activity levels of small urchins has not been reported, and thus their cryptic, crevice-dwelling daylight behaviour has only been considered (Andrew and Underwood 1989; Ling et al. 2009; Ling and Johnson 2012; Byrne and Andrew 2020). Here, overnight underwater filming (by Flukes et al. 2012) and identification of individual urchin sizes has allowed size-specific monitoring of nocturnal urchin movement and proved that small urchins leave their crevices during nocturnal hours (Fig. 2). Of those urchins whose track remained within the FOV all night, only 50% of small urchins were visible at the beginning and end of the filming period (daytime), which was lower than medium (65–70%) and large (67–72%) urchins, indicating that small urchins have heightened cryptic behaviour.
Photographs taken from the timelapse footage at one site showing Centrostephanus rodgersii during daylight (a) and night (b) to demonstrate the crevice-dwelling behaviour seen during the day and the increase in movement during the night. Small black circles are C. rodgersii. Also visible in the field of view is kelp cover (left), boulders, and camera or light tripod legs.
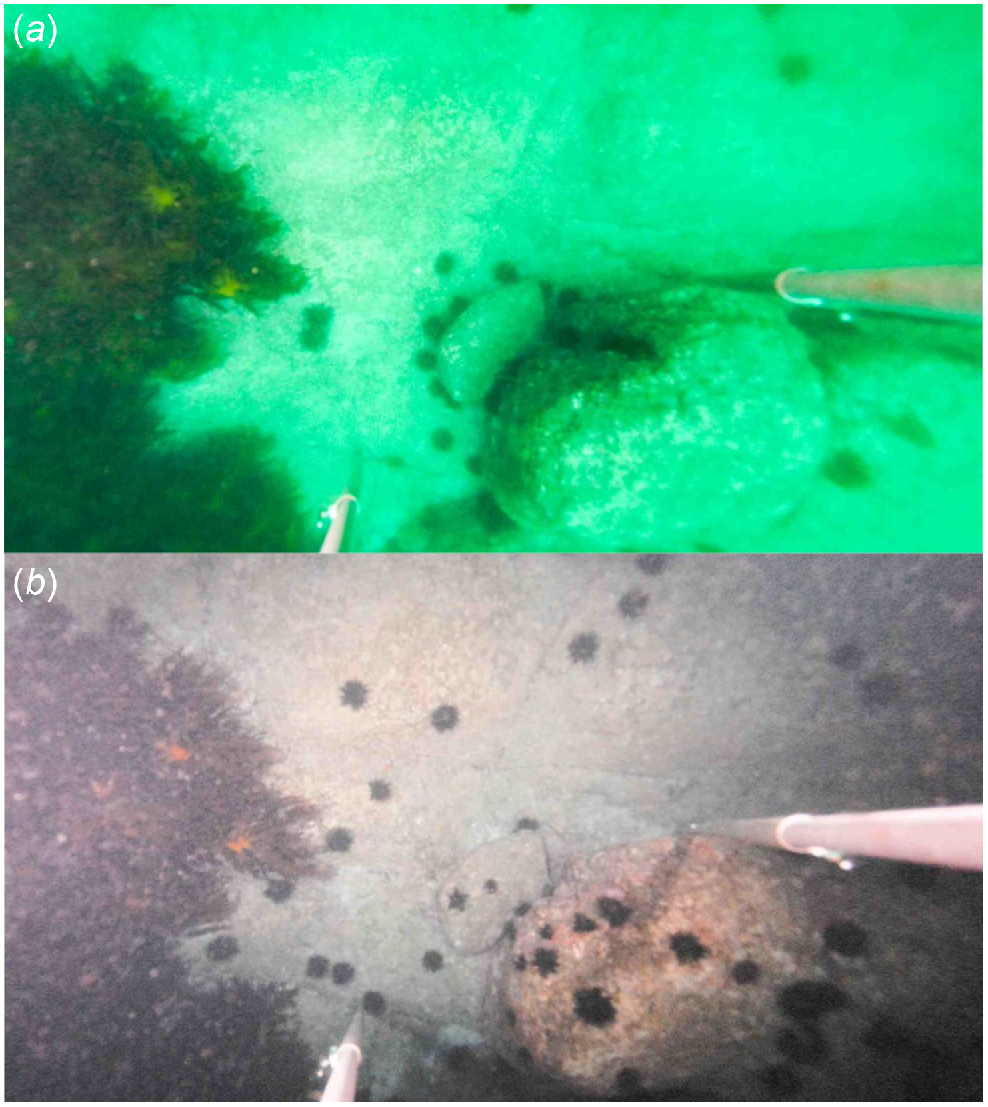
Size class, daylight period and their interaction all had a significant effect on the movement rate of sea urchins (ANOVA: size F(2,388) = 8.04, P < 0.001; daylight period F(3,2540) = 366.0, P < 0.001; interaction F(6,2539) = 3.15, P = 0.004). Overall, urchin movement was highest during night-time (z(night–dawn) = 26.7, P < 0.001; z(night–day) = 41.7, P < 0.001; z(night–dusk) = 18.6, P < 0.001) and showed the lowest movement during the daylight period (z(day–dawn) = −10.1, P < 0.001; z(dusk–day) = 13.3, P < 0.001; z(night–day) = 41.7, P < 0.001, Fig. 3a, Table 1), corroborating the results found by Flukes et al. (2012). The interaction between size and daylight period was explained by small urchins moving significantly less during the dusk period (Supplementary Fig. S1, t(small–medium) = −4.14, P < 0.001; t(small–large) = −4.20, P < 0.001), when the medium and large urchins had increased movement rates, indicating a delayed onset of nocturnal activity in smaller urchins (Fig. 3a). At night-time, small urchins also moved marginally less than the larger size classes (F(2,270) = 3.36, P = 0.036). Post hoc tests indicated there was limited difference in movement between small and medium size urchins (z(small-med) = −2.39, P = 0.05), but no significant difference between the other groups (z(medium–large) = −0.68, P = 1; z(small–large) = −2.26, P = 0.07). Increased sample size may further elucidate the pattern seen here, but this result suggests that small urchins are just as active overnight as larger size classes. Habitat was confirmed not to interact with size to influence movement (F(4, 368) = 1.31, P = 0.27). High and consistent levels of movement across all size classes of urchin overnight demonstrates that all sizes of urchins, even those that are cryptic in the day, are nocturnally active. The reduction in movement of all urchins towards dawn aligns with the previously described behaviour of C. rodgersii returning to their crevices in the morning and becoming diurnally cryptic (Byrne and Andrew 2020).
Differences in urchin movement rate and distance between size classes. All error bars represent ±s.e. (a) Mean movement rate between each daylight period (daylight, dusk, night, dawn). (b) Mean distance travelled (mm) by each urchin size class across the 12-h recording period. (c) Mean distance travelled scaled to test diameter (mm) by each urchin size class across the 12-h recording period.
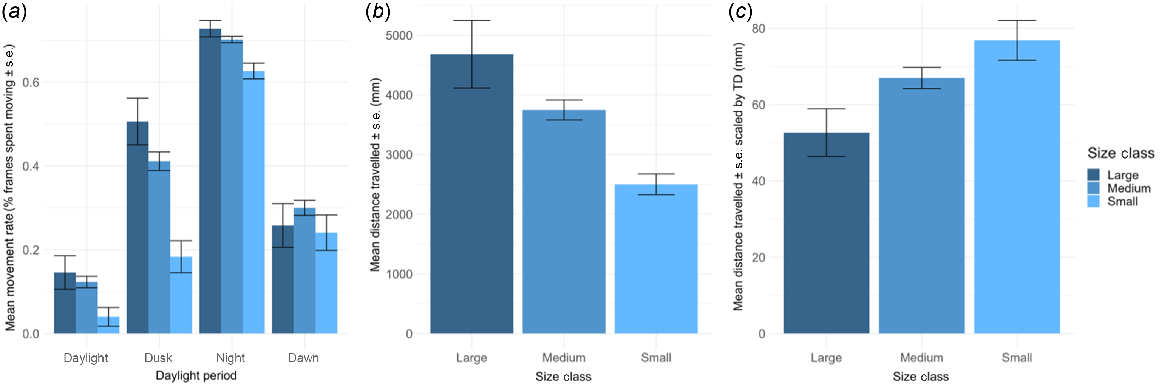
Characteristics | Size class | ANOVA | |||||
---|---|---|---|---|---|---|---|
Large | Medium | Small | d.f. | F | P | ||
Test diameter (TD, mm) | >77 | 39–77 | <39 | – | – | – | |
Total number of sea urchins tracked | 36 | 224 | 32 | – | – | – | |
Total number of sea urchins in FOV all night | 18 | 140 | 24 | – | – | – | |
Movement rate (percentage time moving) | |||||||
Daylight (19:00–07:00 hours, lmer) | 14.6 ± 4.0 | 12.3 ± 1.4 | 4.0 ± 2.2 | 220 | 2.77 | 0.065 | |
Dusk (20:00 hours, lm) | 50.6 ± 5.6 | 41.1 ± 2.2 | 18.3 ± 3.8* | 259 | 10.81 | <0.001 | |
Night (21:00–04:00 hours, lmer) | 72.8 ± 2.0 | 70.2 ± 0.8 | 62.7 ± 1.9 | 271 | 3.36 | 0.036 | |
Dawn (05:00–06:00 hours, lmer) | 25.8 ± 5.2 | 30.0 ± 1.8 | 24.1 ± 4.2 | 175 | 0.68 | 0.506 | |
Distance travelled (mm × 102) (lm) | 4.68 ± 0.6 | 3.74 ± 0.17 | 2.5 ± 0.17* | 179 | 7.05 | 0.001 | |
Distance travelled scaled to TD (mm) (lm) | 52.7 ± 6.23 | 67.0 ± 2.78 | 76.9 ± 5.20* | 179 | 3.05 | 0.050 | |
Displacement (mm × 102) (lm) | 0.58 ± 0.1 | 0.41 ± 0.04 | 0.28 ± 0.05 | 157 | 2.60 | 0.08 |
Where ANOVA results are presented, all numerator d.f. = 2. Asterisks represent result is significantly different from other means indicated by ANOVA and verified by a post hoc Tukey comparison. Models were either linear model (lm) where urchin ID was not included (no replicate urchin measurements included) or linear mixed effect model (lmer) when urchin was included as a random factor.
The interaction between urchin size and timing of movement may be influenced by variations in ambient light within the habitat, as suggested in Flukes et al. (2012). Our research revealed that smaller urchins exhibit a delayed increase in movement at dusk compared to larger size classes (Fig. 3a), meaning that their period of heightened movement is relatively shorter, perhaps due to lesser demand for food (Hart and Chia 1990). During the daytime, smaller urchins tend to seek refuge in crevices, whereas larger urchins can often be found on open rock areas. Consequently, if changes in ambient light serve as the trigger for urchin activity, it is possible that smaller urchins residing in dark crevices during daylight hours may not perceive these changes until later in the night, resulting in delayed onset of activity.
Distance travelled by the urchins overnight was lowest for small urchins, compared to medium and large size classes (Fig. 3b, Table 1, ANOVA: F(2,179) = 7.05, P = 0.001). Small urchins that remained in the FOV all night (n = 24) moved on average 2.5 ± 0.17 m per night (Fig. 3b) and had the lowest average displacement (0.3 m ± 0.05) from their starting point, but this was not significantly different to the displacement of other size classes (ANOVA: F(157,2) = 2.60, P = 0.08). In comparison, medium urchins (n = 140) moved on average 3.7 m ± 0.17 with a displacement value of 0.4 m ± 0.04 and large urchins (n = 18) 4.6 m ± 0.6 with displacement of 0.6 m ± 0.1. When scaled to urchin size, size did appear to slightly influence distance travelled (Fig 3c, Table 1, F(2, 179) = 3.05, P = 0.05). Post hoc analysis showed small urchins travelled further on average than large urchins (t(small–large) = 2.47, P = 0.04) but there was no difference between small and medium t(small–medium) = 1.42, P = 0.47), or medium and large (t(medium–large) = 1.82, P = 0.21). The distance travelled by urchins overnight has similar implications to their movement rates regarding their predation susceptibility. The more time away from, and further distance travelled from their crevice, the more opportunity there is for nocturnal predation. Since only the urchins that remained within FOV all night were used (50% large urchins, 63% medium and 75% small), there is potential for underestimation of movement data, particularly in the larger size class, which had a greater tendency to leave the FOV. Despite this, it is clear that all sizes of urchins, including smaller ones, leave their cryptic refugia and are vulnerable to predation overnight during periods of greater movement.
Temporal overlap of predators and prey
Examining the nocturnal movement of urchins provides insight into their susceptibility to predation during night-time hours, particularly of smaller, diurnally cryptic urchins. In a similar urchin species, Centrostephanus coronatus, nocturnal foraging behaviour is considered a predator-avoidance strategy which evolved in response to daytime predators (Nelson and Vance 1979). Similarly, the tendency of small C. rodgersii urchins to delay onset of movement later than other size classes is likely to be an evolutionary behavioural adaptation to avoid predation during daylight hours. By delaying their emergence, the smaller urchins potentially reduce the risk of being targeted by predators as the larger urchins are fed on first. Alternatively, the small urchins may benefit from the increased number of conspecifics emerging first, reducing their likelihood of predation by a ‘group protection’ effect.
The observed nocturnal increase in movement among small urchins could put them at higher risk of predation in Tasmania. The nocturnal emergence of smaller urchins directly overlaps with the heightened activity levels seen in lobsters overnight, particularly the activity of small and medium lobsters (Frusher et al. 2009). Laboratory studies have shown small lobsters do predate on small urchins (Ling et al. 2009; Smith et al. 2022), whereas field studies show high prevalence of urchin in small wild lobster diet (Smith et al. 2023). Based on this evidence and the results obtained about urchin temporal behaviour in this study, we conclude that small urchins are temporarily susceptible to predation by small lobsters (as well as other size classes) when highly active on open reef spaces throughout the hours of darkness. This has important implications for management of C. rodgersii with predators, and may switch the focus from more large predators to more predators of all sizes.
Conclusions
Small urchins were found to emerge from daytime cryptic refugia to be active on open reef spaces at night, which likely puts them at risk of nocturnal predation by southern rock lobster in their recently extended geographic range of Tasmania. The novelty of this finding relates to the fact that previously small urchins were considered to remain cryptic throughout the night, avoiding undue risk of predations from lobster. This has relevance to the wider ecosystem and implications for ecosystem-based fisheries management as the role of smaller lobsters in the predatory control of longspined sea urchins may be larger than first assumed. The importance of the smaller predators in the Tasmanian system has implications for management as, at present, only large lobsters are deemed important predators of C. rodgersii.
Data availability
Data used for this project are available at the IMAS Data Portal as ‘Longspined sea urchin size and movement metrics (data accompanying: “The risky nightlife of undersized urchins”)’ at https://doi.org/10.25959/7BHW-2D70.
References
Andrew NL, Underwood AJ (1989) Patterns of abundance of the sea urchin Centrostephanus rodgersii (Agassiz) on the central coast of New South Wales, Australia. Journal of Experimental Marine Biology and Ecology 131(1), 61-80.
| Crossref | Google Scholar |
Bates D, Mächler M, Bolker B, Walker S (2015) Fitting linear mixed-effects models using lme4. Journal of Statistical Software 67(1), 1-48.
| Crossref | Google Scholar |
Day J (2020) Urchin predation and marine park residency in the eastern rock lobster (Sagmariasus verreauxi): an initial assessment of its potential to control urchin populations. BMarSc(Hons) thesis, University of Wollongong, Wollongong, NSW, Austalia. Available at https://ro.uow.edu.au/cgi/viewcontent.cgi?article=1192&context=thsci
Department of Primary Industries, Parks, Water and Environment (2018) Tasmanian Rock Lobster Fishery: east coast stock rebuilding strategy 2013–2023. Technical report. Version: September 2018. (DPIPWE, Tasmanian Government) Available at https://fishing.tas.gov.au/Documents/East_Coast_Stock_Rebuilding_Strategy_Sept18.pdf
Filbee-Dexter K, Scheibling RE (2014) Sea urchin barrens as alternative stable states of collapsed kelp ecosystems. Marine Ecology Progress Series 495, 1-25.
| Crossref | Google Scholar |
Flukes EB, Johnson CR, Ling SD (2012) Forming sea urchin barrens from the inside out: an alternative pattern of overgrazing. Marine Ecology Progress Series 464, 179-194.
| Crossref | Google Scholar |
Frusher S, Buxton C, Barrett N, Tarbath D, Redd K, Semmens J, Pederson H, Valentine J, Guest M (2009) Towards integrated multi-species management of Australia’s SE reef fisheries: a Tasmanian example. Technical Report 2004/013. (Fisheries Research and Development Corporation (Australia) and the Tasmanian Aquaculture and Fisheries Institute) Available at https://www.imas.utas.edu.au/__data/assets/pdf_file/0007/743092/Towards-integrated-multi-species-management-of-Australias-SE-reef-fisheries-A-Tasmanian-example.pdf
Grolemund G, Wickham H (2011) Dates and times made easy with lubridate. Journal of Statistical Software 40(3), 1-25.
| Crossref | Google Scholar |
Hart LJ, Chia F-S (1990) Effect of food supply and body size on the foraging behavior of the burrowing sea urchin Echinometra mathaei (de Blainville). Journal of Experimental Marine Biology and Ecology 135, 99-108.
| Crossref | Google Scholar |
Hothorn T, Bretz F, Westfall P (2008) Simultaneous inference in general parametric models. Biometrical Journal 50(3), 346-363.
| Crossref | Google Scholar | PubMed |
Johnson C, Ling S, Ross J, Shepherd S, Miller K (2005) Establishment of the long-spined sea urchin (Centrostephanus rodgersii) in Tasmania: first assessment of potential threats to fisheries. Technical report 2001/044. (Fisheries Research and Development Corporation: Hobart, Tas., Australia) Available at https://www.imas.utas.edu.au/__data/assets/pdf_file/0018/1141335/Long-Spined-Sea-Urchin_Johnson-Ling-et-al-2005.pdf
Ling SD (2008) Range expansion of a habitat-modifying species leads to loss of taxonomic diversity: a new and impoverished reef state. Oecologia 156(4), 883-894.
| Crossref | Google Scholar | PubMed |
Ling SD, Johnson CR (2009) Population dynamics of an ecologically important range-extender: kelp beds versus sea urchin barrens. Marine Ecology Progress Series 374, 113-125.
| Crossref | Google Scholar |
Ling SD, Johnson CR (2012) Marine reserves reduce risk of climate-driven phase shift by reinstating size- and habitat-specific trophic interactions. Ecological Applications 22(4), 1232-1245.
| Crossref | Google Scholar | PubMed |
Ling SD, Keane JP (2018) Resurvey of the longspined sea urchin (Centrostephanus rodgersii) and associated barren reef in Tasmania. Technical report. (Institute for Marine and Antarctic Studies, University of Tasmania: Hobart, Tas., Australia) Available at https://www.imas.utas.edu.au/__data/assets/pdf_file/0005/1176026/129569-Resurvey-of-the-Longspined-Sea-Urchin-Centrostephanus-rodgersii-and-associated-barren-reef-in-Tasmania.pdf
Ling SD, Keane JP (2021) Decadal resurvey of long-term lobster experimental sites to inform Centrostephanus control. Technical Report AIRF2019 08. (Institute for Marine and Antarctic Studies. University of Tasmania: Tasmania, Tas., Australia) Available at https://www.imas.utas.edu.au/__data/assets/pdf_file/0008/1556522/Centro_lobster_exp_site_resurvey_final_report.pdf
Ling SD, Johnson CR, Frusher SD, Ridgway KR (2009) Overfishing reduces resilience of kelp beds to climate-driven catastrophic phase shift. Proceedings of the National Academy of Sciences 106(52), 22341-22345.
| Crossref | Google Scholar |
Ling SD, Scheibling RE, Rassweiler A, Johnson CR, Shears N, Connell SD, Salomon AK, Norderhaug KM, Pérez-Matus A, Hernández JC, Clemente S, Blamey LK, Hereu B, Ballesteros E, Sala E, Garrabou J, Cebrian E, Zabala M, Fujita D, Johnson LE (2015) Global regime shift dynamics of catastrophic sea urchin overgrazing. Philosophical Transactions of the Royal Society of London – B. Biological Sciences 370(1659), 20130269.
| Crossref | Google Scholar |
MacDiarmid AB, Hickey B, Maller RA (1991) Daily movement patterns of the spiny lobster Jasus edwardsii (Hutton) on a shallow reef in northern New Zealand. Journal of Experimental Marine Biology and Ecology 147(2), 185-205.
| Crossref | Google Scholar |
Miller KI, Blain CO, Shears NT (2022) Sea urchin removal as a tool for macroalgal restoration: a review on removing “the spiny enemies”. Frontiers in Marine Science 9, 831001.
| Crossref | Google Scholar |
Nelson BV, Vance RR (1979) Diel foraging patterns of the sea urchin Centrostephanus coronatus as a predator avoidance strategy. Marine Biology 51(3), 251-258.
| Crossref | Google Scholar |
Redd KS, Jarman SN, Frusher SD, Johnson CR (2008) A molecular approach to identify prey of the southern rock lobster. Bulletin of Entomological Research 98(3), 233-238.
| Crossref | Google Scholar | PubMed |
Redd KS, Ling SD, Frusher SD, Jarman S, Johnson CR (2014) Using molecular prey detection to quantify rock lobster predation on barrens-forming sea urchins. Molecular Ecology 23(15), 3849-3869.
| Crossref | Google Scholar | PubMed |
Schneider CA, Rasband WS, Eliceiri KW (2012) NIH Image to ImageJ: 25 years of image analysis. Nature Methods 9(7), 671-675.
| Crossref | Google Scholar |
Smith JE, Keane J, Mundy C, Gardner C, Oellermann M (2022) Spiny lobsters prefer native prey over range-extending invasive urchins. ICES Journal of Marine Science 79(4), 1353-1362.
| Crossref | Google Scholar |
Smith JE, Keane J, Oellermann M, Mundy C, Gardner C (2023) Lobster predation on barren-forming sea urchins is more prevalent in habitats where small urchins are common: a multi-method diet analysis. Marine and Freshwater Research 74(18), 1493-1505.
| Crossref | Google Scholar |
Tegner MJ, Levin LA (1983) Spiny lobsters and sea urchins: analysis of a predator-prey interaction. Journal of Experimental Marine Biology and Ecology 73(2), 125-150.
| Crossref | Google Scholar |
Wickham H, Averick M, Bryan J, Chang W, McGowan LD, François R, Grolemund G, Hayes A, Henry L, Hester J, Kuhn M, Pedersen TL, Miller E, Bache SM, Müller K, Ooms J, Robinson D, Seidel DP, Spinu V, Takahashi K, Vaughan D, Wilke C, Woo K, Yutani H (2019) Welcome to the tidyverse. Journal of Open Source Software 4(43), 1686.
| Crossref | Google Scholar |
Williams BG, Dean IC (1989) Timing of locomotor activity in the New Zealand rock lobster, Jasus edwardsii. New Zealand Journal of Marine and Freshwater Research 23(2), 215-224.
| Crossref | Google Scholar |