Impacts of VIE tagging and Rhodamine B immersion staining on two measures of performance for a small-bodied fish
P. A. Franklin

A
B
C
D Present address:
Abstract
Passage efficiency is an important metric for quantifying the success of fish-passage remediation. Mark–recapture methods are typically employed to estimate fish-passage efficiency. Although biotelemetry methods have become a benchmark standard for such studies, they remain unfeasible for many small-bodies species because of the excessive size of electronic tags.
This study compares two commonly deployed marking methods that are potentially suitable for estimating passage efficiency for small-bodied species, namely, visible implant elastomer (VIE) tagging and batch immersion staining with Rhodamine B.
The critical swimming speeds and passage efficiency at a remediated culvert of Galaxias maculatus were compared for VIE-tagged, Rhodamine B-stained and control treatments.
Both critical swimming speeds and passage efficiency at the culvert were significantly lower in VIE-tagged Galaxias maculatus than in both control fish and fish stained with Rhodamine B.
Rhodamine B may be a suitable batch immersion stain for short-term (<4 day), non-destructive mark–recapture studies in small-bodied fishes, but VIE-tagging methods may result in underestimation of passage efficiency.
It is important to evaluate the impact of marking techniques on the outcomes of mark–recapture studies to ensure that estimates of passage efficiency are accurate.
Keywords: fish migration, fish passage, Galaxias maculatus, immersion staining, mark–recapture, passage efficiency, Rhodamine B, visible implant elastomer.
Introduction
Restoration of river connectivity is a key goal for protecting and enhancing diadromous fish communities (Thieme et al. 2023). However, the effectiveness of many fish-passage solutions remains poorly understood (Silva et al. 2018; Hershey 2021). Biotelemetry has been held up as the gold-standard for evaluating the performance of different fish-passage solutions and for quantifying fish-passage efficiency (Bunt et al. 2012; Noonan et al. 2012; Hershey 2021). Although the information that can be captured from biotelemetry studies is invaluable and ever improving (Cooke et al. 2013; Hussey et al. 2015), applying biotelemetry methods is not physically possible for some species and life stages characterised by small body sizes (Watson et al. 2019).
Amphidromous fishes undertake their primary upstream migration as small-bodied (15–60-mm total length, TL) juveniles and are a key component of the fish communities of many island nations across the globe (Franklin and Gee 2019). Owing to their small size at migration, these species can be highly susceptible to the impacts of instream infrastructure on river connectivity, with vertical drops of as little as 10 cm shown to severely impede the upstream passage of some species (Baker 2003; Jones et al. 2021). Owing to their susceptibility to river fragmentation, evaluating the effectiveness of different fish-passage solutions for these species is essential, yet the use of biotelemetry is infeasible.
As with larger fishes, a wide range of methods has been applied to evaluate the effectiveness of different fish-passage solutions for smaller-bodied fishes. Many of these focus on trapping of fish entering or exiting fishways or other instream structures (e.g. culverts), or on surveys comparing fish community composition upstream and downstream of structures, or before and after remediation (Rolls et al. 2013). For example, Marsden and Stuart (2019) evaluated the effectiveness of three different fishway types for small-bodied species in Australia by using paired upstream and downstream trapping methods. They were able to demonstrate that, for empire gudgeons (Hypseleotris compressa), there was no difference in size between those entering and those exiting any of the fishways, but for bony herring (Nematalosa erebi) there was a significant difference in the sizes of fish entering and those exiting two of the fishways. However, because of the schooling nature of the target species (meaning that the abundance of fish passing can be highly temporally variable), they were unable to compare the abundance of fish entering versus fish exiting the fishway and, hence, estimate passage efficiency in the traditional sense. Similarly, Rolls et al. (2018) compared fish community composition and size structure between downstream control reaches and at the exit of two fishways by using passive trapping methods. They found significant differences in community composition, with a range of small-bodied species being absent from the samples collected at the fishway exits.
Unfortunately, the trapping methods highlighted above do not alone allow for accurate quantification of passage efficiency, that is, an estimation of the proportion of fish arriving at the structure that successfully pass. Although not being the only way that fish-passage effectiveness can be measured, passage efficiency is an important metric for understanding the relative performance of different solutions and is frequently used as a performance measure against which fish-passage projects are evaluated (Bunt et al. 2012; Wilkes et al. 2018; Hershey 2021).
Mark–recapture methods, whereby fish are marked somehow, liberated, and subsequently recaptured or observed later, offer a means of estimating the proportion of a sample population that passes a fishway or instream structure. Although contemporary biotelemetry technologies allow for a wide range of information to be captured about fish behaviour and movements from the tags (Hussey et al. 2015; Lennox et al. 2017), in essence biotelemetry studies are a form of the mark and recapture method. The tags act as unique identifiers for individual fish and allow collection of accurate information on the timing and extent of fish movements. As such, biotelemetry has become the go to approach for quantifying passage efficiency at fishways (Bunt et al. 2012; Noonan et al. 2012; Hershey 2021). Although increasing refinements and miniaturisation of the technology are enabling biotelemetry methods to be applied to species with increasingly smaller body sizes (Tummers et al. 2016; Baker et al. 2017; Watson et al. 2019), they remain impractical for many small-bodied species (Franklin and Gee 2019; Sandford et al. 2019). For example, Watson et al. (2019) showed 100% mortality rates for two small-bodied species (Pseudomugil signifier and Macquaria ambigua) following tagging with microPIT tags. Consequently, alternative marking methods are required for estimation of passage efficiency in these species.
Two examples of such marking methods that have been identified as having potential application for small-bodied species are visible implant elastomer (VIE) tags and immersion staining. VIE tags consist of a fluorescent liquid elastomer that is injected into the tissue of the fish that sets to form a longer-term biocompatible mark. Immersion staining involves total body immersion of the fish in an aqueous dye solution to impart a dye to the fish. In this study we compared VIE tags and immersion staining for their suitability for undertaking mark–recapture studies of a small-bodied fish to estimate passage efficiency at instream structures. To do this, we compared critical swimming speeds (Ucrit) and field-based estimates of passage efficiency through a culvert for unmarked, VIE-tagged, and immersion-stained Galaxias maculatus individuals, a small-bodied amphidromous species with a widespread distribution in the temperate Southern Hemisphere.
Materials and methods
Fish capture and husbandry
Juvenile G. maculatus individuals were captured during their upstream migration by using either fine-mesh rigid frame whitebait traps or Gee-minnow traps from the Waikato River catchment in the North Island of New Zealand. Following capture, fish were transferred to aerated containers and transported either to the NIWA Hamilton laboratory (for the Ucrit trials) or directly to the study site on Bankwood Stream, Hamilton (for the passage-efficiency trials).
On arrival at the laboratory, fish were held in a temperature-controlled room on a 12:12 h day:night cycle. Fish were quarantined in 60-L tanks with a salinity of 6 to prevent disease and transferred to 60 L of dechlorinated water tanks after 1 week. These tanks were kept on a recirculating water system at a temperature of 15 ± 0.5°C. Fish were fed bloodworms every other day and underwent a 24-h fasting period before trials to ensure a post-absorptive state. Fish used in the passage-efficiency trials were transferred to flow-through live bins and held within Bankwood Stream under ambient conditions prior to tagging.
All experimental trials for this study were conducted with approval to manipulate live animals for research by the NIWA Animal Ethics Committee (AEC204), in accordance with the requirements of Section 83 of the New Zealand Animal Welfare Act 1999.
Fish tagging
Fish to be used for the Ucrit trials were tagged or stained within the laboratory, whereas fish used for the passage-efficiency trials were tagged or stained on site. Fish for the Ucrit trials were captured using hand-nets and randomly allocated to the three different treatment groups (VIE-tagged, stained and control) to try and reduce the potential confounding impact of capture order. Fish in the first field-based passage-efficiency trial were all VIE tagged, whereas fish for the second field-based passage-efficiency trial were randomly allocated between the stained and control groups. All fish to be marked with VIE tags (Northwest Marine Technology, Anacortes, WA, USA) were first sedated using Aqui-S (Aqui-S New Zealand Ltd, Lower Hutt, New Zealand), then fish length was recorded and the tags were injected into the dorsal surface of the fish. Staining was achieved by batch immersion of the fish in a solution of Rhodamine B (0.2 g L−1; Sigma–Aldrich, Darmstadt, Germany). To optimise survival and stain retention, aquarium salts were added to the solutions to produce a salinity of ~15. The staining solution was aerated and, for the fish that were stained on site, ice was added to the solution as necessary to maintain the same temperature as the stream. The fish were removed from the stain after 2 h by using a dip net. To allow for recovery, all tagged and stained fish were subsequently transferred either to the laboratory holding tanks (for the Ucrit trials) or flow-through live bins held within Bankwood Stream (for the passage-efficiency trials) for a minimum of 24 h prior to trials commencing. A randomly selected subgroup of VIE-tagged fish were held for 21 days prior to undertaking critical swimming tests to evaluate the influence of time since tagging. The control group of unmarked fish were retained in separate holding tanks to act as a control for the effect of the tagging–marking procedure (Table 1).
Marking method | n | Mean total length (mm) | s.d. (mm) | Minimum total length (mm) | Maximum total length (mm) | |
---|---|---|---|---|---|---|
Control | 19 | 44.0 | ±3.85 | 37 | 50 | |
Rhodamine B stain | 19 | 48.6 | ±3.45 | 42 | 58 | |
VIE tag | 19 | 56.2 | ±4.65 | 48 | 67 | |
VIE tag (21 days) | 9 | 50.2 | ±2.64 | 47 | 55 |
Critical swimming speeds
Critical swimming-speed trials were conducted in a 10-L Loligo Systems Steffensen-type swim tunnel (Loligo Systems, Viborg, Denmark), following the methods of Crawford et al. (2023). Briefly, water temperature in the swim tunnel was maintained at 15 ± 0.5°C and dissolved oxygen concentrations were maintained throughout the trials at a mean of 98.0 ± 0.9% saturation. At the beginning of each trial, individual fish were introduced into the swim tunnel and acclimated for 30 min at 0.5 bodylengths s−1. The water velocity in the swim tunnel was then incrementally raised by 1 bodylength s−1 every 5 min. A trial was concluded once a fish displayed signs of fatigue, characterised by resting against the mesh at the back of the tunnel for a continuous duration of 3 s or for a cumulative total of 10 s within a 30-s timeframe. At the end of the trial, fish were rested in the tunnel with no velocity for 20 min before being returned to the holding tank to ensure recovery. Each trial was conducted independently, without any fish being re-used and all fish were tested once (Table 1).
Critical swimming speed was calculated following Brett (1964) as:
where Ucrit is the critical swimming speed (bodylengths s−1), Uf−1 is the penultimate speed the fish experienced (bodylengths s−1), ΔU represents the speed increase (1 bodylength s−1), tf is the duration of time the fish swam at the highest speed (s; 0 ≤ tf < Δt), Δt represents the time increment (300 s).
Culvert passage efficiency
The passage-efficiency trials were conducted at the River Road culvert on Bankwood Stream in Hamilton, New Zealand. It is a 73.8-m-long single-barrel 1.5-m diameter concrete culvert with a mean gradient of 0.8° (range 0.3–2.55°). The culvert has been retrofitted with a rock-ramp fishway and spoiler baffles to improve fish passage as described in Franklin and Bartels (2012).
Passage trials were initially conducted using VIE-tagged fish and reported in Franklin and Bartels (2012). The initial trial was undertaken during the migratory period for G. maculatus in December 2009 under base-flow conditions. In total, 177 VIE-tagged inanga individuals were released in the receiving pool at the outlet of the culvert, which had been sealed with fine-mesh netting to prevent fish from escaping downstream. A fine-mesh rigid whitebait trap was then set and sealed at the top of the culvert to capture all fish successfully passing the culvert. The trial was run for 24 h to account for any diel variations in migratory motivation. Passage success was defined as being present in the upstream trap at the completion of the trial. The experimental design of Franklin and Bartels (2012) was subsequently replicated at the same site under equivalent base-flow conditions in March 2015 using fish batch stained with Rhodamine B and a control group of unmarked fish (Table 2). The second trial was run for 48 h, with the number of successful fish (i.e. those in the upstream trap) quantified after 24 and 48 h.
Statistical analyses
Analysis of covariance (ANCOVA) was used to test for differences in mean critical swimming speed (Ucrit) between the three treatments. Marking treatment was used as a categorical predictor variable with three levels (control, stained, VIE) and fish total length was used as a continuous predictor variable. Individual critical swimming speeds were used as the response variable. To determine the model of best fit, a likelihood ratio test (LRT) was used, starting with a fully saturated model including interactions. A post hoc Tukey’s HSD test was used to determine which treatments had statistically different critical swimming speeds.
Culvert passage success was compared among treatments by using a binomial generalised linear model (GLM) using the glm() function and a logit link in the base package of R. Individual passage success (pass or fail) was used as the response variable. Marking treatment and individual fish lengths were again used as predictor variables. A fully saturated model including interactions was fitted and stepwise selection was used to remove non-significant predictors. All statistical analyses were conducted using the R statistical computing software (ver. 4.3.1, R Foundation for Statistical Computing, Vienna, Austria, see https://www.r-project.org/).
Results
Critical swimming speeds
Mean critical swimming speeds (±s.d.) of the control, stained and VIE-tagged G. maculatus individuals were respectively 6.45 ± 2.85, 7.08 ± 2.67 and 3.14 ± 3.70 bodylengths s−1 (Fig. 1). For the fish 21-days post-VIE tagging, mean critical swimming speed (±s.d.) was 2.98 ± 2.23 bodylengths s−1 Fish length did not have a statistically significant effect on critical swimming speed (F = 0.047, P = 0.829), but there was a significant difference in critical swimming speed among treatments (F = 7.779, P < 0.001). Mean Ucrit of the VIE-tagged fish was significantly lower than that of both the control (P = 0.007) and stained fish (P < 0.001), but there was no statistically significant difference between the control and stained fish (P = 0.918). Swimming speeds of the 21-day post-VIE tagging fish were significantly different from those of the control fish (P = 0.030), but not statistically different from those of the VIE-tagged fish tested 24 h after tagging (P = 0.999).
Critical swimming speeds of non-marked and marked G. maculatus individuals. The data points correspond to individual fish within each treatment group. The centre bar displays the median, whereas the outer edges of the box display the interquartile range. The whiskers show 1.5 times the interquartile range.
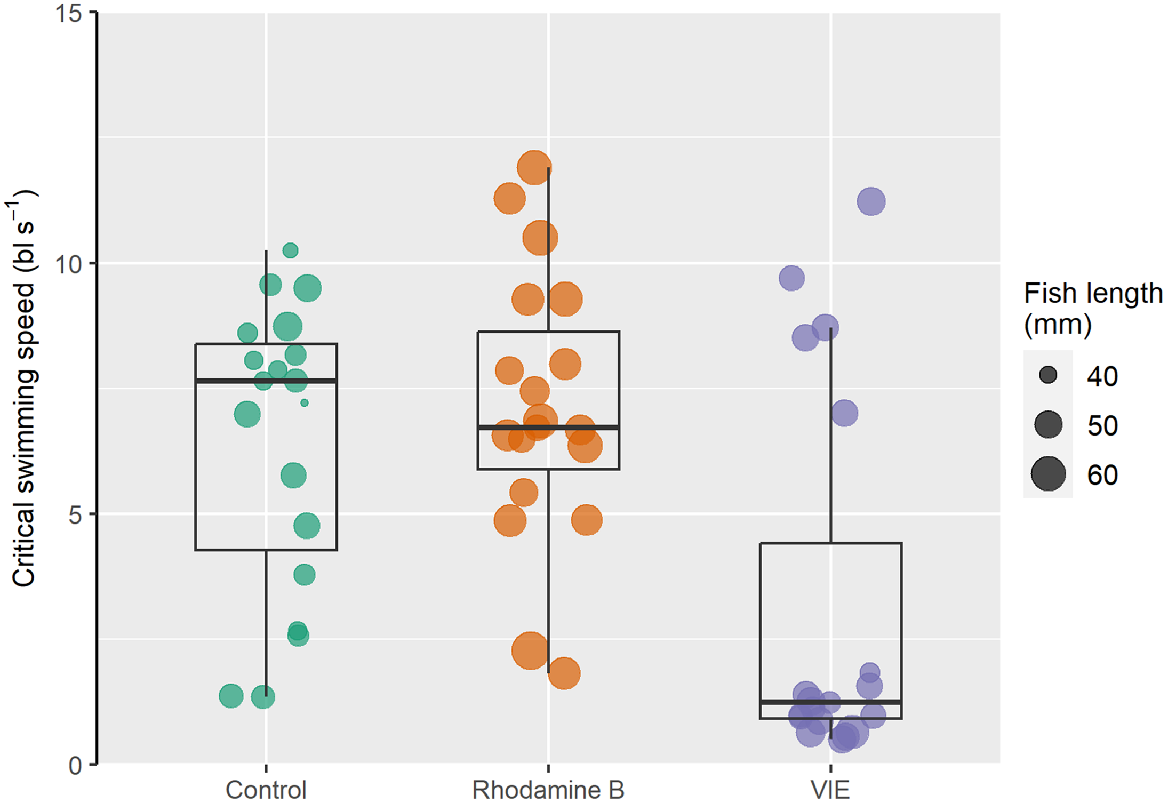
Culvert passage efficiency
Overall passage success after 24 h was 27.0% for the control fish, 28.0% for the stained fish and 6.2% for the VIE-tagged fish (Fig. 2). Fish length had no statistically significant effect on passage success (P = 0.440) and was removed from the GLM model. There was no statistically significant difference in passage success between the control and stained fish (P = 0.823), but passage success was significantly lower for the VIE-tagged fish than both the control and stained fish (P < 0.001). For the control and stained fish, the trial was extended to 48 h, after which overall passage success had increased to 33.5% for both treatments.
Discussion
Passage efficiency is an important metric for evaluating and comparing the effectiveness of different fish passage solutions. In this study, we have demonstrated that marking method can have a significant impact on assessments of passage efficiency for a small-bodied fish, G. maculatus, using mark–recapture methods. Passage efficiency of G. maculatus stained with Rhodamine B was equivalent to that of non-marked control fish. However, passage efficiency was substantially lower for G. maculatus marked with VIE tags than for fish in the control group. These differences in passage efficiency were also apparent in critical swimming speeds, with no difference in Ucrit between G. maculatus stained with Rhodamine B and control fish, but significantly lower swimming speeds being observed for the fish marked with VIE tags. What is more, the impacts of the VIE tags on critical swimming speed were still present 21 days post-tagging. In light of these results, it is clear that the passage-efficiency estimates previously presented by Franklin and Bartels (2012) by using VIE-tagged fish for this remediated culvert are an underestimation of true passage efficiency for G. maculatus at this site.
VIE tags have often been identified as being suitable for individual-based or batch marking and tracking of fish and other aquatic organisms, although numerous potential shortcomings have also been identified with respect to tag misidentification, impacts on survival, and alterations to behaviour (Jungwirth et al. 2019; Moore and Brewer 2021). We have demonstrated negative effects of VIE tagging on fish swimming speeds compared with control fish that are apparently translated into poorer fish-passage performance in a field-based setting for G. maculatus. Although a range of studies have evaluated the impacts of VIE tagging on growth and survival of different species (e.g. Astorga et al. 2005; Bolland et al. 2009; Moore and Brewer 2021), and these generally show minimal or no negative effects, there appear to be few studies that have addressed the potential impacts on locomotive capabilities of fish. Imbert et al. (2007) looked at the impacts of VIE tags on locomotive behaviour of juvenile European eels (Anguilla anguilla) and found no difference between tagged and untagged individuals. However, the water velocities within their test flume were low (0.09 m s−1) compared with elver swimming capabilities (sustained and prolonged swimming threshold ~0.3 m s−1; McCleave 1980; Vezza et al. 2020) and so may not have presented a significant physical challenge for the fish. Other studies that have evaluated fish swimming speeds and passage performance by using small-bodied VIE-tagged fish (e.g. Jones et al. 2020; Jones et al. 2021) have not reported comparisons with untagged (i.e. control) fish. Given our results, we suggest that in future studies it is essential that a control group of untagged fish is included as part of trials so that any potential tagging effects that may arise can be accounted for.
In contrast to the VIE tagged fish, we observed no significant effect on either critical swimming speed or passage success of fish batch stained using Rhodamine B when compared with control fish. A weakness of batch staining compared with some alternative marking methods is that it does not allow for individual identification of fish. However, in the context of determining overall passage efficiency at instream barriers, batch marking offers an effective solution. Another limitation of staining is the duration for which the stain is visible. In our experience, we have found that stained fish can be reliably identified for 3 days post-staining, but by Day 4 it can become more difficult to confidently identify all stained fish. Consequently, applications should be limited to short-term studies that can be completed in <4 days. G. maculatus is scaleless and at the larval life-stage is translucent, which may make it particularly suitable for staining. However, Rhodamine B has previously also been found to be an effective immersion marker for the scaled Gambusia affinis (O’Grady and Hoy 1972). Where longer tag-retention periods are required, Rhodamine B staining will not be suitable. Fluorochrome dyes (e.g. calcein, alizarin red) have been shown to produce more persistent (>100 days) detectable marks on bony structures in a range of fishes (Lü et al. 2015; Kullmann et al. 2018; Afentoulis et al. 2022; Gaillard et al. 2022), put poor survival has been reported for some small-bodied species during and following the dying process (Afentoulis et al. 2022) and detection of the marks is generally destructive.
Marking fish with VIE tags requires considerably more handling of the fish than do the batch immersion methods. Furtbauer et al. (2015), for example, reported notable post-VIE tagging physiological stress responses for three-spined stickleback Gasterosteus aculeatus. We hypothesise that the greater handling of the fish required for VIE tagging imparts higher stresses than does immersion staining that, at least over the short term, imposes a physiological burden on fish that affects swimming capabilities. This may make VIE tagging less suitable for short-term (hours to days) studies using small-bodied fish species than the immersion staining method tested here. However, we also found that critical swimming speeds remained impaired even 21 days post-VIE tagging, suggesting potential prolonged impacts. These effects need to be explored further to understand the potential implications for studies using VIE tags.
Conclusions
We have demonstrated that VIE tagging had a significant negative effect on the critical swimming speed of the small-bodied G. maculatus and that this negative effect is sustained 3 weeks post-tagging. Furthermore, the negative effect of VIE tagging on swimming speeds was also reflected in a measurably lower passage success past a remediated culvert. Consequently, the passage-efficiency results for the remediated culvert previously presented by Franklin and Bartels (2012) are an underestimation of true passage efficiency for G. maculatus at this site.
The results of marking studies may be incorrect if the marks and or associated marking procedures change the behaviour, condition or survival of fish compared with unmarked individuals (Cooke et al. 2013; Sandford et al. 2019). Consequently, it is important that different marking methods are evaluated to determine their suitability for different species and applications. Biotelemetry tagging methods remain ineffective for some small-bodied fishes, meaning that alternative methods are required to evaluate fish-passage efficiency. On the basis of our results, batch immersion staining using Rhodamine B is a more suitable marking method than VIE tagging for short-term studies of G. maculatus passage efficiency.
Data availability
The data that support this study will be shared upon reasonable request to the corresponding author.
Declaration of funding
This work was funded by the New Zealand Ministry for Business Innovation and Employment contract CO1X1002, Waikato River Authority contract WRA 12-055 (P. A. Franklin, C. F. Baker) and a University of Waikato doctoral scholarship (R. Crawford).
References
Afentoulis V, Kalmbach A, Hung TC, Rahman MM, Ellison L, Miranda J (2022) Vital dye immersion evaluations with juvenile delta smelt. Aquaculture, Fish and Fisheries 3, 102-111.
| Crossref | Google Scholar |
Astorga N, Afonso JM, Zamorano MJ, Montero D, Oliva V, Fernandez H, Izquierdo MS (2005) Evaluation of visible implant elastomer tags for tagging juvenile gilthead seabream (Sparus auratus L.); effects on growth, mortality, handling time and tag loss. Aquaculture Research 36, 733-738.
| Crossref | Google Scholar |
Baker CF (2003) Effect of fall height and notch shape on the passage of inanga (Galaxias maculatus) and common bullies (Gobiomorphus cotidianus) over an experimental weir. New Zealand Journal of Marine and Freshwater Research 37, 283-290.
| Crossref | Google Scholar |
Baker CF, Reeve K, Baars D, Jellyman D, Franklin P (2017) Efficacy of 12-mm half-duplex passive integrated transponder tags in monitoring fish movements through stationary antenna systems. North American Journal of Fisheries Management 37, 1289-1298.
| Crossref | Google Scholar |
Bolland JD, Cowx IG, Lucas MC (2009) Evaluation of VIE and PIT tagging methods for juvenile cyprinid fishes. Journal of Applied Ichthyology 25, 381-386.
| Crossref | Google Scholar |
Brett JR (1964) The respiratory metabolism and swimming performance of young sockeye salmon. Journal of the Fisheries Research Board of Canada 21, 1183-1226.
| Crossref | Google Scholar |
Bunt CM, Castro-Santos T, Haro A (2012) Performance of fish passage structures at upstream barriers to migration. River Research and Applications 28, 457-478.
| Crossref | Google Scholar |
Cooke SJ, Midwood JD, Thiem JD, Klimley P, Lucas MC, Thorstad EB, Eiler J, Holbrook C, Ebner BC (2013) Tracking animals in freshwater with electronic tags: past, present and future. Animal Biotelemetry 1, 5.
| Crossref | Google Scholar |
Crawford R, Gee E, Dupont D, Hicks B, Franklin P (2023) No difference between critical and sprint swimming speeds for two galaxiid species. Journal of Fish Biology 102, 1141-1148.
| Crossref | Google Scholar | PubMed |
Franklin PA, Bartels B (2012) Restoring connectivity for migratory native fish in a New Zealand stream: effectiveness of retrofitting a pipe culvert. Aquatic Conservation: Marine and Freshwater Ecosystems 22, 489-497.
| Crossref | Google Scholar |
Franklin P, Gee E (2019) Living in an amphidromous world: perspectives on the management of fish passage from an island nation. Aquatic Conservation: Marine and Freshwater Ecosystems 29, 1424-1437.
| Crossref | Google Scholar |
Furtbauer I, King AJ, Heistermann M (2015) Visible implant elastomer (VIE) tagging and simulated predation risk elicit similar physiological stress responses in three-spined stickleback Gasterosteus aculeatus. Journal of Fish Biology 86, 1644-1649.
| Crossref | Google Scholar | PubMed |
Gaillard M, Parlanti E, Sourzac M, Couillaud F, Genevois C, Boutry S, Rigaud C, Daverat F (2022) New insights into detecting alizarin from autofluorescence in marked glass eels. Scientific Reports 12, 15985.
| Crossref | Google Scholar | PubMed |
Hershey H (2021) Updating the consensus on fishway efficiency: a meta-analysis. Fish and Fisheries 22, 735-748.
| Crossref | Google Scholar |
Hussey NE, Kessel ST, Aarestrup K, Cooke SJ, Cowley PD, Fisk AT, Harcourt RG, Holland KN, Iverson SJ, Kocik JF, Mills Flemming JE, Whoriskey FG (2015) Aquatic animal telemetry: a panoramic window into the underwater world. Science 348, 1255642.
| Crossref | Google Scholar | PubMed |
Imbert H, Beaulaton L, Rigaud C, Elie P (2007) Evaluation of visible implant elastomer as a method for tagging small European eels. Journal of Fish Biology 71, 1546-1554.
| Crossref | Google Scholar |
Jones PE, Svendsen JC, Börger L, Champneys T, Consuegra S, Jones JAH, Garcia de Leaniz C (2020) One size does not fit all: inter- and intraspecific variation in the swimming performance of contrasting freshwater fish. Conservation Physiology 8, coaa126.
| Crossref | Google Scholar |
Jones PE, Champneys T, Vevers J, Börger L, Svendsen JC, Consuegra S, Jones J, Garcia de Leaniz C (2021) Selective effects of small barriers on river-resident fish. Journal of Applied Ecology 58, 1487-1498.
| Crossref | Google Scholar |
Jungwirth A, Balzarini V, Zöttl M, Salzmann A, Taborsky M, Frommen JG (2019) Long-term individual marking of small freshwater fish: the utility of visual implant elastomer tags. Behavioral Ecology and Sociobiology 73, 49.
| Crossref | Google Scholar |
Kullmann B, Hempel M, Thiel R (2018) Chemical marking of European glass eels Anguilla anguilla with alizarin red S and in combination with strontium: in situ evaluation of short-term salinity effects on survival and efficient mass-marking. Journal of Fish Biology 92, 203-213.
| Crossref | Google Scholar | PubMed |
Lennox RJ, Aarestrup K, Cooke SJ, Cowley PD, Deng ZD, Fisk AT, Harcourt RG, Heupel M, Hinch SG, Holland KN, Hussey NE, Iverson SJ, Kessel ST, Kocik JF, Lucas MC, Flemming JM, Nguyen VM, Stokesbury MJW, Vagle S, VanderZwaag DL, Whoriskey FG, Young N (2017) Envisioning the future of aquatic animal tracking: technology, science, and application. BioScience 67, 884-896.
| Crossref | Google Scholar |
Lü HJ, Fu M, Xi D, Yao WZ, Su SQ, Wu ZL (2015) Experimental evaluation of using calcein and alizarin red S for immersion marking of bighead carp Aristichthys nobilis (Richardson, 1845) to assess growth and identification of marks in otoliths, scales and fin rays. Journal of Applied Ichthyology 31, 665-674.
| Crossref | Google Scholar |
Marsden T, Stuart I (2019) Fish passage developments for small-bodied tropical fish: field case-studies lead to technology improvements. Journal of Ecohydraulics 4, 14-26.
| Crossref | Google Scholar |
McCleave JD (1980) Swimming performance of European eel (Anguilla anguilla (L.)) elvers. Journal of Fish Biology 16, 445-452.
| Crossref | Google Scholar |
Moore DM, Brewer SK (2021) Evaluation of visual implant elastomer, PIT, and p-chip tagging methods in a small-bodied minnow species. North American Journal of Fisheries Management 41, 1066-1078.
| Crossref | Google Scholar |
Noonan MJ, Grant JWA, Jackson CD (2012) A quantitative assessment of fish passage efficiency. Fish and Fisheries 13, 450-464.
| Crossref | Google Scholar |
O’Grady JJ, Hoy JB (1972) Rhodamine B and other stains as markers for the mosquito fish, Gambusia affinis. Journal of Medical Entomology 9, 571-574.
| Crossref | Google Scholar | PubMed |
Rolls RJ, Ellison T, Faggotter S, Roberts DT (2013) Consequences of connectivity alteration on riverine fish assemblages: potential opportunities to overcome constraints in applying conventional monitoring designs. Aquatic Conservation: Marine and Freshwater Ecosystems 23, 624-640.
| Crossref | Google Scholar |
Rolls RJ, Faggotter SJ, Roberts DT, Burford MA (2018) Simultaneous assessment of two passage facilities for maintaining hydrological connectivity for subtropical coastal riverine fish. Ecological Engineering 124, 77-87.
| Crossref | Google Scholar |
Sandford M, Castillo G, Hung TC (2019) A review of fish identification methods applied on small fish. Reviews in Aquaculture 12, 542-554.
| Crossref | Google Scholar |
Silva AT, Lucas MC, Castro-Santos T, Katopodis C, Baumgartner LJ, Thiem JD, Aarestrup K, Pompeu PS, O’Brien GC, Braun DC, Burnett NJ, Zhu DZ, Fjeldstad H-P, Forseth T, Rajaratnam N, Williams JG, Cooke SJ (2018) The future of fish passage science, engineering, and practice. Fish and Fisheries 19, 340-362.
| Crossref | Google Scholar |
Thieme M, Birnie-Gauvin K, Opperman JJ, Franklin PA, Richter H, Baumgartner L, Ning N, Vu AV, Brink K, Sakala M, O’Brien GC, Petersen R, Tongchai P, Cooke SJ (2023) Measures to safeguard and restore river connectivity. Environmental Reviews [Published online early 23 August 2023].
| Crossref | Google Scholar |
Tummers JS, Hudson S, Lucas MC (2016) Evaluating the effectiveness of restoring longitudinal connectivity for stream fish communities: towards a more holistic approach. Science of the Total Environment 569, 850-860.
| Crossref | Google Scholar | PubMed |
Vezza P, Libardoni F, Manes C, Tsuzaki T, Bertoldi W, Kemp PS (2020) Rethinking swimming performance tests for bottom-dwelling fish: the case of European glass eel (Anguilla anguilla). Scientific Reports 10, 16416.
| Crossref | Google Scholar | PubMed |
Watson JR, Goodrich HR, Cramp RL, Gordos MA, Franklin CE (2019) Assessment of the effects of microPIT tags on the swimming performance of small-bodied and juvenile fish. Fisheries Research 218, 22-28.
| Crossref | Google Scholar |
Wilkes MA, Webb JA, Pompeu PS, Silva LGM, Vowles AS, Baker CF, Franklin P, Link O, Habit E, Kemp PS (2018) Not just a migration problem: metapopulations, habitat shifts, and gene flow are also important for fishway science and management. River Research and Applications 35, 1688-1696.
| Crossref | Google Scholar |