Cold-water pollution impacts on two ‘warm-water’ riverine fish: interactions of dam size and life-history requirements
John D. Koehn


A Department of Energy, Environment and Climate Action, Arthur Rylah Institute for Environmental Research, PO Box 137, Heidelberg, Vic. 3084, Australia.
B Gulbali Institute for Agriculture, Water and Environment, Charles Sturt University, PO Box 789, Albury, NSW 2640, Australia.
Marine and Freshwater Research 74(13) 1154-1170 https://doi.org/10.1071/MF23023
Submitted: 2 February 2023 Accepted: 17 July 2023 Published: 9 August 2023
© 2023 The Author(s) (or their employer(s)). Published by CSIRO Publishing. This is an open access article distributed under the Creative Commons Attribution-NonCommercial-NoDerivatives 4.0 International License (CC BY-NC-ND)
Abstract
Context: Temperature regulates most ecological processes in freshwater ecosystems. Anthropogenic changes to natural thermal regimes, especially cold water released from stratified impoundments (cold-water pollution, CWP), is a widespread and major threat to fish populations globally.
Aims: Because mitigation options for CWP are often expensive, we aimed to provide robust ecological evidence to inform expenditure.
Methods: We modelled population responses to CWP remediation for two warm-water fish species (Murray cod and golden perch) downstream of two impoundments (Copeton and Pindari dams) in the semi-arid northern Murray–Darling Basin, Australia.
Key results: Predicted populations of both species were severely affected by CWP at both sites; however, impacts were species- and site-specific. Effects were greater on golden perch than Murray cod because of their higher temperature requirements for spawning. Predicted spawning opportunities decreased by 77–100% for golden perch and by 38–92% for Murray cod. The larger Copeton Dam had greater impacts than did the smaller Pindari Dam.
Conclusions: Remediation of CWP can help restore populations and meeting the needs of golden perch may benefit a range of other species.
Implications: Globally, CWP is a serious threat to warm-water fishes that may compromise and even nullify other restoration efforts. However, restoration may be impeded by current low abundances, angler harvest and restrictions to fish passage.
Keywords: Australia, golden perch, Murray cod, Murray–Darling Basin, population modelling, river flows, river restoration, semi-arid rivers.
Introduction
Riverine fishes world-wide are subject to numerous threats, many of which are caused by dams and other water infrastructure (Dudgeon et al. 2006; Cooke et al. 2012; Koehn et al. 2020a). Globally, it is estimated that more than 50 000 large dams (>15 m in height) impound naturally flowing river systems for hydropower, irrigation, and domestic water supplies (Lehner et al. 2011). Increased demands for water, exacerbated by human population increases and climate change, and the trend toward reducing CO2 emissions through hydropower means that many more dams are planned for construction (Lehner et al. 2011). Water stored in impoundments is subject to less natural variation in temperature compared with rivers because of its large thermal mass. Releases can either increase or decrease downstream river temperatures depending on dam location, storage capacity, outlet capabilities and operational use, thereby significantly altering the natural temperature regime of downstream rivers (Zarri et al. 2019; Mejia et al. 2020; Nozzi and Stelzer 2021). Releases of water that are warmer than natural negatively affect ‘cold-water’ fishes whereas release of colder water (i.e. CWP) deleteriously affects ‘warm-water’ fishes (Zarri et al. 2019). Either of these changes forces the species affected to attempt to carry out their life history under conditions to which they may not be adapted.
Temperature has been described as a fundamental physical variable (Chandesris et al. 2019), playing an essential role in freshwater ecosystems (Coutant 1977), with aquatic organisms having evolved to specific thermal regimes (Olden and Naiman 2010). This abiotic factor plays a key role in the structure, functioning, abundance and distribution of organisms, including fish (Brett 1971; Beitinger et al. 2000; Preece and Jones 2002). Anthropogenic modifications to natural temperature regimes often cause suboptimal thermal conditions that have been identified as a contributing factor in reductions in native fish populations in freshwater, estuarine and marine ecosystems in many parts of the world (Clarkson and Childs 2000; Roessig et al. 2004). For example, temperature affects metabolism, development (Johnston and Dunn 1987; Clarkson and Childs 2000), reproductive population processes such as gamete maturation (Domagala et al. 2013), embryogenesis, spawning, hatch rates, growth and survival rates (Trip et al. 2014; Fernández-Montero et al. 2018; Stoffels et al. 2020; Raymond et al. 2022).
Optimal thermal ranges and tolerances vary among species and different life-history stages (Coutant 1977; Beitinger et al. 2000; Comte and Olden 2017) but are particularly important for the early life-history stages of fish when developmental changes, hatching, rapid growth and body size are major determinants of survival (Childs and Clarkson 1996; Fuhrman et al. 2018; Michie et al. 2020a; Raymond et al. 2022). For example, altered temperature regimes greatly increased mortality of larval and early juvenile life stages of razorback sucker, Xyrauchen texanus, flannelmouth sucker, Catostomus latipinnis, Humpback Chub, Gila cypha, and Colorado squawfish (pikeminnow), Ptychocheilus lucius (Clarkson and Childs 2000). Hence, changes to water temperatures are often a major stressor to riverine ecosystems and their biota (Dudgeon et al. 2006; Rodgers et al. 2019).
Most research has focused on cold-water species affected by impoundment releases that are warmer than natural (e.g. from power-station cooling ponds; Olden and Naiman 2010; Zarri et al. 2019; Mejia et al. 2020). Less well explored are the negative effects of CWP on downstream ‘warm-water’ fish. Impoundments can stratify the water column, with water below the hypolimnion being up to 17°C colder than at the surface, with low-level releases having impacts up to 300 km downstream (Lugg and Copeland 2014; Michie et al. 2020b). Despite CWP having been documented in many rivers throughout the world, including China (He et al. 2018), North and South America (Agostinho et al. 2008; Miara et al. 2018), Europe (Slavik and Bartos 1997) and Australia (Lugg and Copeland 2014), its extent and impacts on fishes have received little attention globally.
The Murray–Darling Basin (MDB) in south-eastern Australia is one of the world’s most regulated river systems (Grill et al. 2019). It has over 100 large dams, 49 of which have been assessed to cause CWP, affecting over 3000 river-km (Ryan et al. 2001; Preece 2004; Lugg and Copeland 2014). CWP has been studied in the southern MDB on species such as Murray cod (Maccullochella peelii), trout cod (Maccullochella macquariensis) and Macquarie perch (Macquaria australasica) (Koehn et al. 1995; Todd et al. 2005; Sherman et al. 2007; Raymond et al. 2022; Tonkin et al. 2023). It is less well documented for the semi-arid northern MDB where CWP impacts are likely to be more severe owing to warmer water temperatures, more variable temperatures and flows, and for fish species such as golden perch (Macquaria ambigua) with higher temperature requirements (Koehn et al. 2020a).
Individual temperature requirements, together with varying ecological attributes (e.g. movement capabilities), mean that populations of contrasting species may be expected to be affected differentially, especially when impacts interact with the additional ecological and hydrological threats imposed on them (Koehn and Lintermans 2012). For example, highly mobile species with high temperature requirements, such as golden perch, may be more affected over larger scales than are more sedentary species with lower temperature requirements (e.g. Murray cod). In a similar way, not all dams will have the same impacts on water parameters such as flow and temperature, because this will depend on their size, location, outlet structures and modes of operation (Rish et al. 2000; Caissie 2006; Lugg and Copeland 2014). Consequently, individual dams may affect contrasting species in different ways.
CWP imposes yet another major stressor to riverine ecosystems that are already highly affected by a range of threats (Dudgeon et al. 2006; Baumgartner et al. 2020; Koehn et al. 2020b), but of all the threats to fishes, it is one that can continuously directly affects fish (e.g. physiology and metabolism) and may prevent them from completing their life cycles. Without the remediation of CWP, other costly restoration actions such as environmental flows, riparian revegetation, fishway installation or habitat re-instatement (see Koehn et al. 2020b) may be ineffective.
To assess the severity of CWP in the rivers of the northern MDB, we modelled population dynamics of two contrasting, widespread native fish species (Murray cod and golden perch) downstream of two different-sized large dams identified as a high priority for CWP remediation (Preece 2004). Murray cod is an often-sedentary species with reach-scale movements (Koehn et al. 2009), while golden perch is highly mobile at all life stages, responds to flow changes and has higher temperature requirements for spawning (Koehn and Nicol 2016; Zampatti et al. 2018; Koehn et al. 2020a). Although both dams may be categorised as large (Lehner et al. 2011), Pindari Dam is a smaller dam on the Severn River with a multi-level outlet, whereas Copeton Dam is a larger dam on the Gwydir River without one (Fig. 1, Table 1).
Map of the Severn–Macintyre and the Gwydir River study sites in the northern Murray–Darling Basin, Australia. Major storages that potentially release cold-water pollution are indicated by black triangles. Dashed box in the inset shows the scale of the larger map, where the potential extent of CWP is shown in dark blue. The spatial extent of the Murray cod and golden perch populations in this study in the Gwydir River (green line) and Severn River (orange line), with an additional golden perch population in the Mehi River (yellow line). Hydrological gauges analysed in this study are indicated by black diamonds. Note that not all storages and weirs are shown in the figure.
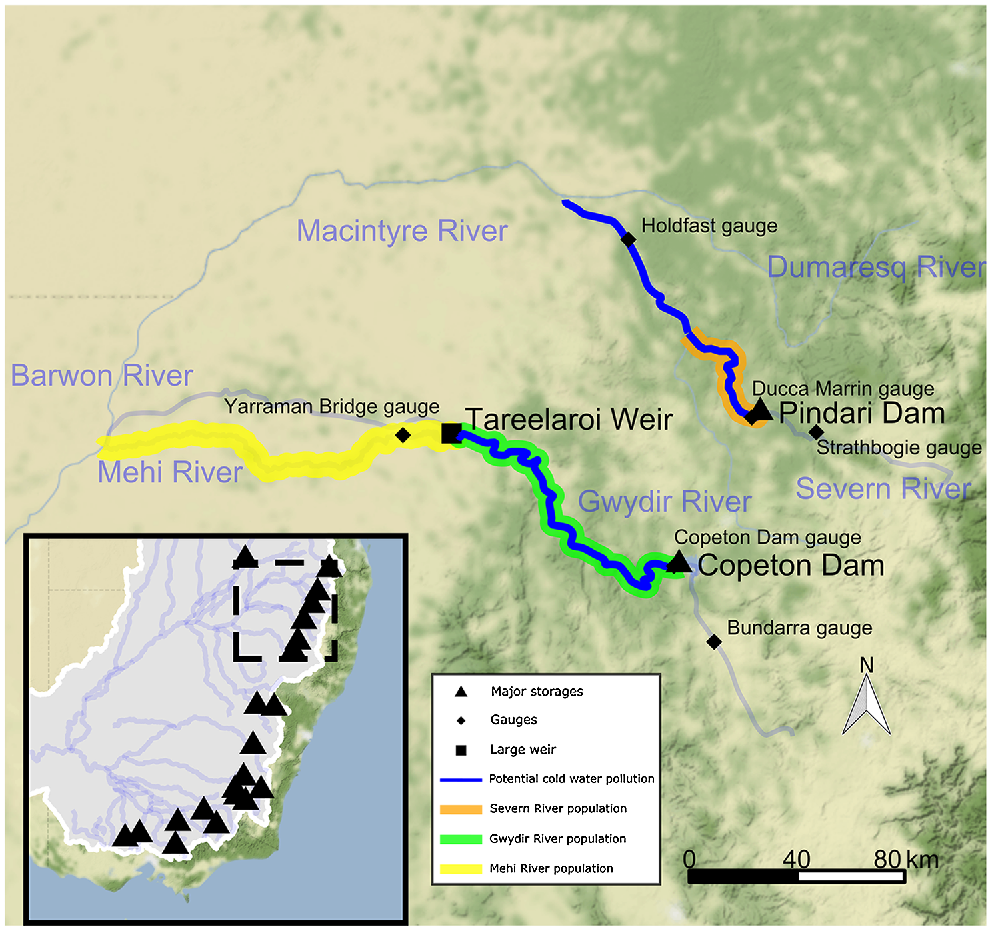
Storage attribute | Pindari Dam | Copeton Dam |
---|---|---|
Storage capacity (GL) | 312 | 1364 |
Maximum depth (m) | 72 | 104 |
High-level outlet structures (m below FSL) | 0–37 (multi-level) | 57–69 |
Deep outletA (m below FSL) | 70 | 84 |
Maximum outlet capacity (ML day−1)B | 5000 | 10 850 |
Thermocline depth range (m below surface) | 5–10 | 5–10 |
Bottom temperature range (°C)C | 11.2–14.1 | 10.1–13.9 |
Surface temperature range (°C)C | 13.5–27.5 | 12.6–26.9 |
River affected | Severn | Gwydir |
Estimated maximum persistence of CWP (km downstream) | >100D | 300D |
The most up-to-date knowledge of these species’ ecology (Koehn et al. 2020a) was incorporated into population models used in this study (Todd et al. 2022). The potential impacts of several thermal scenarios were explored using a population modelling approach to test the following hypotheses:
Decreased water temperatures recorded downstream of the two dams would be likely to inhibit spawning for both species.
Despite ecological differences, populations of both fish species would decline because of CWP in the river reaches downstream of both dams.
There would be species-specific responses with effects on golden perch being greater than for Murray cod.
Impacts on populations from the larger Copeton Dam would be greater than those from the smaller Pindari Dam.
Improvements to populations of both species could be achieved by remediation actions.
Methods
The Murray–Darling Basin
Native fish populations of the MDB are in poor condition, with overall populations now estimated to be <10% levels prior to European settlement (1850s), with the need for restoration now unquestioned (Koehn and Lintermans 2012; Koehn et al. 2020b; Murray–Darling Basin Authority 2020). There are 22 dams identified in the MDB that have the potential to cause some degree of impact on water temperatures downstream (inset, Fig. 1), with CWP reported to have major adverse effects on many river reaches (Koehn et al. 1995; Astles et al. 2003; Lugg and Copeland 2014). Most cold-water discharges occurring seasonally between September and March (austral spring to autumn) (usually involving large volume releases to supply irrigation water) and this largely overlaps with important spawning and recruitment periods for many native fish species (Koehn et al. 2020b).
Naturally, stream temperatures vary depending on meteorological conditions, location, topography, discharge, atmospheric conditions, and streambed attributes (Caissie 2006). In addition, the impact of dam releases depends on the attributes of the dam (Table 1), dam water levels, difference between surface and outlet water temperatures (greater disparity over warmer months), outlets in use, discharge volume (maximum during austral spring and summer), the downstream river channel and volume of water within the downstream reach. On occasions, dams may also spill or rapidly default to low-level outlets to prevent spilling, or to prevent the downstream dispersal of cyanobacteria (Rish et al. 2000).
Effects of CWP may be especially pronounced in the naturally warm, highly flow-variable rivers of the northern MDB. We examined the potential impacts of the following two contrasting dams in the northern MDB: Pindari Dam on the Severn River, which does have a multi-level outlet, and Copeton Dam on the Gwydir River, which does not (Fig. 1, Table 1). These two dams are considered representative for many other sites in both this region and semi-arid rivers more generally. Although both dams can affect water temperatures by ~16°C, Copeton Dam has a capacity approximately four times that of Pindari Dam, is 30 m deeper, has twice the outlet capacity and affects approximately three times the downstream river length (Table 1).
Study species
Murray cod and golden perch are widespread, important species that are highly sought after by recreational anglers (Koehn and Todd 2012). As Murray cod has a national conservation listing, it must be managed for both conservation and recreational fishery objectives (Koehn and Todd 2012), whereas golden perch has no listing. The ecology of both species is well known for the northern MDB (Koehn et al. 2020b), providing a solid knowledge base for the population models. Although both species have similar and large geographic distributions (~1 × 106 km2), their life histories and reported spawning seasons and temperatures vary (Table 2, see Koehn et al. 2020b). The parameters used in this study (Table 2) were conservatively set after reviewing the existing literature and accessing recent research and the expert opinion of local researchers (Jason Thiem and Gavin Butler, NSW DPI Fisheries, unpubl. data). Proportional egg and larval survival as a function of temperature for both species were derived from Todd et al. (2005) (see Fig. S1 and S5 in the Supplementary material).
Species | Murray cod | Golden perch |
---|---|---|
Maximum age (years) | 48 | 26 |
Maximum size (mm) | 1800 (commonly to 1200) | 760 (commonly to 400) |
Ecological description | Apex predator | Predator |
Female age at maturity (years) | 5 | 4 |
Fecundity (approximate number of eggs) | 100 000 | 500 000 |
Spawning type | Pairs, nesting | Communal, pelagic |
Spawning season (NMDB) | October–December | August–February |
Spawning temperature (°C) | 18 | 20 |
Egg type | Adhesive | Pelagic |
Parental care | Eggs and early larvae by male | None |
Egg size (mm) | 6–10 | 3–4 |
Hatch time (days) | 10 | 2 |
Larvae size at hatch (mm) | 10–15 | 3–6 |
Time as drifting larvae (days) | 10 | 14 |
Movement types | Largely sedentary, spawning movement (<120 km) | Highly mobile, all life stages, up to ~1000 km |
Movement scale | River reach | Catchment |
NMDB, northern Murray–Darling Basin.
Murray cod is a demersal river species strongly associated with instream woody habitats (Koehn 2009a, 2009b; Koehn and Nicol 2014) with largely within-reach movements (Koehn et al. 2009; Koehn and Nicol 2016). Therefore, the use of a single population model for each river reach is appropriate, while inter-reach movements of all life stages in and out of each reach should balance out. Paired Murray cod adults spawn and fertilise eggs that adhere to hard substrates (e.g. hollow logs, clay banks) in slow-flowing (<0.1 m s−1) areas. Eggs are guarded by the parental male until they hatch (Cadwallader 1979), with larvae surviving on large yolk-sacs for up to a week as they drift and disperse downstream (Koehn and Harrington 2006).
Golden perch is considered a flow-pulse specialist, with many components of its lifecycle driven by river flows (Sharpe 2011; Koehn et al. 2020a; Stuart and Sharpe 2020). Spawning temperature has variously been recorded up to 24°C (see Koehn et al. 2020a); however, in the model, this trigger was set conservatively at 20°C to reflect recent data (Aquatic Ecology Unit 2010; Jason Thiem, Gavin Butler, NSW DPI Fisheries). This highly mobile species has large-scale movements at all life stages, including drifting eggs and larvae (Koehn and Harrington 2005, 2006) and often considerable upstream and downstream movements of highly mobile juveniles and adults (O’Connor et al. 2005; Koehn and Nicol 2016; Zampatti et al. 2018; Koehn et al. 2020a).
Flow and water-temperature data
Daily flow and temperature data were collected from appropriate gauge locations along each river. These data were analysed within spawning seasons to estimate annual spawning rates and changes to estimates of egg and larval survival rates for each species in each population. The effects of hypoxic blackwater events (see Todd et al. 2022) were also estimated for Murray cod, and movement rates of multiple life-history stages were estimated for golden perch using flow-temperature data inputs. These estimates form the inputs for the population models. Daily water discharge and temperature data were obtained from the river gauges closest to each impoundment (upstream and immediately downstream, CWP-affected) and further downstream (not CWP-affected) of dams (Supplementary Table S1). Available flow and temperature data were downloaded from the MDBA (https://www.mdba.gov.au/) and WaterNSW (https://www.waternsw.com.au/) websites for each location. Data missing from gauges used in this study were estimated (with repetition or modelling of records from the same dataset) to ensure completeness for population modelling (see Table S1 in the Supplementary material for further details of data replication and missing value analysis).
Because of limited complete continuous-flow and temperature datasets, we used shorter but complete flow and temperature data sets to create sequences of data to be able to generate ecologically meaningful output. For the Severn River, we generated a 35-year flow and temperature data set by repeating a 7-year sequence five times (Supplementary material). For the Gwydir River, we generated a 45-year flow and temperature data set for Murray cod by repeating a 9-year sequence five times, and a 27-year flow and temperature data set for the Gwydir and Mehi rivers for golden perch by repeating a 9-year sequence three times (see the Flow and temperature data section of the Supplementary material). It is recognised that this may remove any longer-term trends (including climate change over recent decades) and may dampen natural variability over multiple decades
Population models
We reviewed previously collated ecological knowledge for each species (Koehn et al. 2020a), seeking additional opinion through expert workshops to ensure use of the most recent ecological and operational knowledge relevant to the sites. A conceptual model for the effects of CWP on fishes and their life-stages was developed for each site, assessing the likely levels of susceptibility of the different life stages to temperature impacts. The Murray cod population model was a stage–age matrix construct previously developed for the MDB (Todd and Koehn 2007; Koehn and Todd 2012) that was modified to reflect the species’ ecology in the northern MDB. Two separate cod populations were modelled (Fig. 1), including one population in the Severn River from immediately downstream of Pindari Dam to the confluence of the Macintyre River, and one population in the Gwydir River from downstream of Copeton Dam to Tareelaroi Weir.
The golden perch population models were also modified from a model previously developed for the southern MDB (Todd et al. 2022) to reflect their ecology (Table 2; Koehn et al. 2020a). The golden perch model is similar to the Murray cod model, but includes the flow-dependent movement of multiple life-stages (e.g. larvae, fingerlings, juveniles and adults) of this highly mobile species (Koehn and Nicol 2016; Zampatti et al. 2018). Golden perch populations in the Severn and Gwydir rivers were modelled with the same spatial extents as for Murray cod models above, with an additional population in the Mehi River (downstream of Tareelaroi Weir to the confluence of the Barwon River; Fig. 1). Movement (downstream larval drift, juvenile and adult movements) was estimated between the two populations on the Gwydir and Mehi rivers, making this a metapopulation model. This was necessary to model population dynamics (e.g. movement) between the two reaches split by a the instream Tareelaroi Weir that severely restricts upstream movement. Movements are also restricted by several other structures such as the Gundare Bridge Regulator and Combadello Weir. A second population was not modelled in the Severn because a natural weir at the bottom of the Severn River makes upstream movement impossible (although loss of individuals into the Macintyre River from the Gwydir population is modelled, see the Golden perch model construct section of the Supplementary material for more detail). Further details of the population-model development process, data generation, model rules and parameterisation, including specific spawning cues and flow-movement and larval drift relationships, are provided in Fig. S1–S8 of the Supplementary material.
The output from each population-model simulation was 1000 trajectories of the abundance of fish in each age-class over the period of the applied flow and temperature data for each system and scenario modelled (described in the next section). Trajectories of all adult age classes were tallied, resulting in 1000 trajectories of the total adult population size. The minimum population size from each total adult trajectory was recorded with the associated cumulative frequency distribution of minimum population sizes used to represent risk for the adult population (risk curves: Burgman et al. 1993; Todd et al. 2017). The expected value of these risk curves was also calculated to compare outputs.
CWP amelioration scenarios
To assess a range of CWP amelioration options, several temperature scenarios were explored for each species in each location. In total, six scenarios were modelled in each river, including current conditions (observed cold-water pollution as measured immediately downstream of the dams) and decreasing CWP effects by 2, 4, 6, 8 and 10°C. A counterfactual scenario was also included for the Severn River, with ‘natural’ temperature (i.e. not affected by an impoundment) taken from above Pindari Dam (data from Strathbogie gauge) combined with flow data from gauges downstream of Pindari Dam (Table S1).
Data for each cold-water amelioration scenario were generated by adding 2°C increments to daily temperature records at the site immediately downstream from dam structures in each river. As the affected river reaches are relatively short, temperature changes were not included as a gradient across the affected reaches but modelled as a uniform change. This simple CWP amelioration ‘model’ was used because examination of data on dam levels, depths of the hypolimnion and water release rules (for both irrigation water supply and to avoid releases of cyanobacteria) provided no clear pattern to predict the usage of outlets levels. This uncertainty in dam operations necessitated the use of existing datasets from gauges near the two major storages in this study.
Stocking and angler harvest
Fish stocking data for 2009–2020 were obtained from NSW DPI Fisheries for the Gwydir River reach in this study. Stocking of Murray cod in the Gwydir River was generally low (mean = 560, s.d. = 1701 across modelled years), whereas stocking numbers of golden perch were higher (mean = 7653, s.d. = 10 539 for Gwydir River; mean = 1850, s.d. = 4058 for Mehi River across modelled years). No data of stocking in the Severn River were available. To test whether stocking would assist population recovery for this golden perch population, a simulated stocking rate of 25 000 per year was added from time-step 20 (2034) onward. Stocked individuals were added into the population models as fingerlings (without penalty for being bred in a hatchery) at each relevant location. Angling harvest also occurs in all reaches; however, because data were lacking, this impact was set at an estimated conservative 5% per annum for legal-sized (adult) golden perch and 10% for Murray cod (within the legal slot size of 550–750 mm TL) (see Nicol et al. 2005; Forbes et al. 2015).
Model assumptions
For the Severn River, the average adult population size (AAPS) and initial adult population sizes (IAPS) for Murray cod were 10 000 and 10 000 respectively (assuming ~24 adults per 200 m of river length) and 50 000 and 10 000 for golden perch (assuming ~24 adults per 200 m). The models were run with a ‘burn in’ period of 50 time-steps (years) before implementing temperature effects to avoid initial population size effects.
For the Gwydir River, the density of both species was thought to be lower than in the Severn River and so the AAPS and IAPS for Murray cod were set at 10 000 and 10 000 respectively (assuming ~8 adults per 200 m of river length), with 20 000 and 2000 for golden perch in the Gwydir River (assuming ~2 adults per 200 m) and 20 000 and 5000 for golden perch in the Mehi River (assuming ~4 adults per 200 m).
Spawning opportunity analysis
Changes to temperatures are often expressed as degrees Clesius change from natural. However, there is a need to consider the effects that this may have on fish populations ecologically by affecting environmental conditions in relation to critical life stages and seasons. To provide this ecological perspective on the predicted impacts of CWP, flow and water temperature data were collated and then assessed using the spawning rules within the model for each species (i.e. days above the spawning temperature threshold). The maximum number of spawning days possible in each year under the spawning mechanism is 212 for golden perch and 92 for Murray cod (i.e. the length of the spawning season), where spawning is triggered by flow and temperature attributes for golden perch and temperature alone for Murray cod (see Fig. S1–S3 of the Supplementary material for further details). The number of days in each spawning season from all available years of data at each gauge was calculated for both Murray cod and golden perch at three locations on each river. Locations included immediately downstream of the dam, as well as an upstream and downstream location (details in Table S1). These analyses were undertaken separately to the population modelling and were designed to illustrate the likely impacts of CWP on critical spawning opportunities (and an important component within the population model), without the overlaid complexity of responses within the population models.
Results
Spawning opportunity analysis
Examination of the daily discharge and temperature data indicated that there were considerable changes to both flows and water-temperature regimes in the rivers downstream of both dams (Fig. 2). High flows are initially reduced as water is stored, and then increase as water is released to satisfy irrigation demands. As these high-volume releases are made from low-level outlets, river water temperatures decline markedly (e.g. maximum temperatures declined from 20 to 14°C).
Plots of the daily flow (ML day−1) and temperatures (°C) for (a) Severn River, and (b) Gwydir River for each study site gauge. Daily flow is shown with black lines across upper panels, and daily temperature is represented by red lines (left y-axis) in lower panels for each river. The numbers of spawning days for golden perch are included as annual horizontal black bars (right y-axis) for each site across the lower panels for each river. Note that the length of these bars spans each year and has no bearing on temporal magnitude.
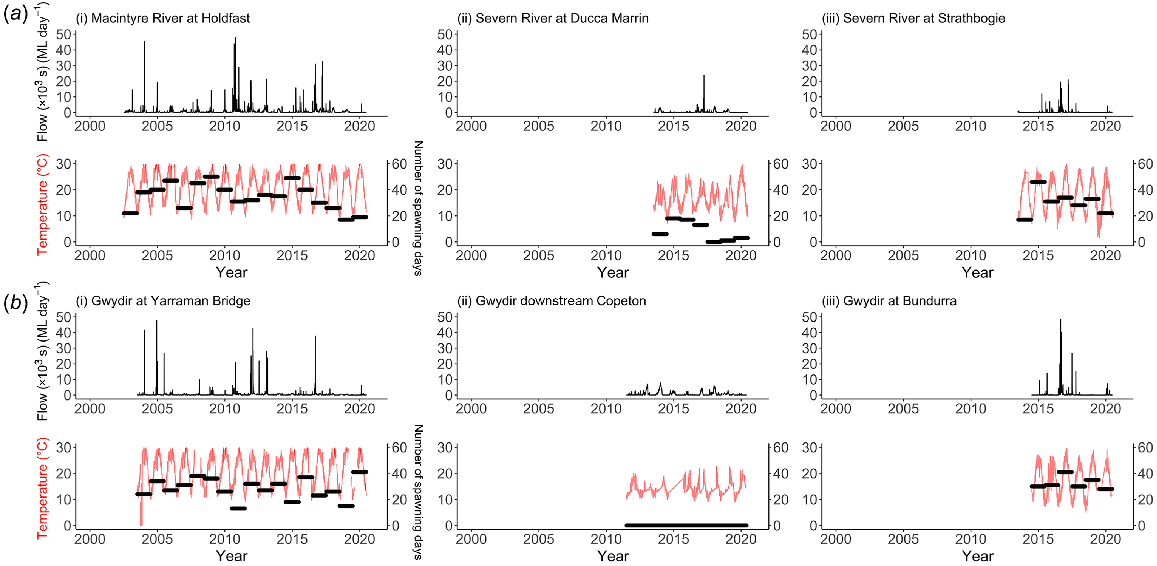
Analysis of these data indicated a significant decrease in average spawning opportunities in CWP-affected reaches (Fig. 2, right-hand y-axis) compared with unaffected reaches (Table 3), up to 100% for golden perch in the reach immediately downstream from Copeton Dam where no spawning was estimated to occur across the analysed years of data. Predicted impacts of CWP on Murray cod spawning were lower than those on golden perch in the Severn River (reduced by 38% below Pindari Dam), but similar in the Gwydir River (reduced by 92% below Copeton Dam). These differences were due to the lower temperature threshold required to initiate spawning for Murray cod than for golden perch (i.e. spawning is modelled as independent of flow, see Methods). As expected, average spawning opportunities were also slightly reduced at the sites upstream of dams, owing to their higher elevation and, hence, naturally cooler water temperatures and more natural flow regimes.
River and gauge site | Murray cod | Golden perch | River and gauge site | Murray cod |
---|---|---|---|---|
Mean (+s.d.) number of spawning days | Percentage reduction in spawning days | Mean (+s.d.) number of spawning days | Percentage reduction in spawning days | |
Severn River | ||||
Macintyre River at Holdfast (downstream of Pindari Dam) | 91 (3) | 35 (10) | ||
Severn River; Ducca Marrin | 56 (19) | 38 | 8 (8) | 77 |
Severn River; Strathbogie (upstream of of Pindari Dam) | 82 (12) | 30 (9) | ||
Gwydir River | ||||
Yarraman Bridge (downstream of Copeton Dam) | 87 (13) | 28 (8) | ||
At Copeton Dam | 7 (11) | 92 | 0 (0) | 100 |
Bundarra (upstream of Copeton Dam) | 76 (7) | 32 (5) |
Spawning days were calculated within the model construct for each species in each year of available data and then summarised and rounded to the nearest integer. See Fig. 1.
Population modelling results
Murray cod was largely unaffected by CWP in the Severn River downstream of Pindari Dam. The population remained relatively stable over time, with little distinction among the different scenarios tested (Fig. 3, Table 4). Remediating CWP had little effect on Murray cod, with only the counter-factual scenario completely removing any cold-water effects (Fig. 3a, b), with negligible percentage differences from the baseline CWP scenario (Table 4). Spawning occurred every year as indicated by spawning analysis (Fig. 2, Table 3). Every 7th year, because of the constructed nature of the flow-temperature timeseries, Murray cod larvae were strongly affected by CWP, although there was recruitment to 1-year olds every year. Including 10% angler harvest further decreased population outcomes with no additional CWP effects (Fig. 3c, d).
Murray cod average adult population size for the Severn River with (a) cold-water pollution (CWP) effects and remediation scenarios; (b) associated risk curves; (c) CSP remediation scenarios with fish harvest; and (d) associated risk curves. CWP-affected temperature scenario increases (a, b) are indicated by coloured lines in the key (+°C). Fishing (c, d) includes angler harvest.
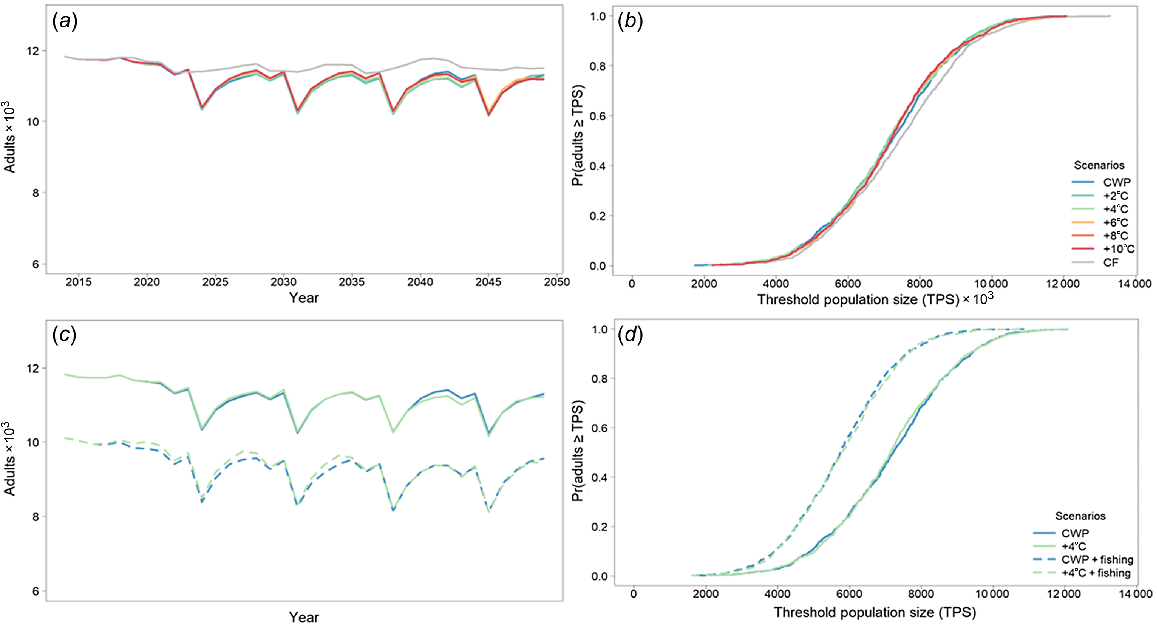
Item | CWP | CWP + 2°C | CWP + 4°C | CWP + 6°C | CWP + 8°C | CWP + 10°C | CF |
---|---|---|---|---|---|---|---|
Severn River | |||||||
Murray cod | 7175 | 7127 | 7150 | 7192 | 7168 | 71 668 | 7413 |
Murray codA | 5757 | 5808 | |||||
Golden perch | 0.58 | 122 | 300 | 256 | 271 | 267 | 3.08 |
Golden perchB | 22 | 569 | |||||
Gwydir River | |||||||
Murray cod | 826 | 1717 | 3781 | 5785 | 6484 | 6490 | n.a. |
Golden perchC | 0.8 | 0.8 | 1.5 | 8.2 | 129.1 | 130.0 | n.a. |
Golden perchD | 12.1 | 12.2 | 20.5 | 71.3 | 333.2 | 336.7 | n.a. |
Golden perchE | 150 | 147 | 160 | 229 | 1078 | 1089 | n.a. |
Golden perchF | 4020 | 3965 | 3978 | 4117 | 4770 | 4804 | n.a. |
The expected minimum population size is the expected value of the distribution of the minimum population size collected from each scenario trajectory, i.e. the expected value of the risk curve.
CF, counter-factual scenario, with ‘natural’ temperature taken from above the impoundment.
AWith fishing.
BWith stocking.
CGwydir with barriers.
DGwydir without barriers.
EMehi with barriers.
FMehi without barriers.
Golden perch was significantly affected by CWP in the Severn River downstream of Pindari Dam. The modelled population declined to near-extinction for the CWP scenario, but was predicted to improve when CWP was remediated (Fig. 4, Table 4). Remediating CWP improves the outcomes for golden perch, with a 4°C increase producing the best results. However, similar improvements were predicted for 6, 8 and 10°C increases, with the percentage differences from the baseline CWP scenario being substantially increased for a 4°C increase and higher (Table 4). Recruitment to 1-year-olds largely occurs every 5 years (Fig. 4a, b), again because of constructed nature of the flow-temperature timeseries, with the greatest recruitment events occurring with 4, 6, 8 and 10°C increases. Even with the improved outcomes from at least a 4°C increase, there is still a high likelihood that the population will be small (Fig. 4). However, stocking may mask the effects of CWP and, in conjunction with remediation, may stabilise a golden perch population (Fig. 4c, d).
Golden perch average adult population size for the Severn River with (a) cold-water pollution (CWP) effects and remediation scenarios; (b) associated risk curves; (c) CWP effects and remediation scenarios with annual stocking of 25 000 fingerlings beginning in 2034; and (d) associated risk curves. CWP-affected temperature scenario increases (a, b) are indicated by coloured lines in the key (+°C). Fishing (c, d) includes angler harvest.
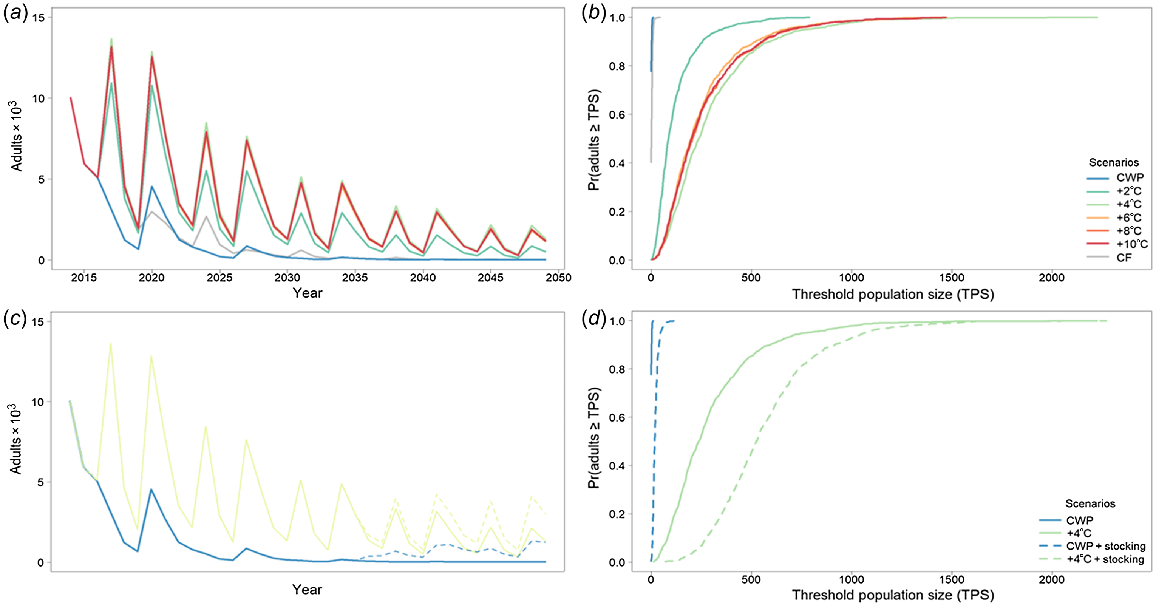
Murray cod was significantly affected by CWP in the Gwydir River downstream of Copeton Dam, with severe population declines and generally low population sizes (e.g. CWP scenario had ~50% smaller population size than did CWP + 2°C) (Fig. 5a, b, Table 4) without any CWP remediation. Remediating CWP in the Gwydir River improved the modelled outcomes for Murray cod, with 8 and 10°C increases producing the best results (i.e. ~680% increase in population size above CWP scenario), although gains are also made with a 6°C increase (Fig. 5a, b, Table 4).
Murray cod average adult population size for the Gwydir River with (a) cold-water pollution (CWP) effects and remediation scenarios; (b) associated risk curves; (c) CWP effects and remediation scenarios on 1-year-olds; and (d) associated risk curves. CWP-affected temperature scenario increases (a, b) are indicated by coloured lines in the key (+°C). Fishing (c, d) includes angler harvest.
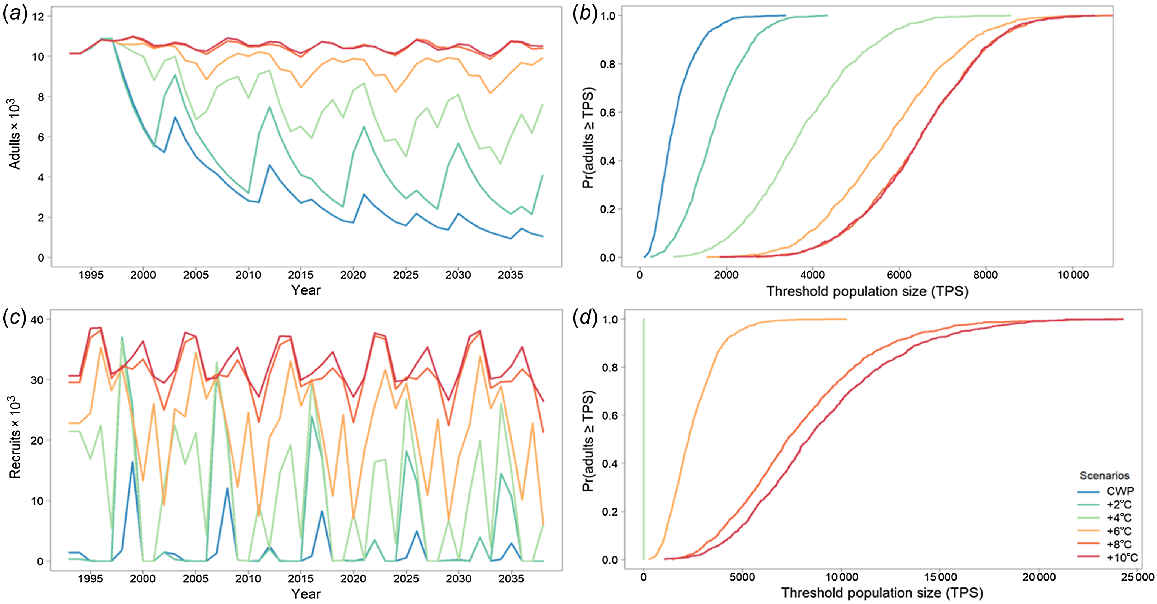
When modelling golden perch as a metapopulation with no barriers to movement, there were two levels of responses to CWP, based on whether there were barriers to movement or not and which population was being assessed (Fig. 6, 7). There were significant effects of CWP with and without barriers in the Gwydir River (Fig. 6), whereas the effects of CWP were marginally different when barriers were removed in the Mehi River (Fig. 7, Table 4). Remediating CWP in the Gwydir River improves the outcomes for golden perch, with 8 and 10°C increases producing the best results. There is an important change in population response with the removal of barriers; however, CWP remains a significant threat for smaller temperature improvements (2–6°C) and it is only the higher levels (8°C+) of CWP remediation (8°C+) that further population increases and reduced risks occur (Fig. 6, Table 4).
Golden perch average adult population size for the Gwydir River with (a) cold-water pollution (CWP) effects and remediation scenarios; (b) associated risk curves; (c) CWP effects and remediation scenarios with barriers removed; and (d) associated risk curves. CWP-affected temperature scenario increases (a, b) are indicated by coloured lines in the key (+°C). Fishing (c, d) includes angler harvest.
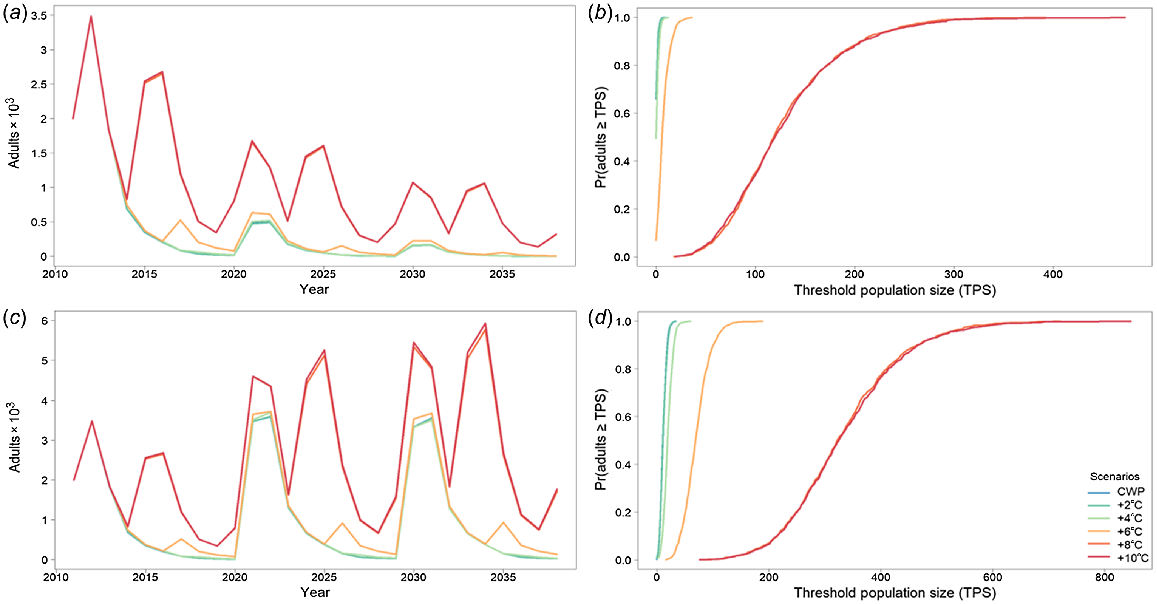
Golden perch average adult population size for the Mehi River with (a) cold-water pollution (CWP) effects and remediation scenarios; (b) associated risk curves; (c) CWP effects and remediation scenarios with barriers removed; and (d) associated risk curves. CWP-affected temperature scenario increases (a, b) are indicated by coloured lines in the key (+°C). Fishing (c, d) includes angler harvest.
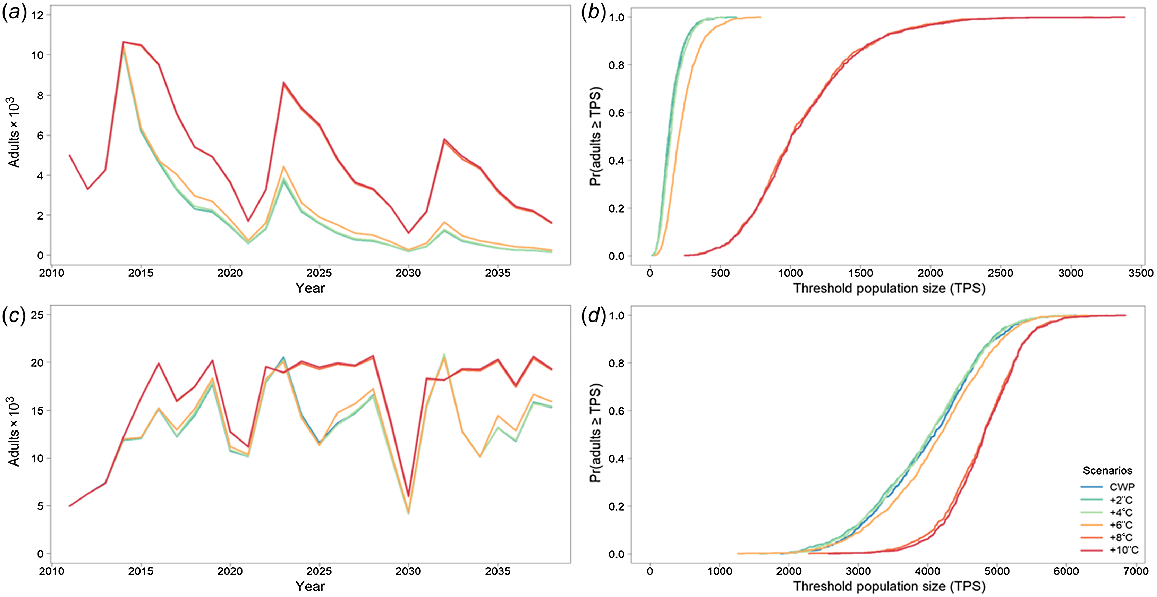
Golden perch in the Mehi River is not directly affected by CWP. However, because of larval drift and other movement rules, the Mehi golden perch population is dependent on the Gwydir population. This dependency is captured in the modelling results where, when modelled with barriers, there is a significant effect on golden perch because of CWP, even though the Mehi River is not affected by CWP (Fig. 7a, b, Table 4). Remediation of CWP, with existing barriers in place occurs only with an increase of 8°C or more. However, with barriers removed, the CWP effect on golden perch populations in the Mehi River is substantially reduced, with much smaller temperature improvement CWP scenarios (Fig. 7c, d, Table 4), with much greater predicted population improvements and significantly reduced risks to the population.
Angler harvest and stocking also had significant effects on population-model outcomes of Murray cod and golden perch. Here, we have highlight impacts of angling on Murray cod and stocking on golden perch on the Severn River. When considered alone, angling significantly reduced the predicted adult Murray cod minimum population size by ~20% in the Severn River (Fig. 3c, d, Table 4). Importantly, the effects of angling also almost completely nullified the positive effects of 4°C CWP amelioration (Fig. 3c, d, Table 4). Conversely, stocking of golden perch into the Severn River supplemented the predicted adult population size above the CWP scenario and when 4°C CWP amelioration (e.g. ~90% increase in the minimum population size) was applied (Fig. 4c, d, Table 4).
Discussion
Modelling in this study indicated that CWP is likely to have considerable negative effects on the riverine populations of Murray cod and golden perch populations in the river reaches downstream of Copeton Dam, and for golden perch population downstream of Pindari Dam. The results supported our hypotheses posed at the beginning of this study. Ecologically, the changes to water temperatures recorded were found to significantly reduce opportunities for spawning for both species (Hypothesis 1). Modelled populations of both species reacted similarly in each river reach (Hypothesis 2), although there were species-specific responses, with predicted impacts being greater for golden perch than for Murray cod, presumably because of their higher temperature requirements (Hypothesis 3). Predicted impacts were greater from the larger Copeton Dam than from Pindari Dam, especially for Murray cod (Hypothesis 4). CWP is expected to cause major impacts on the populations of these two important ‘warm-water’ native fishes, and modelling showed that improvements to populations of both species could be achieved by remediation actions (Hypothesis 5). This highlights that investment to ameliorate this ecological water-quality issue (i.e. to bring temperatures closer to natural) could significantly reduce risk to Murray cod and golden perch, and potentially other native fishes, and improve their population outcomes.
Additionally, our modelling highlighted the impacts CWP may have on populations well beyond the directly affected reach, especially when associated with other threats. When we modelled golden perch as a metapopulation with barriers (weirs) in place, CWP not only affected the population in the Gwydir River immediately downstream of Copeton Dam, but also affected the population in the downstream Mehi River. Rules within the metapopulation structure reflect how barriers can restrict the movement of golden perch and, consequently, exacerbate the effects of CWP by preventing any recolonisation. Tareelaroi Weir is a major barrier to upstream movement, impeding movement of juveniles and adults from the Mehi River to the Gwydir River. Concurrently, the level of egg and larval drift from the Gwydir River is affected by CWP and, hence, the Mehi River does not get the benefit of this input to its golden perch population. Our modelling showed that there is a need to consider other threats such as barriers to fish passage to maximise any benefits from ameliorating CWP.
Interactions of species ecological requirements, dam size and CWP impacts
Golden perch differs from Murray cod in having a higher temperature requirement for spawning and greater mobility throughout its life stages (and, hence, a need for extended riverine connectivity). Analysis and interpretation of the likely ecological impacts of the changes to water temperatures proved useful to highlight the effects on fish population processes. There were significant reductions of the days available with appropriate conditions for spawning within the defined seasons for each species. While spawning is not the only important factor included in the population modelling, it is an important factor, and reductions in spawning opportunities do negatively affect populations. Although the effects of CWP had not previously been explored for golden perch, it was anticipated that the effects would be greater for golden perch than for Murray cod. Indeed, temperature requirements for spawning and recruitment of golden perch (Koehn et al. 2020a) are higher than for many other, but not all, species present at these sites and hence CWP remediation would also meet the needs of other species with similar or lower temperature needs, such as silver perch, Bidyanus bidyanus (Tonkin et al. 2019), or Murray cod. The 20°C threshold used for golden perch spawning was considered somewhat conservative and the effects would be expected to be greater if this key temperature were higher, as has been previously suggested (20–24°C, see Koehn et al. 2020a). Higher temperatures may also be needed for other species such as freshwater catfish, Tandanus tandanus (>20 or 24°C, see Koehn et al. 2020a), and it is best that ecologically significant temperatures for such species be used as targets for remediation.
The CWP-modelled impacts from Pindari Dam on golden perch in the Severn River showed that the population will decline, with a very high likelihood of the population being small if no remediation is undertaken. Our modelled results indicated that population improvements are somewhat attenuated after an increase of ~4°C. The attenuation possibly occurs because the temperature increases above 4°C may modify the start of the spawning season, from which no additional benefits occur because of being confounded with the constructed nature of the flow-temperature timeseries. It is unlikely that this would occur under ‘real world’ circumstances, and although our modelling did not indicate it, it is likely that there would be further improvements beyond a 4°C increase in water temperature. Although it is possible that this temperature sequence may have underestimated the CWP effects on Murray cod, the overall outcome is clear in that CWP poses a major threat to golden perch populations, and a lesser one to Murray cod in the Severn River. CWP also has a major impact on the population of Murray cod in the Gwydir River downstream of Copeton Dam. Reinstatement of a natural temperature regime would dramatically increase the adult Murray cod population size (84% over 45 years) and decrease risks of population reductions. This modelled response to CWP remediation effects is much greater than those for the Severn River.
The difference in modelled CWP impacts between the two dams in this study is at least in part due to their size, with greater effects from the larger Copeton Dam than from the smaller Pindari Dam. A priority may be to remediate the larger dams to give the greatest environmental benefits. The impact of CWP is not only a result of the dam size and location, but also of factors such as dam fullness, the difference between surface and bottom water temperatures (typically greatest in warmer seasons), the low-level outlets that are in use, volume of discharge and other operational rules such as not releasing cyanobacteria or supplying drinking water (Rish et al. 2000; Weber et al. 2017). The impacts form Pindari Dam may also have been ameliorated by its multi-level outlet, which would provide warmer water when operated correctly. However, full use of this outlet is often limited owing to cyanobacterial blooms making its efficacy hard to determine. Such complexities result in site-specific effects and indicate the necessity to assess CWP impacts on a case-by-case and species-specific basis.
Fish passage, angler harvest and stocking
There are many other impacts on native fishes that may either compound the effects of CWP or prevent population recovery through remediation measures that need to be considered (Koehn and Lintermans 2012). In particular, these include the need for fish passage (Baumgartner et al. 2014), adequate river flows and stocking and harvesting for recreational angling species (such as Murray cod and golden perch). Our modelling in the Gwydir–Mehi River system highlighted the importance of fish passage for a highly mobile species such as golden perch, indicating the need for considering CWP in conjunction with barriers to movements. Our exploration of angler harvest within the slot legal limit at the modest rate of 10% also illustrated the potential additional impact that additional fish removal may have on population recovery. Here, simulated harvest almost completely negated the positive effects of 4°C of CWP amelioration, likely as a function of fewer adults, and hence reduced reproductive potential of the population. Overall, this suggests that angling is likely to dampen the positive influence of CWP amelioration efforts. For example, when the angler harvest is included, there is a further reduction in populations. CWP has a much greater impact on golden perch where recruitment to 1-year olds shows episodic recruitment, with only two recruitment events in the 7-year repeated cycle modelled. Conversely, stocking may mask the effects of CWP through population stabilisation, but will not necessarily restore population function. However, stocking did improve ‘recruitment’ for some populations, which would be unlikely to remain extant without continued stocking.
Remediation options
Remediating CWP is possible through a range of engineering and operational options (Sherman 2000; Michie et al. 2020c). For example, multi-level outlets have been successful in mitigating thermal pollution (Du Xiaohu 2009; Wu et al. 2011). Whereas retrofitting older dams may be costly (Sherman 2000), there are other infrastructure options such as trunnions, destratification mixers, bubble mixers and thermal curtains that divert warmer surface waters to low-level outlets (He et al. 2017; Gray et al. 2019; Michie et al. 2020b). The utility and effectiveness of these options will be site-specific, and improvements to water temperatures and their potential benefits require quantification.
Although our results indicated that remediation of CWP could help restore populations of Murray cod and golden perch, even at dams with selective withdrawal infrastructure, full remediation of CWP may still not occur because of structural or operational limitations (e.g. Acaba et al. 2000; Rish et al. 2000). The delivery of irrigation flow water is largely dependent on the seasonal demands of farmers, which unfortunately overlaps considerably with the spawning and critical early life-stage seasons of many MDB warm-water fishes. Avoiding the delivery of cold water in these key seasons would be helpful as an interim measure. In the absence of this option, remediating CWP largely relies on investment in engineering and operational options (Sherman 2000; Boys et al. 2009; Michie et al. 2020c). It is especially important that CWP mitigation options are included in the design and construction of new dams or the expansion of existing dams. There may also be some operational alterations, such as water delivery times, that could assist in reducing the downstream impacts, particularly in years when delivery of irrigation water is limited (Tonkin et al. 2023). Additional to any planned remediation or construction of new dams, the design must predict daily temperatures and these temperatures need to be modelled to ascertain their appropriateness for the species affected.
The existing low abundances of MDB fish populations estimated to be <10% of pre-European levels (Murray–Darling Basin Ministerial Council 2004; Koehn and Lintermans 2012; Murray–Darling Basin Authority 2020) may also be an impediment to any rapid improvement in fish populations. Data from recent fish surveys in the northern MDB (from the Sustainable Rivers Audit and Murray–Darling Fish Surveys; Greg Ringwood, MDBA, unpubl. data) indicated low existing population abundances for both species, which would mean long recovery times, even with threat remediation. Additional measures such as stocking or additional harvest restrictions may be needed to facilitate recovery. Hence, population restoration is more complex than just remediating any one threat, and requires an holistic approach.
Implications for water management
Given the widespread nature and serious impacts of CWP, it also potentially compromises the recovery of fish populations through other rehabilitation programs such as the use of environmental water (Arthington 2012), which includes the Basin Plan, costing ~A$13 billion (Murray–Darling Basin Authority 2011). Most attention in such water delivery is placed on the quantity of water available, rather than its quality. Without providing the temperature thresholds needed for the adequate completion of fish life-cycles and recruitment into adult populations, the objective of this and other environmental programs (e.g. habitat re-instatement) may not be achieved and expenditure may be wasted. This places the remediation of CWP as a priority action in many rivers. The requirements of golden perch have been used as an example for species in this study, but by using ecologically significant threshold-temperature requirements relating to the needs of other aquatic species will provide the best outcomes from remediation.
Benefits, constraints and future advances of population modelling
Population modelling provides an important method by which to evaluate the likely impacts of threats such as CWP. It also provides a mechanism to assist managers to prioritise rehabilitation options to improve native fish populations and to ensure the best value for expenditure (Koehn and Todd 2012). Modelling of CWP has been piecemeal, and a strategic and consolidated approach across other sites and species would help further quantify this very widespread threat. Ecological knowledge forms a strong basis for such modelling, and this knowledge is now generally very good for Murray cod and golden perch, the subject species for this study (Koehn et al. 2019, 2020a). However, it is recognised that this may not be so for other locations and species.
Modelling in this project was hampered by the availability of complete long-term flow and water temperature datasets, and this highlights the need for more comprehensive, consistent and accurate water-temperature monitoring with the ready availability of such data. Temperature underpins many physiological and ecological functions of fish (Johnston and Dunn 1987; Whiterod et al. 2018) that are not considered within this study. For example, CWP affects swimming capabilities (Lyon et al. 2008; Whiterod 2013) and may pose a barrier to fish movement, which has wider implications for predator avoidance and the movements, recolonisation and population dynamics of mobile species such as golden perch (Koehn et al. 2020a). Temperature is also an important regulator of riverine processes such as productivity and the life history of invertebrates, an important food source for fish.
Population modelling is needed for other affected river reaches and a range of other species and life-stages, especially threatened species such as silver perch and the many small-bodied fishes that are often forgotten (Todd et al. 2017). Early life-stages are often the most vulnerable (Raymond et al. 2022), but often receive the least research attention (Koehn et al. 2019). There is also a need to identify biological, ecological and limnological knowledge gaps and undertake relevant research (e.g. minimum site-specific ecological temperature tolerances) to inform operational targets and protocols, and the development of monitoring and evaluation plans (Mejia et al. 2020).
Conclusions
This study has predicted major detrimental impacts on the populations of the two warm-water species, Murray cod and golden perch, from CWP in Australia’s northern MDB. It is likely that these CWP effects are greater in the northern MDB than those previously reported for the cooler, southern MDB. The fact that CWP affects over 3000 km of rivers in Australia’s MDB (Lugg and Copeland 2014) exemplifies the possible, but under-appreciated, magnitude of such impacts elsewhere in the world. Results from this study have application to river reaches and warm-water fishes downstream of dams globally. The impacts on golden perch exceeded those on Murray cod because of their requirement for higher temperatures for spawning. Meeting the water-temperature needs of golden perch is likely to accommodate the needs of many other species that have lower temperature requirements. Impacts from the larger Copeton Dam were greater than from the smaller Pindari Dam and perhaps these larger dams should be prioritised for remediation to achieve the best environmental outcomes. Results showed how various levels of mitigation of CWP could help restore populations, although restoration may be difficult and long term, owing to current low abundances of these species and the effects of other threats. In particular, population restoration was shown to be severely inhibited or even prevented when barriers to fish passage were in place for the highly mobile golden perch. Restoration could also be reduced by angler harvest, and stocking may be masking the full effects of CWP in some reaches. The mitigation of CWP on existing structures may not only restore ~3000 km of river reaches in the MDB, but also remove an impediment that is likely to thwart the full benefits from other restoration measures such as the use of environmental water. Mechanisms to prevent CWP should also be incorporated into all new structures.
Acknowledgements
The authors thank Charlie Caruthers, Heleena Bamford and Tony Townsend from NSW DPI Fisheries for their support on this project. Thanks go to Ivor Stuart and Andrew Boulton for helpful discussions during this project and paper, along with Matt Gordos (NSW Fisheries) and Andrew Boulton for their very helpful comments on manuscript drafts. The production of this paper was supported by the Applied Aquatic Ecology writing retreat through the Arthur Rylah Institute Capability Fund. Many other colleagues have contributed to the development of the population models for these two study species.
References
Agostinho AA, Pelicice FM, Gomes LC (2008) Dams and the fish fauna of the Neotropical region: impacts and management related to diversity and fisheries. Brazilian Journal of Biology 68, 1119-1132.
| Crossref | Google Scholar |
Baumgartner LJ, Reynoldson NK, Cameron L, Stanger JG (2009) Effects of irrigation pumps on riverine fish. Fisheries Management and Ecology 16, 429-437.
| Crossref | Google Scholar |
Baumgartner L, Zampatti B, Jones M, Stuart I, Mallen-Cooper M (2014) Fish passage in the Murray–Darling Basin, Australia: not just an upstream battle. Ecological Management & Restoration 15, 28-39.
| Crossref | Google Scholar |
Baumgartner LJ, Gell P, Thiem JD, Finlayson CM, Ning N (2020) Ten complementary measures to assist with environmental watering programs in the Murray–Darling river system, Australia. River Research and Applications 36, 645-655.
| Crossref | Google Scholar |
Beitinger TL, Bennett WA, McCauley RW (2000) Temperature tolerances of north American freshwater fishes exposed to dynamic changes in temperature. Environmental Biology of Fishes 58, 237-275.
| Crossref | Google Scholar |
Brett JR (1971) Energetic responses of salmon to temperature. A study of some thermal relations in the physiology and freshwater ecology of sockeye salmon (Oncorhynchus nerkd). American Zoologist 11, 99-113.
| Crossref | Google Scholar |
Cadwallader PL (1979) Distribution of native and introduced fish in the Seven Creeks River system, Victoria. Australian Journal of Ecology 4, 361-385.
| Crossref | Google Scholar |
Caissie D (2006) The thermal regime of rivers: a review. Freshwater Biology 51, 1389-1406.
| Crossref | Google Scholar |
Chandesris A, Van Looy K, Diamond JS, Souchon Y (2019) Small dams alter thermal regimes of downstream water. Hydrology and Earth System Sciences 23, 4509-4525.
| Crossref | Google Scholar |
Childs MR, Clarkson RW (1996) Temperature effects on swimming performance of larval and juvenile Colorado squawfish: implications for survival and species recovery. Transactions of the American Fisheries Society 125, 940-947.
| Crossref | Google Scholar |
Clarkson RW, Childs MR (2000) Temperature effects of hypolimnial-release dams on early life stages of Colorado River Basin big-river fishes. Copeia 2000(2), 402-412.
| Google Scholar |
Comte L, Olden JD (2017) Evolutionary and environmental determinants of freshwater fish thermal tolerance and plasticity. Global Change Biology 23, 728-736.
| Crossref | Google Scholar |
Cooke SJ, Paukert C, Hogan Z (2012) Endangered river fish: factors hindering conservation and restoration. Endangered Species Research 17, 179-191.
| Crossref | Google Scholar |
Coutant CC (1977) Compilation of temperature preference data. Journal of the Fisheries Board of Canada 34, 739-745.
| Crossref | Google Scholar |
Domagala J, Kirczuk L, Pilecka-Rapacz M (2013) Annual development cycle of gonads of Eurasian ruffe (Gymnocephalus cernuus L.) females from lower Odra River sections differing in the influence of cooling water. Journal of Freshwater Ecology 28, 423-437.
| Crossref | Google Scholar |
Du Xiaohu LY (2009) Analysis of design and operation of selective withdrawal modifications of Hungry Horse Dam. Water Power 4, 007.
| Google Scholar |
Dudgeon D, Arthington AH, Gessner MO, Kawabata Z-I, Knowler DJ, Lévêque C, Naiman RJ, Prieur-Richard A-H, Soto D, Stiassny MLJ, Sullivan CA (2006) Freshwater biodiversity: importance, threats, status and conservation challenges. Biological Reviews of the Cambridge Philosophical Society 81, 163-182.
| Crossref | Google Scholar |
Fernández-Montero A, Caballero MJ, Torrecillas S, Tuset VM, Lombarte A, Ginés RR, Izquierdo M, Robaina L, Montero D (2018) Effect of temperature on growth performance of greater amberjack (Seriola dumerili Risso, 1810) juveniles. Aquaculture Research 49(2), 908-918.
| Crossref | Google Scholar |
Forbes JP, Watts RJ, Robinson WA, Baumgartner LJ, Steffe AS, Murphy JJ (2015) Recreational fishing effort, catch, and harvest for Murray cod and Golden perch in the Murrumbidgee River, Australia. North American Journal of Fisheries Management 35, 649-658.
| Crossref | Google Scholar |
Fuhrman AE, Larsen DA, Steel EA, Young G, Beckman BR (2018) Chinook salmon emergence phenotypes: describing the relationships between temperature, emergence timing and condition factor in a reaction norm framework. Ecology of Freshwater Fish 27, 350-362.
| Crossref | Google Scholar |
Gray R, Jones HA, Hitchcock JN, Hardwick L, Pepper D, Lugg A, Seymour JR, Mitrovic SM (2019) Mitigation of cold-water thermal pollution downstream of a large dam with the use of a novel thermal curtain. River Research and Applications 35, 855-866.
| Crossref | Google Scholar |
Grill G, Lehner B, Thieme M, Geenen B, Tickner D, Antonelli F, Babu S, Borrelli P, Cheng L, Crochetiere H, Ehalt Macedo H, Filgueiras R, Goichot M, Higgins J, Hogan Z, Lip B, McClain ME, Meng J, Mulligan M, Nilsson C, Olden JD, Opperman JJ, Petry P, Reidy Liermann C, Sáenz L, Salinas-Rodríguez S, Schelle P, Schmitt RJP, Snider J, Tan F, Tockner K, Valdujo PH, van Soesbergen A, Zarfl C (2019) Mapping the world’s free-flowing rivers. Nature 569, 215-221.
| Crossref | Google Scholar |
He W, Lian J, Yao Y, Wu M, Ma C (2017) Modeling the effect of temperature-control curtain on the thermal structure in a deep stratified reservoir. Journal of Environmental Management 202, 106-116.
| Crossref | Google Scholar |
He W, Lian J, Du H, Ma C (2018) Source tracking and temperature prediction of discharged water in a deep reservoir based on a 3-D hydro-thermal-tracer model. Journal of Hydro-environment Research 20, 9-21.
| Crossref | Google Scholar |
Johnston IA, Dunn J (1987) Temperature acclimation and metabolism in ectotherms with particular reference to teleost fish. Symposia of the Society for Experimental Biology 41, 67-93.
| Google Scholar |
Koehn JD (2009a) Using radio telemetry to evaluate the depths inhabited by Murray cod (Maccullochella peelii peelii). Marine and Freshwater Research 60, 317-320.
| Crossref | Google Scholar |
Koehn JD (2009b) Multi-scale habitat selection by Murray cod Maccullochella peelii peelii in two lowland rivers. Journal of Fish Biology 75, 113-129.
| Crossref | Google Scholar |
Koehn JD, Harrington DJ (2005) Collection and distribution of the early life stages of the Murray cod (Maccullochella peelii peelii) in a regulated river. Australian Journal of Zoology 53, 137-144.
| Crossref | Google Scholar |
Koehn JD, Harrington DJ (2006) Environmental conditions and timing for the spawning of Murray cod (Maccullochella peelii peelii) and the endangered trout cod (M. macquariensis) in southeastern Australian rivers. River Research and Applications 22, 327-342.
| Crossref | Google Scholar |
Koehn JD, Lintermans M (2012) A strategy to rehabilitate fishes of the Murray–Darling Basin, south-eastern Australia. Endangered Species Research 16, 165-181.
| Crossref | Google Scholar |
Koehn JD, Nicol SJ (2014) Comparative habitat use by large riverine fishes. Marine and Freshwater Research 65, 164-174.
| Crossref | Google Scholar |
Koehn JD, Nicol SJ (2016) Comparative movements of four large fish species in a lowland river. Journal of Fish Biology 88, 1350-1368.
| Crossref | Google Scholar |
Koehn JD, Todd CR (2012) Balancing conservation and recreational fishery objectives for a threatened fish species, the Murray Cod, Maccullochella peelii. Fisheries Management and Ecology 19, 410-425.
| Crossref | Google Scholar |
Koehn JD, McKenzie JA, O’Mahony DJ, Nicol SJ, O’Connor JP, O’Connor WG (2009) Movements of Murray cod (Maccullochella peelii peelii) in a large Australian lowland river. Ecology of Freshwater Fish 18, 594-602.
| Crossref | Google Scholar |
Koehn JD, Balcombe SR, Zampatti BP (2019) Fish and flow management in the Murray–Darling Basin: directions for research. Ecological Management & Restoration 20, 142-150.
| Crossref | Google Scholar |
Koehn JD, Raymond SM, Stuart I, Todd CR, Balcombe SR, Zampatti BP, Bamford H, Ingram BA, Bice CM, Burndred K, Butler G, Baumgartner L, Clunie P, Ellis I, Forbes JP, Hutchison M, Koster WM, Lintermans M, Lyon JP, Mallen-Cooper M, McLellan M, Pearce L, Ryall J, Sharpe C, Stoessel DJ, Thiem JD, Tonkin Z, Townsend A, Ye Q (2020a) A compendium of ecological knowledge for restoration of freshwater fishes in Australia’s Murray–Darling Basin. Marine and Freshwater Research 71, 1391-1463.
| Crossref | Google Scholar |
Koehn JD, Balcombe SR, Baumgartner LJ, Bice CM, Burndred K, Ellis I, Koster WM, Lintermans M, Pearce L, Sharpe C, Stuart I, Todd CR (2020b) What is needed to restore native fishes in Australia’s Murray–Darling Basin? Marine and Freshwater Research 71, 1464-1468.
| Crossref | Google Scholar |
Lehner B, Liermann CR, Revenga C, Vörösmarty C, Fekete B, Crouzet P, Döll P, Endejan M, Frenken K, Magome J, Nilsson C, Robertson JC, Rödel R, Sindorf N, Wisser D (2011) High-resolution mapping of the world’s reservoirs and dams for sustainable river-flow management. Frontiers in Ecology and the Environment 9, 494-502.
| Crossref | Google Scholar |
Lugg A, Copeland C (2014) Review of cold water pollution in the Murray–Darling Basin and the impacts on fish communities. Ecological Management & Restoration 15, 71-79.
| Crossref | Google Scholar |
Lyon JP, Ryan TJ, Scroggie MP (2008) Effects of temperature on the fast-start swimming performance of an Australian freshwater fish. Ecology of Freshwater Fish 17, 184-188.
| Crossref | Google Scholar |
Mejia FH, Torgersen CE, Berntsen EK, Maroney JR, Connor JM, Fullerton AH, Ebersole JL, Lorang MS (2020) Longitudinal, lateral, vertical, and temporal thermal heterogeneity in a large impounded river: implications for cold-water refuges. Remote Sensing 12, 1386.
| Crossref | Google Scholar |
Miara A, Vörösmarty CJ, Macknick JE, Tidwell VC, Fekete B, Corsi F, Newmark R (2018) Thermal pollution impacts on rivers and power supply in the Mississippi River watershed. Environmental Research Letters 13, 034033.
| Crossref | Google Scholar |
Michie LE, Thiem JD, Boys CA, Mitrovic SM (2020a) The effects of cold shock on freshwater fish larvae and early-stage juveniles: implications for river management. Conservation Physiology 8, coaa092.
| Crossref | Google Scholar |
Michie LE, Hitchcock JN, Thiem JD, Boys CA, Mitrovic SM (2020b) The effect of varied dam release mechanisms and storage volume on downstream river thermal regimes. Limnologica 81, 125760.
| Crossref | Google Scholar |
Michie LE, Thiem JD, Facey JA, Boys CA, Crook DA, Mitrovic SM (2020c) Effects of suboptimal temperatures on larval and juvenile development and otolith morphology in three freshwater fishes: implications for cold water pollution in rivers. Environmental Biology of Fishes 103(12), 1527-1540.
| Crossref | Google Scholar |
Murray–Darling Basin Authority (2011) Delivering a healthy working basin. About the draft basin plan. MDBA publication number 65/10. (MDBA: Canberra, ACT, Australia) Available at https://www.mdba.gov.au/sites/default/files/publications/delivering-a-healthy-working-basin.pdf [Verified 16 April 2020]
Murray–Darling Basin Authority (2013) Preliminary overview of constraints to environmental water delivery in the Murray–Darling Basin. Technical Support Document. Publication Number 14/13. (MDBA: Canberra, ACT, Australia) Available at https://www.mdba.gov.au/sites/default/files/publications/mdbaconstraintsoverview-20130703.pdf
Murray–Darling Basin Authority (2020) Native fish recovery strategy. Working together for the future of native fish. (MDBA: Canberra, ACT, Australia) Available at https://www.mdba.gov.au/sites/default/files/publications/native-fish-recovery-strategy-brochure.pdf [Verified 28 July 2023]
Murray–Darling Basin Ministerial Council (2004) Native fish strategy for the Murray–Darling Basin 2003–2013. (MDBMC: Canberra, ACT, Australia) Available at https://www.fish.gov.au/Archived-Reports/2014/Documents/2014_refs/MDBC%202004%20Native%20fish%20strategy%20for%20the%20Murray-Darling%20Basin%202003-2013.pdf
Nicol S, Todd C, Koehn J, Lieschke J (2005) How can recreational angling regulations help meet the multiple objectives for the management of Murray cod populations? In ‘Management of Murray Cod in the Murray–Darling Basin: statement, recommendations and supporting papers’, 3–4 June 2004, Canberra, ACT, Australia. (Eds M Lintermans, B Phillips) MDBC Publication Number 22/05, pp. 98–106. (Murray–Darling Basin Commission and Cooperative Research Centre for Freshwater Ecology: Canberra, ACT, Australia)
Nozzi N, Stelzer RS (2021) Responses of fish communities to longitudinal thermal gradients in coldwater, lake outflow streams. Environmental Biology of Fishes 104, 1235-1249.
| Crossref | Google Scholar |
Olden JD, Naiman RJ (2010) Incorporating thermal regimes into environmental flows assessments: modifying dam operations to restore freshwater ecosystem integrity. Freshwater Biology 55, 86-107.
| Crossref | Google Scholar |
O’Connor JP, O’Mahony DJ, O’Mahony JM (2005) Movements of Macquaria ambigua, in the Murray River, south-eastern Australia. Journal of Fish Biology 66, 392-403.
| Crossref | Google Scholar |
Preece RM, Jones HA (2002) The effect of Keepit Dam on the temperature regime of the Namoi River, Australia. Rivers Research and Applications 18, 397-414.
| Crossref | Google Scholar |
Raymond S, Ryall J, Koehn J, Fanson B, Hill S, Stoessel D, Tonkin Z, Sharley J, Todd C, Campbell A, Lyon J, Turner M, Ingram B (2022) Larval fish sensitivity to a simulated cold-water pulse varies between species and age. Journal of Limnology 81, 2056.
| Crossref | Google Scholar |
Rodgers EM, Todgham AE, Connon RE, Fangue NA (2019) Stressor interactions in freshwater habitats: effects of cold water exposure and food limitation on early-life growth and upper thermal tolerance in white sturgeon, Acipenser transmontanus. Freshwater Biology 64, 348-358.
| Crossref | Google Scholar |
Roessig JM, Woodley CM, Cech JJ, Jr, Hansen LJ (2004) Effects of global climate change on marine and estuarine fishes and fisheries. Reviews in Fish Biology and Fisheries 14, 251-275.
| Crossref | Google Scholar |
Sherman B, Todd CR, Koehn JD, Ryan T (2007) Modelling the impact and potential mitigation of cold water pollution on Murray cod populations downstream of Hume Dam, Australia. Rivers Research and Applications 23, 377-389.
| Crossref | Google Scholar |
Stoffels RJ, Weatherman KE, Bond NR, Morrongiello JR, Thiem JD, Butler G, Koster W, Kopf RK, McCasker N, Ye Q, Zampatti B, Broadhurst B (2020) Stage-dependent effects of river flow and temperature regimes on the growth dynamics of an apex predator. Global Change Biology 26, 6880-6894.
| Crossref | Google Scholar |
Stuart IG, Sharpe CP (2020) Riverine spawning, long distance larval drift, and floodplain recruitment of a pelagophilic fish: a case study of golden perch (Macquaria ambigua) in the arid Darling River, Australia. Aquatic Conservation: Marine and Freshwater Ecosystems 30, 675-690.
| Crossref | Google Scholar |
Todd CR, Ryan T, Nicol SJ, Bearlin AR (2005) The impact of cold water releases on the critical period of post-spawning survival and its implications for Murray cod (Maccullochella peelii peelii): a case study of the Mitta Mitta River, southeastern Australia. River Research and Applications 21, 1035-1052.
| Crossref | Google Scholar |
Todd CR, Koehn JD, Pearce L, Dodd L, Humphries P, Morrongiello JR (2017) Forgotten fishes: what is the future for small threatened freshwater fish? Population risk assessment for southern pygmy perch, Nannoperca australis. Aquatic Conservation: Marine and Freshwater Ecosystems 27, 1290-1300.
| Crossref | Google Scholar |
Todd C, Wootton H, Koehn J, Stuart I, Hale R, Fanson B, Sharpe C, Thiem J (2022) Population modelling of native fish outcomes for the Reconnecting River Country Program: Golden Perch and Murray Cod. Final report for the NSW Department of Planning and Environment, Reconnecting River Country Program. Arthur Rylah Institute for Environmental Research, Technical Report Series Number 341, Department of Environment, Land, Water and Planning, Melbourne, Vic., Australia.
Tonkin Z, Stuart I, Kitchingman A, Thiem JD, Zampatti B, Hackett G, Koster W, Koehn J, Morrongiello J, Mallen-Cooper M, Lyon J (2019) Hydrology and water temperature influence recruitment dynamics of the threatened silver perch Bidyanus bidyanus in a regulated lowland river. Marine and Freshwater Research 70, 1333-1344.
| Crossref | Google Scholar |
Tonkin Z, Koehn J, Commens S, Hackett G, Harris A, Kitchingman A, Lyon J, Moloney P, Todd C, Woodhead J (2023) Using multiple lines of evidence to assess recovery potential of a warm water fish population in a cold water impacted river. Frontiers in Conservation Science 4, 1103256.
| Crossref | Google Scholar |
Trip EDL, Clements KD, Raubenheimer D, Choat JH (2014) Temperature-related variation in growth rate, size, maturation and life span in a marine herbivorous fish over a latitudinal gradient. Journal of Animal Ecology 83, 866-875.
| Crossref | Google Scholar |
Weber M, Rinke K, Hipsey MR, Boehrer B (2017) Optimizing withdrawal from drinking water reservoirs to reduce downstream temperature pollution and reservoir hypoxia. Journal of Environmental Management 197, 96-105.
| Crossref | Google Scholar |
Whiterod NS (2013) The swimming capacity of juvenile Murray cod (Maccullochella peelii): an ambush predator endemic to the Murray–Darling Basin, Australia. Ecology of Freshwater Fish 22, 117-126.
| Crossref | Google Scholar |
Whiterod NS, Meredith SN, Humphries P, Sherman BS, Koehn JD, Watts RJ, Ingram BA, Ryan T (2018) Flow alteration and thermal pollution depress modelled growth rates of an iconic riverine fish, the Murray cod Maccullochella peelii. Ecology of Freshwater Fish 27, 686-698.
| Crossref | Google Scholar |
Wu SY, Cao W, Wang HM, Sun SK, Chen QW (2011) Research on the multi-level intake water temperature effect of the Yalong River Jinping-I hydropower project. Science China Technological Sciences 54, 125-132.
| Crossref | Google Scholar |
Zampatti BP, Strawbridge A, Thiem J,Tonkin Z, Mass R,Woodhead J, Fredberg J (2018) Golden perch (Macquaria ambigua) and silver perch (Bidyanus bidyanus) age demographics, natal origin and migration history in the River Murray, Australia. SARDI Publication Number F2018/000116-1, SARDI Research Report Series Number 993, South Australian Research and Development Institute (Aquatic Sciences), Adelaide, SA, Australia.
Zarri LJ, Danner EM, Daniels ME, Palkovacs EP (2019) Managing hydropower dam releases for water users and imperiled fishes with contrasting thermal habitat requirements. Journal of Applied Ecology 56, 2423-2430.
| Crossref | Google Scholar |