Starch and sucrose metabolism plays an important role in the stem development in Medicago sativa
Jierui Wu







A
B
Abstract
The forage quality of alfalfa (Medicago sativa) stems is greater than the leaves. Sucrose hydrolysis provides energy for stem development, with starch being enzymatically converted into sucrose to maintain energy homeostasis. To understand the physiological and molecular networks controlling stem development, morphological characteristics and transcriptome profiles in the stems of two alfalfa cultivars (Zhungeer and WL168) were investigated. Based on transcriptome data, we analysed starch and sugar contents, and enzyme activity related to starch-sugar interconversion. Zhungeer stems were shorter and sturdier than WL168, resulting in significantly higher mechanical strength. Transcriptome analysis showed that starch and sucrose metabolism were significant enriched in the differentially expressed genes of stems development in both cultivars. Genes encoding INV, bglX, HK, TPS and glgC downregulated with the development of stems, while the gene encoding was AMY upregulated. Weighted gene co-expression network analysis revealed that the gene encoding glgC was pivotal in determining the variations in starch and sucrose contents between the two cultivars. Soluble carbohydrate, sucrose, and starch content of WL168 were higher than Zhungeer. Enzyme activities related to sucrose synthesis and hydrolysis (INV, bglX, HK, TPS) showed a downward trend. The change trend of enzyme activity was consistent with gene expression. WL168 stems had higher carbohydrate content than Zhungeer, which accounted for more rapid growth and taller plants. WL168 formed hollow stems were formed during rapid growth, which may be related to the redistribution of carbohydrates in the pith tissue. These results indicated that starch and sucrose metabolism play important roles in the stem development in alfalfa.
Keywords: developmental stage, enzymatic activity, hollow, Medicago sativa L., plant morphology, starch–sugar interconversion, stems, transcriptome.
Introduction
Alfalfa (Medicago sativa) is an important legume forage with high feeding value and economic value. In China, the cultivated area of alfalfa is very extensive, and the demand for high-quality and high-yield alfalfa cultivars is urgent. The stems and leaves of alfalfa are the main feeding materials, but the biomass of the stems accounts for a larger proportion (Engels and Jung 1998). With the gradual maturity of alfalfa, the stem-leaf ratio gradually increased (Sheaffer et al. 2000). The alfalfa stem is mainly composed of cell wall (Doblin et al. 2010). The main components of the cell wall are polysaccharides, followed by proteins and lipids. Carbohydrates not only affect the nutritional value of plants, but also release energy during hydrolysis to promote plant growth. Carbohydrate is the main product of photosynthesis, and its accumulation is the basis of stem development (Wu et al. 2019).
Starch and sucrose metabolism is a complex biochemical process, which requires the synergistic effect of multiple enzymes (Liu et al. 2021). Sucrose, the primary organic carbon source, is transported from photosynthetic leaves to non-photosynthetic tissues via the phloem. Upon removal of the phloem, sucrose must undergo hydrolysis into glucose and fructose by invertase (INV), or degradation into UDPglucose and fructose by sucrose synthase (SUS). Additionally, sucrose can be degraded into hexose for various purposes (Mathan et al. 2021). Through INV or SUS-mediated sucrose metabolism and related transport processes that produce hexose and its derivatives, an energy source is provided while simultaneously supplying a carbon skeleton for synthesising substances such as starch and cellulose (Gao et al. 2018a). Sucrose undergoes hydrolysis and is converted into glucose 1-phosphate through a series of reactions. Glucose 1-phosphate is considered the most efficient precursor for starch synthesis (Neuhaus and Emes 2000). ADPglucose and pyrophosphate are then formed from glucose 1-phosphate through the action of ADPglucose pyrophosphorylase (AGPase). Starch is composed of two glucose polymers: (1) amylose; and (2) amylopectin. ADPglucose is a substrate for the biosynthesis of two types of starch (Gao et al. 2018b). Amylose formation is mediated by granule-bound starch synthase (GBSS), while soluble starch synthase (SS), starch branching enzyme (SBE) and starch debranching enzyme (DBEs) catalyse amylopectin formation (Jeon et al. 2010).
Recently, genes involved in sucrose and starch biosynthesis have been reported in some plant species, such as potato (Solanum tuberosum) (Lin et al. 2019), rice (Oryza sativa) (Zhu et al. 2020), chestnut (Castanea mollissima) (Li et al. 2020) and lily bulbs (Lilium davidii) (Li et al. 2022). Important progress has been made in the study of the structure, properties and functions of these genes. Studies have demonstrated that OsSUS1 and OsSUS3 contribute to the biosynthesis of rice seed starch (Cho et al. 2011). During the development of Cardiocrinum giganteum bulbs, INVNH (invertase inhibitor) is downregulated and regulates sucrose metabolism together with INV (Zhao et al. 2022). There are three types of INV, which are located in cell wall, cytoplasm and vacuole, named CWIN, CIN and VIN, respectively. Inhibition of CWIN or VIN in carrots (Daucus carota) would produce more leaves (Ruan et al. 2010). CWIN-mediated glucose signalling can activate or enhance the expression of genes responsible for cell division and auxin biosynthesis (LeClere et al. 2010). The overexpression of SUS in cotton has been shown to accelerate leaf formation and expansion from the shoot apex, potentially through the regulation of cell cycle genes via glucose signalling pathways (Xu et al. 2012). Although this information is crucial for elucidating the regulatory mechanism of stem development, there is currently a lack of published data on the identification of starch and sucrose biosynthesis genes in alfalfa stems.
The nutritional superiority of alfalfa cv. WL168 over cv. Zhungeer has been observed in our previous studies (Wu et al. 2022). Starch and soluble sugars are readily assimilated nutrients for animals. Disparities in starch and soluble sugar contents within the stems of Zhungeer and WL168 alfalfa varieties may contribute to variations in nutritional quality between these two cultivars. Starch and sucrose play pivotal roles as energy substrates during alfalfa stem development, potentially influencing plant morphology such as height and growth rate. The interconversion of starch and sucrose necessitates intricate regulation involving diverse enzymes and genes. Discrepancies in enzyme activity and gene expression can give rise to metabolite disparities among different alfalfa cultivars. The purpose of this study was to understand the physiological and molecular networks controlling stem development in alfalfa. Morphological index, transcriptome, weighted gene co-expression network analysis (WGCNA), soluble carbohydrate, sucrose and starch content, enzymes activity related to starch-sugar interconversion and genes involved in sucrose and starch were observed and analysed using Zhungeer and WL168 with different stems development stages. Our results may provide a new theoretical basis for understanding the molecular mechanism of sucrose and starch accumulation in alfalfa stems, and provide a new idea for improving the nutritional quality of alfalfa stems.
Materials and methods
Plant materials
Two alfalfa (Medicago sativa L.) cultivars were used in this study: (1) WL168; and (2) Zhungeer. The IVTD (in vitro true digestibility) of stems in WL168 was significantly higher than that of Zhungeer, along with a significantly lower lignin content (Wu et al. 2022). The plants were planted in Hohhot, Inner Mongolia. Water and fertiliser management practices in the experimental field remained consistent. After turning green, alfalfa was cut to stubble height of 4–6 cm. According to the method descibed by Jung and Engels (2022), the alfalfa stem development process was classified into four stages. The four sampling periods were 17, 24, 32 and 85 days after cutting, respectively. Alfalfa in the experimental field was cut on 22 May 2022 to ensure consistent growth rate, and subsequently sampled at the designated stage of stem development following the prescribed sampling procedure. The seventh internode of alfalfa was collected at four different developmental stages, starting from the base and counting upwards. Stage 1 was collected at the point when the seventh internode became visible on the majority of new shoots. Stage 2 was taken when the seventh internode reached approximately half the length of the fifth internode on the same shoot. Stage 3 occurred when the seventh internode achieved equal length to that of the fifth internode. Stage 4 was taken after a period of 53 days from the third sampling date, by which time the seventh internode had fully matured (Fig. 1a). The stem samples used for transcriptome sequencing analysis and physiological determination were immediately flash-frozen in liquid nitrogen and stored at −80°C.
Phenotypes of the seventh internode of two alfalfa cultivars at four developmental stages during the growth cycle. (a) Phenotypes of two alfalfa cultivars, (b) mitre cutting of two alfalfa cultivars. H1–H4 represent the four stages of development in WL168. S1–S4 represent the four stages of development in Zhungeer.
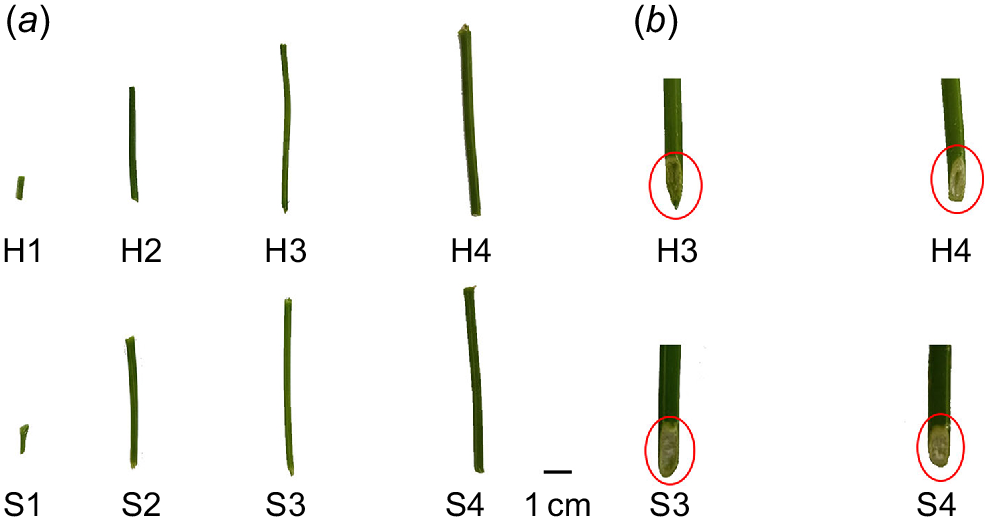
Measurement of stem firmness and morphological index
The mechanical strength is represented by the mechanical strength of second internode (starting from the base and counting upwards). The mechanical strength of the second internode of two alfalfa stems was assessed using a plant stem hardness tester (YYD-1, Tuopu Co., Ltd, Zhejiang, China). Force was applied to the midpoint of the stem segment until the needle tip completely penetrated the epidermis, and the mechanical strength was calculated. Each variety underwent five measurements, and an average value was obtained.
Morphological index encompass the plant height, stem (the seventh internode) diameter, length and weight. According to the four developmental stages, the absolute plant height of alfalfa was measured (in metre scale). Stem diameter and stem length were measured with a vernier calliper. Stem weight was determined using an electronic balance.
Transcriptome analysis
The stem sample (the seventh internode) was subjected to total RNA isolation using an RNA reagent kit (DP441, Tiangen Biotech, Beijing, China). The quality and quantity of the isolated total RNA were assessed through NanoDrop 2000 analysis and gel electrophoresis. Biomarker Technologies Co., Ltd (Beijing, China) conducted cDNA library construction and transcriptome sequencing. A total of 24 libraries were sequenced on the Illumina Hi-seq4000 platform. The raw sequence reads have been deposited in the NCBI Sequence Read Archive (https://www.ncbi.nlm.nih.gov/sra/PRJNA628807). The high-quality clean reads were obtained by removing adaptor sequences and low-quality reads. Subsequently, the clean reads were assembled into transcripts using the Trinity software (https://github.com/trinityrnaseq/trinityrnaseq), resulting in the generation of unigenes (Grabherr et al. 2011).
The overlapping differentially expressed genes (DEGs) between the H1 vs H4 and the S1 vs S4 comparison, the H2 vs H3 and the S2 vs S3 comparison were screened. The analysis of DEGs in the samples was performed using the DEseq 2 software on the BMKCloud platform (www.biocloud.net). A threshold of FC (fold change) ≥ 2 and FDR (False Discovery Rate) < 0.01 was set for identification. Functional annotations of the differentially expressed genes were conducted by comparing unigene sequences with GO databases (http://www.geneontology.org) (Young et al. 2010). Additionally, a gene set enrichment analysis of the differentially expressed genes was carried out to identify over-represented functional pathways (P < 0.05) in KEGG Plant Database (https://www.genome.jp/kegg/genome/plant.html) annotations at each comparison level (for different developmental stages) (Kanehisa and Goto 2000). The WGCNA analysis was performed using the cloud platform at Biomarker Technologies Co., Ltd to identify the core genes in the differential genes.
Determination of soluble carbohydrate, sucrose and starch content
The stem sample (the seventh internode) was subjected to physiological indexes using an reagent kit. The soluble carbohydrate, sucrose and starch content were determined using the test kit of Beijing Solarbio Technology Co., Ltd, China (article number: BC0035 soluble carbohydrate; BC2460 sucrose; BC0700 starch). In brief, the determination of soluble carbohydrate content was conducted using anthrone colorimetry (Liu et al. 2008). The determination principle for sucrose content involves subjecting the sample to heat with NaOH, thereby eliminating reducing sugars. Subsequently, hydrolysis of sucrose into glucose and fructose is carried out under acidic conditions. Finally, a characteristic absorption peak at 480 nm is observed due to the formation of a coloured substance between fructose and resorcinol (Fils-Lycaon et al. 2011). Soluble sugars and starch in the sample were separated using 80% ethanol, followed by acid hydrolysis to convert the starch into glucose. The glucose content was determined through anthrone colorimetry, and the starch content was calculated (Zhang et al. 2015).
Determination of enzyme activity related to starch and sucrose metabolism
The stem sample (the seventh internode) was subjected to enzyme activity using an reagent kit. The invertase (INV), β-glucosidases (bglX), hexokinase (HK) and α-amylase (AMY) activity were determined using the test kit of Beijing Solarbio Technology Co., Ltd (article number: BC0035 soluble carbohydrate; BC2460 sucrose; BC0700 starch). Trehalose 6-phosphate synthase (TPS) was determined using the test kit of Shanghai Fusheng Industrial Co., Ltd, China (article number: AS012147 TPS). The kit is designed to determine the concentration of reducing sugar catalysed by INV using the 3,5-dinitrosalicylic acid method, thereby obtaining the hydrolysis rate of INV (Karley et al. 2005). The hydrolysis of p-nitrophenyl-β-D-glucopyranoside by bglX resulted in the formation of p-nitrophenol, with its maximum absorption peak observed at 400 nm. The activity of bglX was determined by quantifying the rate of absorbance increase (Martins et al. 2008). HK acts as a catalyst for the synthesis of glucose 6-phosphate, while glucose-6-phosphate dehydrogenase further facilitates the oxidation of glucose 6-phosphate to produce NADPH (nicotinamide adenine dinucleotide phosphate). NADPH exhibits a distinctive absorption peak at 340 nm (Pancera et al. 2006). The AMY exhibits thermal stability, whereas the β-amylase undergoes passivation at 70°C. Consequently, following a water bath at 70°C, only the AMY retains its ability to catalyse starch hydrolysis (Hashemi et al. 2013). The reaction between UDPglucose and glucose 6-phosphate is catalysed by TPS, resulting in the production of trehalose 6-phosphate. Subsequently, trehalose-6-phosphate phosphatase dephosphorylates it to yield trehalose (Paul 2007).
Statistical analysis
The differences in firmness, morphological index, metabolite content, and enzyme activity among the analysed stem tissues of alfalfa were assessed using one-way analysis of variance (ANOVA) with SPSS 21.0 software. A significance level of P < 0.05 was considered statistically significant. Graphs were generated using Sigmaplot 14.0.
Results
Firmness and morphological index of stem development in alfalfa
Alfalfa stems were divided into four developmental stages throughout the growth cycle (Fig. 1a). In a previous study (Wu et al. 2022), it was observed that WL168 forms a hollow structure during Stage 3, while Zhungeer maintains a solid structure throughout the entire development process. To confirm this conclusion, mitre cutting was conducted. We observe that the pith part of WL168 disappeared to form a gap, while the pith part of Zhungeer is complete (Fig. 1b).
Stem firmness of the two alfalfa cultivars gradually increased during the developmental process and peaked at the Stage 4. However, significant disparities in stem firmness were observed between the two alfalfa cultivars at equivalent developmental stages. At Stage 4, the Zhungeer stem firmness was measured at 22.87N, while the WL168 stem firmness was 20.80N. Throughout all stages of development, the firmness of stems in Zhungeer was significantly higher than in WL168 (P < 0.05). Specifically, compared to WL168, the stem firmness in Zhungeer was 27.61% (stage 1), 26.85% (stage 2), 23.11% (stage 3) and 9.95% (stage 4) higher (Fig. 2a).
Firmness and morphological index of stem development in alfalfa. (a) Mechanical strength, (b) plant height, (c) stem length, (d) stem diameter, and (e) stem weight. Different lowercase letters indicate significant differences between the two alfalfa cultivars at the same developmental stage (P < 0.05).
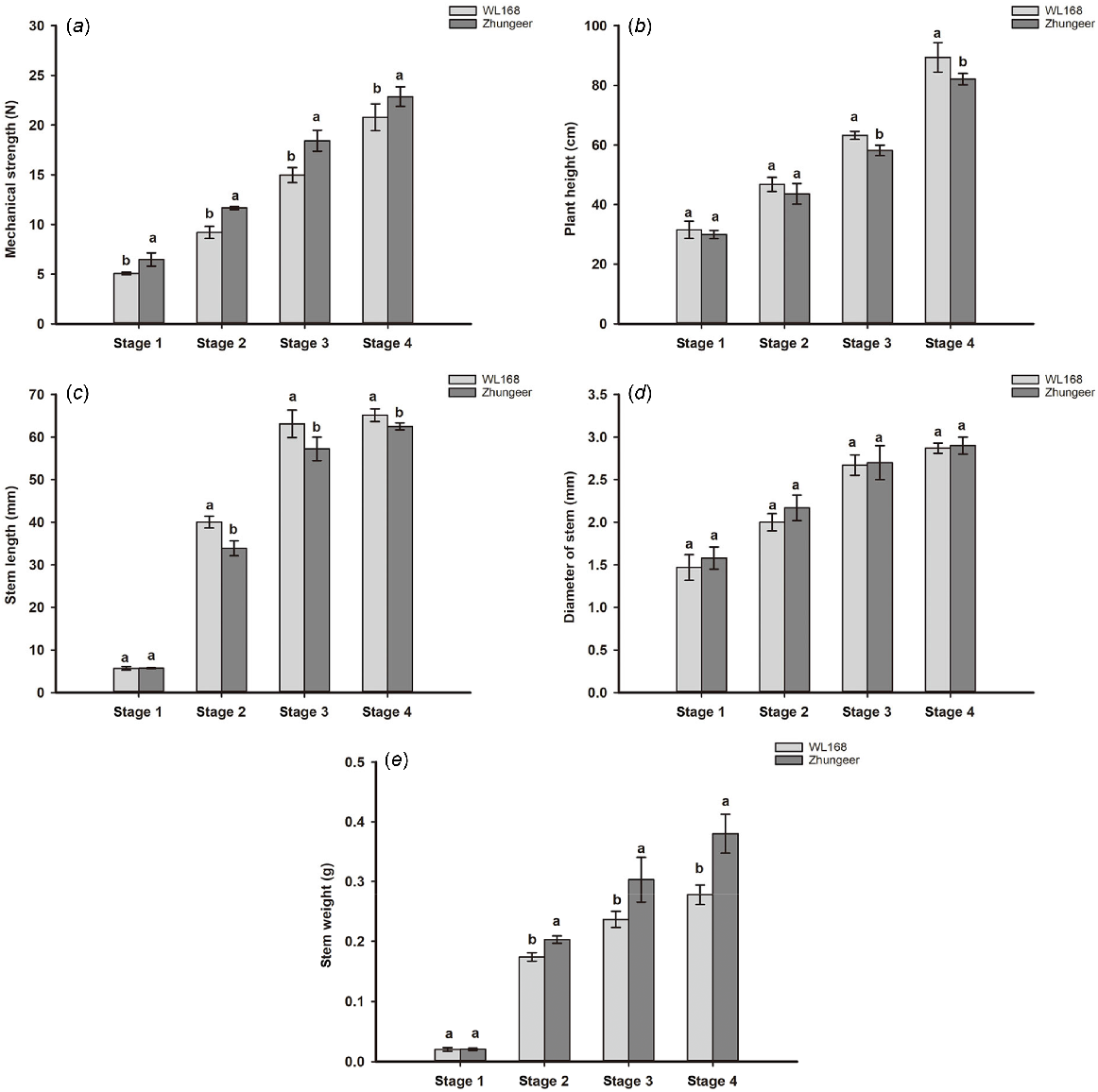
During the development process, the stem of alfalfa exhibit significant changes in morphology, including plant height, stem length, stem diameter and stem weight. A comparison of morphological traits showed that the plant height of WL168 was significantly higher than Zhungeer in Stage 3–4 (Fig. 2b), and the stem length of WL168 was significantly higher than Zhungeer in Stage 2–4 (P < 0.05) (Fig. 2c). There was no significant difference in stem diameter between the two alfalfa cultivars at four developmental stages (Fig. 2d). The stem weight of Zhungeer was significantly higher than WL168 in Stage 2–4 (P < 0.05) (Fig. 2e). In conclusion, the stem of Zhungeer is shorter but firmer compared to WL168.
Comparative transcriptomic analysis of the stems in two alfalfa cultivars
Transcriptome sequencing was carried out on stems of the two alfalfa cultivars in four developmental stages to reveal the molecular networks controlling stems development in alfalfa. A total of 24 libraries were constructed with three biological replicates in each group. There were 1636, 3723, 23 and 30 DEGs in the H1 vs H4, S1 vs S4, H2 vs H3, and S2 vs S3 comparisons, respectively. A total of 721 overlapping DEGs between the H1 vs H4 and the S1 vs S4 comparison, but none in the H2 vs H3, and the S2 vs S3 comparison (Fig. 3).
Venn diagram of overlapping DEGs between the H1 vs H4 and the S1 vs S4 comparison, the H2 vs H3 and the S2 vs S3 comparison.
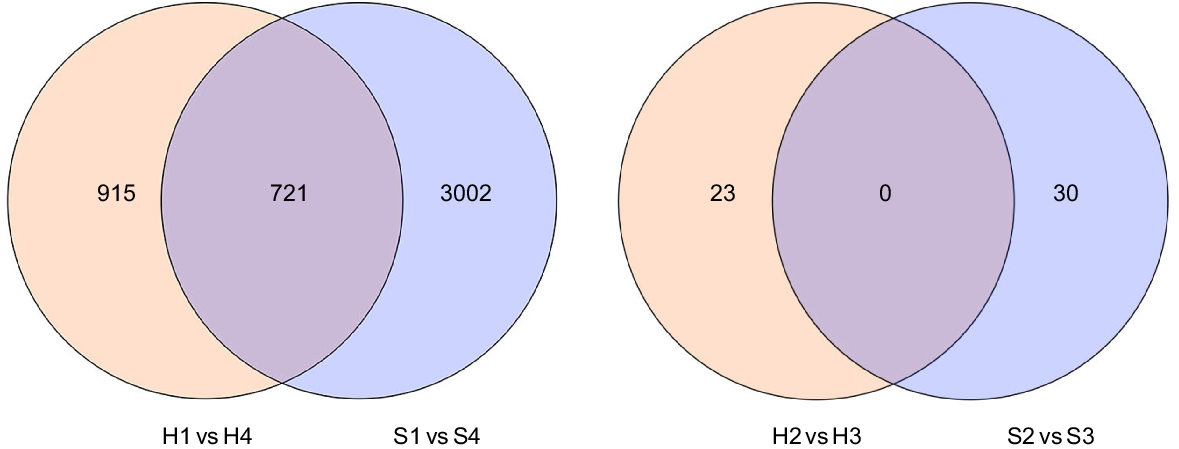
To further understand the function of the 721 overlapping DEGs in both cultivars, DEGs sequences were searched the GO database for functional prediction. The GO terms were divided into biological processes, cellular component and molecular function. In the biological processes category, most of the DEGs were assigned to carbohydrate metabolic process (21 unigenes), cell wall organisation (11 unigenes), cell wall organisation or biogenesis (eight unigenes) (Fig. 4a). For the cellular component category, integral component of membrane (175 unigenes) was the most highly represented groups, followed by plasma membrane (34 unigenes) and Golgi apparatus (14 unigenes) (Fig. 4b). In the molecular function category, carbohydrate binding (14 unigenes) was the most highly represented group. In addition, many enzymes related to carbohydrate were annotated, including beta−galactosidase activity, xyloglucan: xyloglucosyl transferase activity, polygalacturonase activity (Fig. 4c).
GO classification of DEGs. (a) Biological process, (b) cellular component, and (c) molecular function.
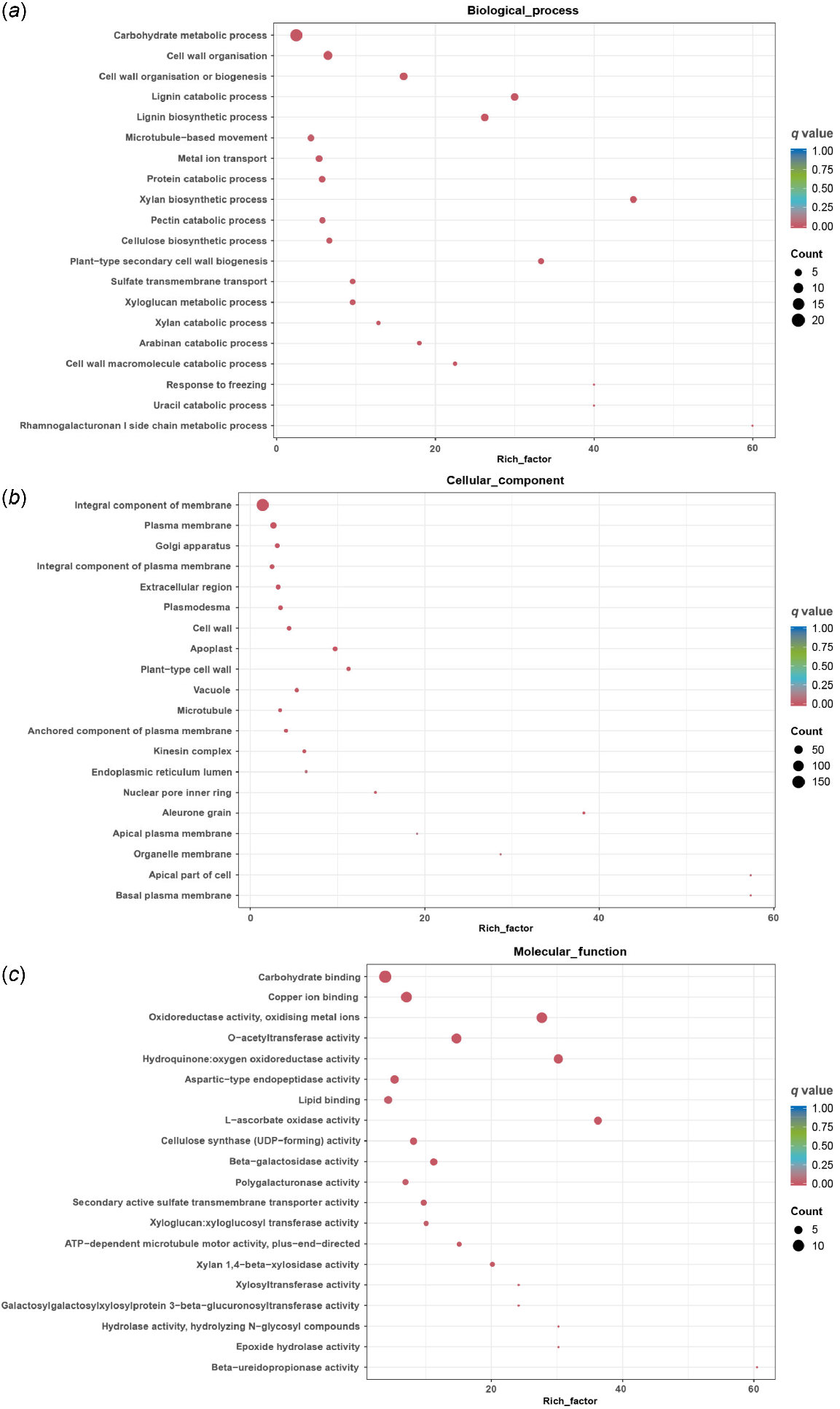
Furthermore, KEGG annotations were used to determine the metabolic pathways involved in the DEGs during different development stages. A total of 160 DEGs were grouped into 57 pathways. Among these pathways, the starch and sucrose metabolism enriched most genes (15 unigenes) and showed significant enrichment (P < 0.05) (Fig. 5).
KEGG pathway enrichment of DEGs. We have obtained a copyright licence to use the KEGG image depicted.
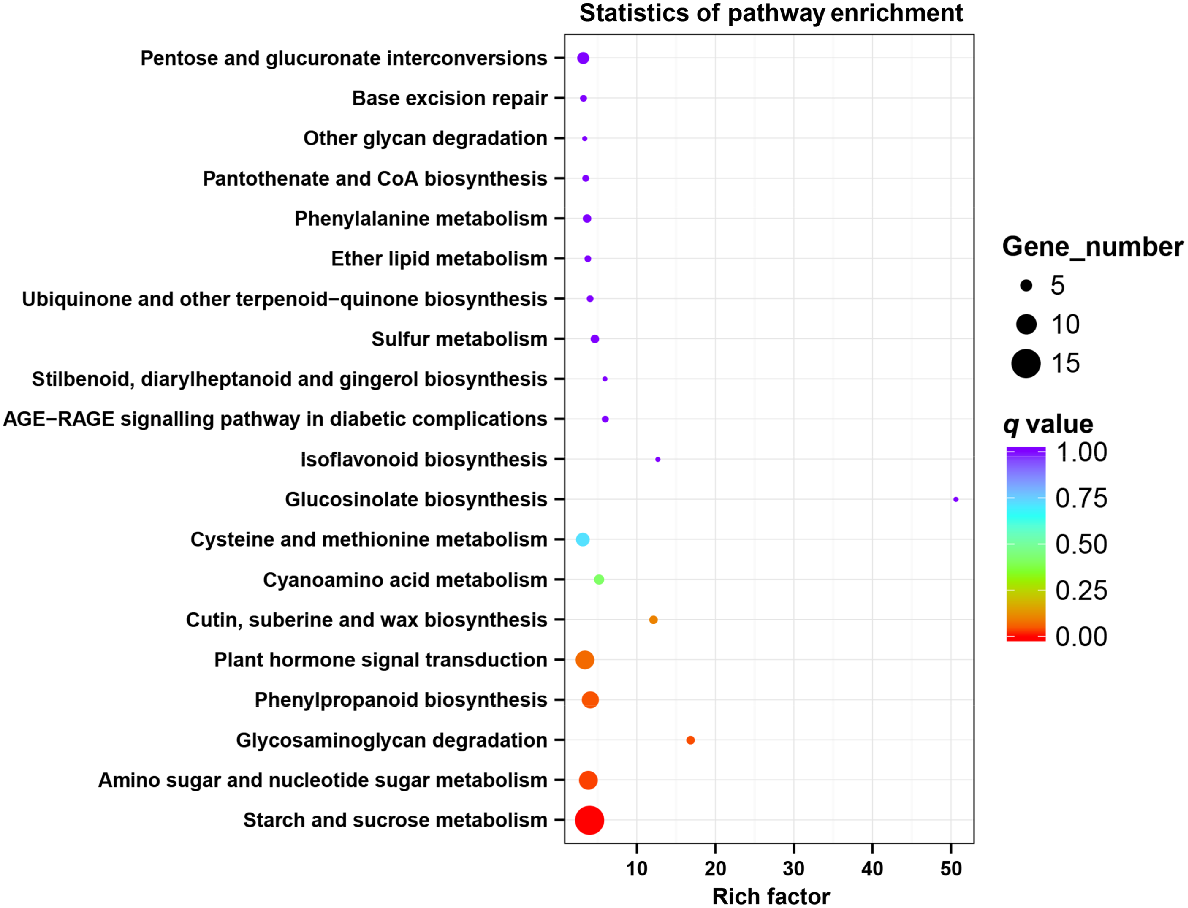
To identify key genes involved in the sucrose and starch pathways, a total of 721 overlapping DEGs were subjected to WGCNA. The gene expression was subjected to cluster analysis, and genes exhibiting a high degree of clustering were assigned to specific modules. The study yielded a total of nine gene co-expression modules, with the turquoise module exhibiting the highest number of genes (167 genes), followed by the green modules (114 genes), and then blue modules (111 genes) (Fig. 6a). The correlation between the nine gene co-expression modules and the phenotypic traits of two alfalfa cultivars was investigated. Results demonstrate that the black module exhibits the highest correlation with S4 (R = 0.84), while the turquoise module displays the strongest association with H1 (R = 0.67). As the alfalfa matures (one to four developmental stage), the black and magenta modules exhibited upregulation of DEGs in both alfalfa cultivars, while downregulation of DEGs was observed in other modules. Genes associated with the biosynthesis of starch and sucrose were identified within the brown, pink, green, blue, red and magenta module, the red module exhibits the highest correlation coefficient (R = 0.65) (Fig. 6b). Further mining of the red module, we identified the gene involved in starch and sucrose metabolism in the module as glgC.
WGCNA of DEGs. (a) Gene number of each module, (b) correlation analysis between modules and traits. The abscissa represents different phenotypes, and the ordinate represents different modules. Each set of data in the figure shows the correlation coefficient R-value and significance P-value between the module and the phenotype (in parentheses).
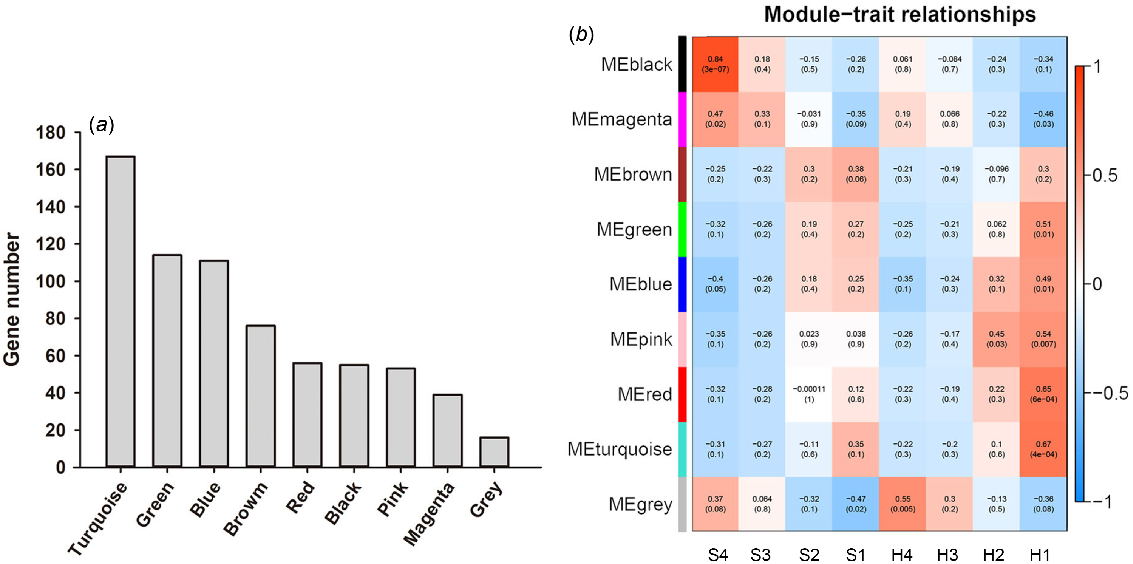
Genes related to starch and sucrose metabolism
Starch and sucrose are the two predominant carbohydrates found in plants. They serve a crucial function in energy storage and supply within the plant. To explore further details on the molecular mechanism of starch-sugar interconversion in alfalfa stems, we selected DEGs in the starch and sucrose metabolism to perform a detailed analysis. We identified eight candidate enzyme genes implicated in the starch-sugar interconversion of alfalfa stems during the developmental process. These genes mainly encode key enzymes such as INV (invertase, one unigene), bglX (β-glucosidases, two unigenes), HK (hexokinase, one unigene), TPS (trehalose-6-phosphate synthase, two unigenes), glgC (glucose-1-phosphate adenylyltransferasewere, one unigene) and AMY (α-amylase, one unigene). The results showed that genes encoding INV, bglX, HK, TPS and glgC downregulated gradually with the development of stems in both cultivars, while the gene encoding AMY upregulated (Fig. 7).
Soluble carbohydrate, sucrose and starch content of stem development in alfalfa
Soluble carbohydrate, sucrose and starch content of the stem development was further examined based on the transcriptome sequencing findings. During the process of stem development in the two alfalfa cultivars, the content of soluble carbohydrate and sucrose were decreased during the Stage 1–3 but then increased at Stage 4. Soluble carbohydrate content of stems in WL168 was significantly higher than Zhungeer in all development stages (P < 0.05). Sucrose content of stems in WL168 was significantly higher than Zhungeer in Stages 1, 2 and 4. (P < 0.05). The accumulation patterns of soluble carbohydrate and sucrose were similar. Starch content gradually increased during stem development, and the starch content of WL168 was significantly higher than Zhungeer in Stages 1 and 4 (P < 0.05) (Fig. 8).
Enzyme activity related to starch and sucrose metabolism
Based on the above transcriptome sequencing and metabolite content, we further measured the enzyme activity related to starch-sugar interconversion. The activity of INV, bglX, TTPS and HK were decreased gradually with the stem development in both cultivars. The enzyme activities of the two alfalfa cultivars exhibited significant disparities at the identical developmental stage (P < 0.05). Compared with WL168, INV activity of Zhungeer was higher, and there was a significant difference between the two alfalfa cultivars in Stage 1–3 (P < 0.05). bglX activity decreased rapidly from Stage 1 to Stage 2. TPS activity of WL168 was significantly higher than Zhungeer in Stages 1 and 3 (P < 0.05). HK activity of WL168 was significantly higher than Zhungeer in Stages 1 and 4, but it was opposite in Stage 3 (P < 0.05). AMY activity increased and then decreased, with highest activity in Stage 3. AMY activity of WL168 was significantly lower than Zhungeer in Stages 3 and 4 (Fig. 9).
Discussion
Alfalfa is a high-quality legume forage, with the stem providing the majority of nutritional quality and biomass (Lamb et al. 2012). The nutritional quality differences between the two alfalfa cultivars have been investigated in our previous studies, and WL168 exhibits a higher nutritional value than Zhungeer (Wu et al. 2022). The mitre cutting experiment confirmed the formation of a hollow structure in Stage 3 for WL168, while Zhungeer consistently exhibited a solid structure. This finding is consistent with our prior research (Wu et al. 2022). The mechanical strength of the stems exhibited significant variations between the two alfalfa cultivars throughout their developmental stages. The height of a plant is determined by the number and length of its internodes. The mechanical strength of stem is closely related to plant morphology (Navabi et al. 2006). In this study, the stems of Zhungeer exhibited shorter yet sturdier characteristics compared to WL168, potentially resulting in a significantly higher mechanical strength for Zhungeer (P < 0.05). Sucrose and starch not only impact the nutritional quality of alfalfa but also influence its growth. Sucrose serves as the primary energy source during alfalfa stem development, while starch decomposition supplements the energy requirements for stem development. The regulation pathways of sucrose and starch play a crucial role in stem development. Exploring the sucrose and starch biosynthesis during stem development is highly significant for the yield and quality of alfalfa.
The transcription profiles of stems from two alfalfa cultivars at four different developmental stages were investigated using the Hi-seq 4000 sequencing platform. A total of 721 overlapping DEGs between H1 vs H4 and S1 vs S4 comparisons. The GO functional annotation of 721 overlapping DEGs revealed that the majority of these genes were assigned to carbohydrate metabolism in biological processes. The KEGG pathway analysis showed that DEGs were significantly enriched in starch and sucrose metabolism. These results suggest that the starch-sugar interconversion plays an important role in stem development. Furthermore, we identified eight candidate enzyme genes implicated in the starch-sugar interconversion of alfalfa stems during the developmental process. These genes mainly encode key enzymes such as INV (one unigene), bglX (two unigenes), HK (one unigene), TPS (two unigenes), glgC (one unigene) and AMY (one unigene). Results showed that genes encoding INV, bglX, HK, TPS and glgC downregulated gradually with the development of stems in both cultivars, while the gene encoding AMY upregulated. INV, bglX, HK, TPS are mainly involved in sucrose hydrolysis and synthesis (Zhao et al. 2022). glgC is the core component of starch biosynthesis (Smith and Zeeman 2020). AMY is one of the main enzymes that catalyse the hydrolysis of starch, glycogen and α−1,4-glycosidic linkages (Huang et al. 2019). The results of the WGCNA revealed that a total of 721 DEGs were classified into nine distinct modules. Excluding the grey module, six modules exhibited downregulation of differential genes that displayed a positive correlation with H1, while two modules showed upregulation and a positive correlation with S4. The expression profiles of DEGs in the starch and sucrose pathways were consistent with the findings obtained from the WGCNA. The WGCNA revealed that the gene encoding glgC played a pivotal role in determining the variations in starch and sucrose contents between the two alfalfa cultivars.
Based on the results of transcriptome sequencing, the contents of soluble carbohydrates, sucrose and starch during stem development were further detected. During the process of stem development in two alfalfa cultivars, the content of soluble carbohydrate and sucrose were decreased during Stage 1–3 but then increased at Stage 4. The reduction in soluble carbohydrates is among the factors contributing to the decline in feeding value, as forage undergoes growth and development (Blaser 1964). Soluble carbohydrate, sucrose, and starch content of WL168 were higher in comparison to Zhungeer. This explains the reason for the difference in quality between the two alfalfa cultivars. The presence of soluble carbohydrate in plants not only impacts quality, but also plays a crucial role in responding to various abiotic stresses (Gill et al. 2003). In this study, soluble carbohydrates and sucrose exhibited a gradual decline during the rapid growth phase of stems, followed by an accumulation at maturity. Conversely, starch content demonstrated a continuous increase throughout the developmental process. This indicates that starch and sucrose maintain energy stability through mutual conversion during stem development. During the initial stage of stem development, WL168 exhibited significantly higher levels of soluble carbohydrate, sucrose, and starch than Zhungeer. This disparity in initial energy levels may give rise to variations in the growth rate of alfalfa, potentially impacting plant morphology such as stature and stem elongation. We further measured the enzyme activity related to starch-sugar interconversion of stem development. The enzyme activities related to sucrose synthesis and hydrolysis showed a downward trend, including INV, bglX, HK and TPS. AMY activity showed a trend of increasing and then decreasing, and the enzyme activity was the highest in Stage 3. Stage 1–3 represents a phase of rapid growth for alfalfa stems, characterised by a high rate of sucrose and starch conversion, leading to an increase in AMY activity. During Stage 3–4, stems gradually matured as various metabolites accumulate while enzyme activity declines. The change trend of enzyme activity was basically consistent with that of gene expression. Starch serves as a crucial reservoir of carbohydrates in stems, while sucrose acts as the primary soluble carbohydrate form responsible for carbon source distribution and sugar signal initiation (Chang et al. 2013). The process of sucrose hydrolysis provides energy for stem development, with starch being enzymatically converted into sucrose to maintain energy homeostasis (Yang et al. 2017). The mutual transformation process of starch and sucrose necessitates the synergistic regulation of multiple enzymes. The granule-bound starch synthase (GBSS) plays a crucial role in the biosynthesis of amylose (Zhang et al. 2020). The soluble starch synthase (SSS) primarily participates in the biosynthesis of amylopectin and plays a pivotal role in enhancing the structural integrity and quality of starch (Smith and Zeeman 2020). Sucrose synthase (SuS) exhibits reversible activity and mediates the synthesis and degradation of sucrose, thereby playing a crucial role in regulating carbohydrate distribution, structural composition, and storage within plant cells (Fugate et al. 2019).
The formation process of the hollow stem is that a small elliptical crack appears in the pith tissue of the stem, and one or more of these elliptical cracks expand to form a cavity (Griffith and Carling 1991). Two mechanisms have been proposed for the formation of hollow stems: (1) pith tissue autolysis; and (2) radial strain caused by rapid growth of stems (Boersma et al. 2013). The incidence of hollow stems is closely related to the rapid growth of plants (Griffith and Carling 1991). The hollow stem is produced during the early development of the inflorescence and starts from the top of the stem. In the study of beans and tomatoes, it has been proposed that the redistribution of carbohydrates is the cause of pith degeneration (Carr and Jaffe 1995). In the process of rapid growth of plants, the demand for photosynthetic assimilates exceeds the ability of the photosynthetic system, and carbohydrates in the pith tissue are utilised, finally autolysis of these cells is achieved through programmed cell death. In our study, the stem of WL168 has higher carbohydrate content than Zhungeer, which provides a material basis for the rapid growth of WL168, resulting in high plant height of WL168. Hollow stems were formed during the rapid growth of WL168, which may be related to the redistribution of carbohydrates in the pith tissue. After the degeneration of the pith tissue, the plant can use more nutrients to build mechanical tissue to support plants.
Under the regulation of differential gene expression, the activities of enzymes involved in starch and sugar conversion processes exhibited variations, ultimately resulting in disparities in starch and sucrose content between the two alfalfa cultivars. Starch, sucrose and lignin are key determinants of the nutritional value of alfalfa. WL168 exhibits high starch and sucrose content, along with low lignin content, whereas Zhungeer demonstrates the opposite characteristics. Consequently, WL168 possesses superior nutritional quality compared to Zhungeer. Lignin and plant morphology play a crucial role in determining stem mechanical strength. The Zhungeer stem is characterised by its short and sturdy structure, accompanied by a high lignin content, thereby resulting in enhanced mechanical strength. Starch and sucrose not only impact the nutritional quality of alfalfa but also influence its plant height and stem elongation. In comparison to Zhungeer, WL168 exhibits higher levels of starch and sucrose content, resulting in taller plants with longer stem nodes. This rapid growth rate leads to the development of a hollow structure in WL168. The disparity in starch and sucrose content between the two alfalfa varieties primarily arises from differential expression of INV, bglX, HK, TPS, glgC and AMY genes. By identifying and screening these differentially expressed genes, we hypothesise that glgC may serve as the core differential gene. Furthermore, enzyme activity measurements provide further validation for consistency between trend of enzyme activity changes and gene expression related to starch-sucrose conversion. However, functional verification of these differentially expressed genes requires further exploration. Our study offers a theoretical foundation for enhancing both nutritional quality and yield of alfalfa.
Conclusion
The stems of Zhungeer were shorter but sturdier than WL168, potentially resulting in a significantly higher mechanical strength in Zhungeer. Transcriptome analysis showed that the starch and sucrose metabolism were significant enriched in the DEGs of stems development of both cultivars. In details, the INV, bglX, HK, TPS and glgC genes downregulated gradually with the development of stems in both cultivars, while the AMY gene upregulated. The WGCNA analysis revealed that the gene encoding glgC played a pivotal role in determining the variations in starch and sucrose contents between the two alfalfa cultivars. In accordance with the gene expression, the enzyme activities related to sucrose synthesis and hydrolysis including INV, bglX, HK and TPS also showed a downward trend with the development of stems. Soluble carbohydrate, sucrose, and starch content of WL168 were higher than Zhungeer, which provides a material basis for the rapid growth of WL168, resulting in high plant height of WL168. Hollow stems were formed during the rapid growth of WL168, which may be related to the redistribution of carbohydrates in the pith tissue. Our results may provide a new theoretical basis for understanding the molecular mechanism of sucrose and starch accumulation in alfalfa stems.
Data availability
The datasets presented in this study can be found in online repositories. The names of the repository/repositories and accession number(s) can be found at: https://www.ncbi.nlm.nih.gov/search/all/?term=PRJNA628807.
Declaration of funding
This work was supported by Pratacultural Science Youth Fund of Inner Mongolia Agricultural University, China (IMAUCXQJ2023004), Inner Mongolia Seed Industry Science and technology innovation major demonstration project (2022JBGS0016), Fundamental scientific research funds of Inner Mongolia, China (BR22-11-12), Youth Science and Technology Talent Support Project of Inner Mongolia, China (NJYT23009).
Author contributions
Zhiqiang Zhang designed and performed the experiments. Jierui Wu, Xiaoyu Wang and Lin Bian performed the main experiments. Zhenyi Li and Xiaohong Jiang contributed to data analysis. Fengling Shi and Fang Tang provided technical and material support. Jierui Wu wrote the manuscript. Zhiqiang Zhang revised the manuscript. All authors reviewed and approved the manuscript.
References
Blaser RE (1964) Symposium on forage utilization: effects of fertility levels and stage of maturity on forage nutritive value. Journal of Animal Science 23(1), 246-253.
| Crossref | Google Scholar |
Boersma M, Gracie AJ, Brown PH (2013) Evidence of mechanical tissue strain in the development of hollow stem in broccoli. Scientia Horticulturae 164, 353-358.
| Crossref | Google Scholar |
Carr SM, Jaffe MJ (1995) Pith autolysis in herbaceous, dicotyledonous plants: experimental manipulation of pith autolysis in several cultivated species. Annals of Botany 75, 587-592.
| Crossref | Google Scholar |
Chang L, Xiao YM, She LF, Xia YP (2013) Analysis of gene expression and enzyme activities related to starch metabolism in Lycoris sprengeri bulbs of different sizes. Scientia Horticulturae 161, 118-124.
| Crossref | Google Scholar |
Cho J-I, Kim H-B, Kim C-Y, Hahn T-R, Jeon J-S (2011) Identification and characterization of the duplicate rice sucrose synthase genes OsSUS5 and OsSUS7 which are associated with the plasma membrane. Molecules and Cells 31, 553-562.
| Crossref | Google Scholar | PubMed |
Doblin MS, Pettolino F, Bacic A (2010) Plant cell walls: the skeleton of the plant world. Functional Plant Biology 37(5), 357-381.
| Crossref | Google Scholar |
Engels FM, Jung HG (1998) Alfalfa stem tissues: cell-wall development and lignification. Annals of Botany 82(5), 561-568.
| Crossref | Google Scholar |
Fils-Lycaon B, Julianus P, Chillet M, Galas C, Hubert O, Rinaldo D, Mbéguié-A-Mbéguié D (2011) Acid invertase as a serious candidate to control the balance sucrose versus (glucose+fructose) of banana fruit during ripening. Scientia Horticulturae 129(2), 197-206.
| Crossref | Google Scholar |
Fugate KK, Eide JD, Martins DN, Grusak MA, Deckard EL, Finger FL (2019) Colocalization of sucrose synthase expression and sucrose storage in the sugarbeet taproot indicates a potential role for sucrose catabolism in sucrose accumulation. Journal of Plant Physiology 240, 153016.
| Crossref | Google Scholar | PubMed |
Gao S, Zhu Y, Zhou L, Fu X, Lei T, Chen Q, et al. (2018) Sucrose signaling function on the formation and swelling of bulblets of Lilium sargentiae E.H. Wilson. Plant Cell, Tissue and Organ Culture (PCTOC) 135, 143-153.
| Crossref | Google Scholar |
Gao M, Zhang S, Luo C, He X, Wei S, Jiang W, et al. (2018b) Transcriptome analysis of starch and sucrose metabolism across bulb development in Sagittaria sagittifolia. Gene 649, 99-112.
| Crossref | Google Scholar | PubMed |
Gill PK, Sharma AD, Singh P, Bhullar SS (2003) Changes in germination, growth and soluble sugar contents of Sorghum bicolor (L.) Moench seeds under various abiotic stresses. Plant Growth Regulation 40, 157-162.
| Crossref | Google Scholar |
Grabherr MG, Haas BJ, Yassour M, Levin JZ, Thompson DA, Amit I, et al. (2011) Full-length transcriptome assembly from RNA-Seq data without a reference genome. Nature Biotechnology 29(7), 644-652.
| Crossref | Google Scholar | PubMed |
Griffith M, Carling DE (1991) Effects of plant spacing on broccoli yield and hollow stem in Alaska. Canadian Journal of Plant Science 71, 579-585.
| Crossref | Google Scholar |
Hashemi M, Mousavi SM, Razavi SH, Shojaosadati SA (2013) Comparison of submerged and solid state fermentation systems effects on the catalytic activity of Bacillus sp. KR-8104 α-amylase at different pH and temperatures. Industrial Crops and Products 43, 661-667.
| Crossref | Google Scholar |
Huang Q, Xu M, Zhang H, He D, Kong Y, Chen L, Song H (2019) Transcriptome and proteome analyses of the molecular mechanisms associated with coix seed nutritional quality in the process of breeding. Food Chemistry 272, 549-558.
| Crossref | Google Scholar | PubMed |
Jeon J-S, Ryoo N, Hahn T-R, Walia H, Nakamura Y (2010) Starch biosynthesis in cereal endosperm. Plant Physiology and Biochemistry 48(6), 383-392.
| Crossref | Google Scholar | PubMed |
Jung HG, Engels FM (2002) Alfalfa stem tissues: cell wall deposition, composition, and degradability. Crop Science 42(2), 524-534.
| Crossref | Google Scholar |
Kanehisa M, Goto S (2000) KEGG: kyoto encyclopedia of genes and genomes. Nucleic Acids Research 28(1), 27-30.
| Crossref | Google Scholar | PubMed |
Karley AJ, Ashford DA, Minto LM, Pritchard J, Douglas AE (2005) The significance of gut sucrase activity for osmoregulation in the pea aphid, Acyrthosiphon pisum. Journal of Insect Physiology 51(12), 1313-1319.
| Crossref | Google Scholar | PubMed |
Lamb JFS, Hans-Joachim GJ, Riday H (2012) Harvest impacts on alfalfa stem neutral detergent fiber concentration and digestibility and cell wall concentration and composition. Crop Science 52(5), 2402-2412.
| Crossref | Google Scholar |
LeClere S, Schmelz EA, Chourey PS (2010) Sugar levels regulate tryptophan-dependent auxin biosynthesis in developing maize kernels. Plant Physiology 153(1), 306-318.
| Crossref | Google Scholar | PubMed |
Li S, Liang H, Tao L, Xiong L, Liang W, Shi Z, Zhao Z (2020) Transcriptome sequencing and differential expression analysis reveal molecular mechanisms for starch accumulation in chestnut. Forests 11(4), 388.
| Crossref | Google Scholar |
Li W, Huang D, Wang B, Hou X, Zhang R, Yan M, Liao W (2022) Changes of starch and sucrose content and related gene expression during the growth and development of Lanzhou lily bulb. PLoS ONE 17(1), e0262506.
| Crossref | Google Scholar | PubMed |
Lin Q, Xie Y, Guan W, Duan Y, Wang Z, Sun C (2019) Combined transcriptomic and proteomic analysis of cold stress induced sugar accumulation and heat shock proteins expression during postharvest potato tuber storage. Food Chemistry 297, 124991.
| Crossref | Google Scholar | PubMed |
Liu T, Hu Y, Li X (2008) Comparison of dynamic changes in endogenous hormones and sugars between abnormal and normal Castanea mollissima. Progress in Natural Science 18(6), 685-690.
| Crossref | Google Scholar |
Liu X, Chen L, Shi W, Xu X, Li Z, Liu T, et al. (2021) Comparative transcriptome reveals distinct starch-sugar interconversion patterns in potato genotypes contrasting for cold-induced sweetening capacity. Food Chemistry 334, 127550.
| Crossref | Google Scholar | PubMed |
Martins LF, Kolling D, Camassola M, Dillon AJP, Ramos LP (2008) Comparison of Penicillium echinulatum and Trichoderma reesei cellulases in relation to their activity against various cellulosic substrates. Bioresource Technology 99(5), 1417-1424.
| Crossref | Google Scholar | PubMed |
Mathan J, Singh A, Ranjan A (2021) Sucrose transport and metabolism control carbon partitioning between stem and grain in rice. Journal of Experimental Botany 72(12), 4355-4372.
| Crossref | Google Scholar | PubMed |
Navabi A, Iqbal M, Strenzke K, Spaner D (2006) The relationship between lodging and plant height in a diverse wheat population. Canadian Journal of Plant Science 86(3), 723-726.
| Crossref | Google Scholar |
Neuhaus HE, Emes MJ (2000) Nonphotosynthetic metabolism in plastids. Annual Review of Plant Biology 51(1), 111-140.
| Crossref | Google Scholar |
Pancera SM, Gliemann H, Schimmel T, Petri DFS (2006) Adsorption behavior and activity of hexokinase. Journal of Colloid and Interface Science 302(2), 417-423.
| Crossref | Google Scholar | PubMed |
Paul M (2007) Trehalose 6-phosphate. Current Opinion in Plant Biology 10(3), 303-309.
| Crossref | Google Scholar | PubMed |
Ruan Y-L, Jin Y, Yang Y-J, Li G-J, Boyer JS (2010) Sugar input, metabolism, and signaling mediated by invertase: roles in development, yield potential, and response to drought and heat. Molecular Plant 3(6), 942-955.
| Crossref | Google Scholar | PubMed |
Sheaffer CC, Martin NP, Lamb JFS, Cuomo GR, Jewett JG, Quering SR (2000) Leaf and stem properties of alfalfa entries. Agronomy Journal 92(4), 733-739.
| Crossref | Google Scholar |
Smith AM, Zeeman SC (2020) Starch: a flexible, adaptable carbon store coupled to plant growth. Annual Review of Plant Biology 71, 217-245.
| Crossref | Google Scholar | PubMed |
Wu Y, Sun M, Zhang J, Zhang L, Ren Z, Min R, et al. (2019) Differential effects of paclobutrazol on the bulblet growth of oriental lily cultured in vitro: growth behavior, carbohydrate metabolism, and antioxidant capacity. Journal of Plant Growth Regulation 38, 359-372.
| Crossref | Google Scholar |
Wu J, Wang X, Zhang R, Fu Q, Tang F, Shi F, et al. (2022) Comparative transcriptome and anatomic characteristics of stems in two alfalfa genotypes. Plants 11(19), 2601.
| Crossref | Google Scholar | PubMed |
Xu S-M, Brill E, Llewellyn DJ, Furbank RT, Ruan Y-L (2012) Overexpression of a potato sucrose synthase gene in cotton accelerates leaf expansion, reduces seed abortion, and enhances fiber production. Molecular Plant 5(2), 430-441.
| Crossref | Google Scholar | PubMed |
Yang P, Xu L, Xu H, Tang Y, He G, Cao Y, et al. (2017) Histological and transcriptomic analysis during bulbil formation in Lilium lancifolium. Frontiers in Plant Science 8, 1508.
| Crossref | Google Scholar | PubMed |
Young MD, Wakefield MJ, Smyth GK, Oshlack A (2010) Gene ontology analysis for RNA-seq: accounting for selection bias. Genome Biology 11(2), R14.
| Crossref | Google Scholar | PubMed |
Zhang L, Lin Q, Feng Y, Fan X, Zou F, Yuan D-Y, Zeng X, Cao H (2015) Transcriptomic identification and expression of starch and sucrose metabolism genes in the seeds of Chinese chestnut (Castanea mollissima). Journal of Agricultural and Food Chemistry 63(3), 929-942.
| Crossref | Google Scholar | PubMed |
Zhang K, Luo K, Li S, Peng D, Tang D, Lu H, et al. (2020) Genetic variation and sequence diversity of starch biosynthesis and sucrose metabolism genes in sweet potato. Agronomy 10(5), 627.
| Crossref | Google Scholar |
Zhao Z, Li R, Wang X, Liang W, Liao J, Huang X, et al. (2022) The starch-sugar interconversion mechanism during bulb development of Cardiocrinum giganteum (Wall.) Makino revealed by transcriptome and metabolite analysis. Industrial Crops and Products 187, 115318.
| Crossref | Google Scholar |
Zhu J, Yu W, Zhang C, Zhu Y, Xu J, Li E, et al. (2020) New insights into amylose and amylopectin biosynthesis in rice endosperm. Carbohydrate Polymers 230, 115656.
| Crossref | Google Scholar | PubMed |