Leaf formononetin content of Trifolium subterraneum increases in response to waterlogging but its proportion of total isoflavones is little changed
Gereltsetseg Enkhbat

A UWA School of Agriculture and Environment and Institute of Agriculture, The University of Western Australia, 35 Stirling Highway, Crawley, WA 6009, Australia.
B Graduate School of Bioagricultural Science at Nagoya University, Furo-cho, Chikusa-ku, Nagoya, Japan.
Functional Plant Biology 50(6) 507-518 https://doi.org/10.1071/FP22151
Submitted: 5 July 2022 Accepted: 13 April 2023 Published: 5 May 2023
© 2023 The Author(s) (or their employer(s)). Published by CSIRO Publishing. This is an open access article distributed under the Creative Commons Attribution 4.0 International License (CC BY)
Abstract
The isoflavone formononetin (F) impacts livestock fertility and cultivars of the pasture legume Trifolium subterraneum L. (subclover) have been selected for F levels ≤0.2% of leaf dry weight. However, the impact of waterlogging (WL) on isoflavones is little studied. We investigated the response of isoflavones, biochanin A (BA), genistein (G) and F, to WL for: (1) Yarloop (high F) and eight low F cultivars each from subspecies subterraneum, brachycalycinum and yanninicum (Experiment 1); and (2) four cultivars and 12 ecotypes of ssp. yanninicum (Experiment 2). WL impacted F: estimated means increased from 0.19% (control) to 0.31% (WL) in Experiment 1 and from 0.61% to 0.97% in Experiment 2. Isoflavones under WL were highly heritable, particularly F (H2 = 95%). The proportions of BA, G and F were little changed by WL, with strong positive correlations between free-drained and waterlogged treatments. Isoflavone contents were not related to WL tolerance, as assessed by shoot relative growth rate. In conclusion, isoflavones varied among genotypes and increased with WL, but the proportion of individual isoflavones in each genotype was stable. High F under WL was unrelated with genotype tolerance to WL. Instead, it was a consequence of inherently high F for that particular genotype.
Keywords: eco-geographic variables, formononetin, genetic resources, heritability, leaf isoflavones, pasture legumes, subterranean clover, waterlogging.
Introduction
Subterranean clover (subclover; Trifolium subterraneum L.), native to Mediterranean regions, is highly valued for providing nutritious livestock feed with high protein content (Nichols et al. 2013). Subclover is the dominant annual pasture legume in southern Australia with 29 million ha sown (Hill and Donald 1998) and is an essential part of both the livestock and grain industries, where it is often grown in rotation with cereal crops. Subclover is self-pollinated (Nichols et al. 2013) and is comprised of three subspecies, which Katznelson and Morley (1965) classify as: (1) ssp. subterraneum; (2) ssp. brachycalycinum; and (3) ssp. yanninicum. Among these, ssp. yanninicum exhibits a higher tolerance to waterlogging (Enkhbat et al. 2021a) and is mostly grown in medium-high rainfall areas (450–1200 mm mean annual rainfall), typically in fields with poor drainage (Nichols et al. 2013).
Phytoestrogenic isoflavones are commonly found in legumes and are naturally-occurring, genetically-controlled, and highly heritable non-steroidal compounds (Rossiter and Beck 1966; Benassayag et al. 2002; Marley et al. 2011; Reed 2016). Isoflavones are present in all aboveground parts of the plant except the seed, but contents are highest in newly-opened leaves and tend to diminish with the age of both leaf and plant (Morley and Francis 1968; Smith et al. 1986; Marley et al. 2011). Fresh leaves of subclover can contain the isoflavones biochanin A, genistein and formononetin. Consumption of some cultivars of subclover has been shown to reduce reproductive efficiency in herbivores such as sheep and cattle, commonly known as ‘clover disease’ (Bennetts et al. 1946; Guggolz et al. 1961; Hungerford 1975; Brightling 2006). Clover disease has been linked to severe clinical conditions including dystocia (difficult birth), polarised uteri, low conception and post-natal lamb and ewe mortality (Beck and Gardiner 1965; Collins and Cox 1984).
Extensive research shows that high formononetin content in the green leaves is primarily responsible for clover disease in sheep due to its oestrogenic effects. Formononetin itself is not oestrogenic, but is metabolised rapidly by rumen microflora and demethylated to form the oestrogenic isoflavone equol, a molecule that structurally and biologically resembles the major sex steroid, oestradiol-17ß (Braden et al. 1967; Shutt and Braden 1968; Adams 1998; Reed 2016). Biochanin A and genistein are considered of lesser importance (Millington et al. 1964; Shutt and Braden 1968; Adams 1990; Marley et al. 2011). While these are also oestrogenic compounds, they are degraded in the rumen to the non-oestrogenic compound, para-ethanol, after a few days intake of pasture (Cox and Braden 1974). Collins and Cox (1984) and Davies (1986) suggest that formononetin levels <0.3% (on a leaf dry weight basis) are ‘safe’ for sheep reproduction. However, the safe level of formononetin has not been determined precisely. Indeed, Davies (1986) and Adams et al. (1988) found some histological symptoms of clover disease in ewes grazing even low-formononetin cultivars.
A rapid screening protocol to measure levels of the three isoflavones in subclover was developed in the early 1960s by Francis and Millington (1965). Subclover breeding programs have subsequently aimed to select genotypes with formononetin ≤0.2% of leaf dry matter (Nichols et al. 1996, 2013) and no cultivars have been released since 1982 with levels higher than this. Clover disease, previously prevalent across southern Australia, consequently diminished by the 1990s due to adoption of low-formononetin cultivars, as well as improved livestock management (Rossiter 1989; Adams 1995). However, Meat & Livestock Australia reported in 2002 that 10–15 million sheep in the Australian national flock were affected to some level by oestrogenic subclover (Walker et al. 2002) and this likely reflects the persistence of old cultivars of subclover with high levels of formononetin (Foster et al. 2021).
The content of formononetin in subclover pastures may be increased by abiotic stress factors such as waterlogging (Francis and Devitt 1969; Rossiter 1970a). In southern Australia, waterlogging, caused by rainfall exceeding drainage ability of the soil, extends over large areas of pasture during the growing season (Humphries 1962; Chapman et al. 2012). Such waterlogging may be transient, lasting several hours or days, or prolonged over several weeks (Malik et al. 2002). There is a need for further knowledge of the impact of waterlogging on formononetin in subclover pastures for two main reasons. First, subclover cultivars are screened in breeding programs for formononetin content under ideal growing conditions (irrigated, well drained soils) before release (Nichols et al. 2013) and their formononetin content under waterlogging is therefore unknown. Second, the high formononetin ssp. yanninicum cultivar Yarloop, commercialised in 1947 (Nichols et al. 2013), was historically the only commercially available cultivar for sites susceptible to waterlogging and is broadly established in high rainfall areas (Francis 1976a; Cocks et al. 1982; Nichols 2004); it likely remains common in these areas (Foster et al. 2021).
Waterlogging tolerance varies among subclover genotypes (Marshall and Millington 1967; Reed et al. 1985; Enkhbat et al. 2021a, 2022). However, the effect of waterlogging on leaf isoflavone content among subclover genotypes with contrasting tolerance to waterlogging has been little researched. The only study to examine this issue was conducted by Francis and Devitt (1969). In their glasshouse experiment, ssp. yanninicum exhibited higher tolerance of waterlogging, and had a lower increase in isoflavone content under waterlogging, than the two other subspecies. However, they only reported the average leaf isoflavone response to waterlogging for each subspecies (each represented by 25 genotypes) and did not examine the isoflavone response post-waterlogging. Recovery ability following waterlogging is considered essential to evaluate tolerance to waterlogging (Striker 2012).
Ecotypes of subclover have been collected from its native habitat in the Mediterranean region and surrounding areas and are a primary source of genetic diversity (Enkhbat et al. 2021b). These can be investigated to improve understanding of subclover adaptation, distribution and production potential (Hill 1996; Ghamkhar et al. 2007). Exploring a link between leaf isoflavone responses under waterlogging with existing genetic diversity among subclover genotypes could be beneficial to gain an understanding of simple selection criteria for a plant breeding program targeted to better animal reproductive performance in waterlogged pastures.
In view of the above, this study examines the relationship between leaf isoflavones and waterlogging tolerance in subclover cultivars and ecotypes with contrasting waterlogging tolerance levels identified by Enkhbat et al. (2021a) and Enkhbat et al. (2022). The aim was to determine if the impact of waterlogging on both individual leaf isoflavones (biochanin A, genistein, formononetin) and total isoflavone content is related to waterlogging tolerance. We tested three hypotheses in this study: (1) waterlogging will increase leaf isoflavones in subclover; (2) the magnitude of this impact will vary among subclover genotypes in relation to their degree of waterlogging tolerance and/or agronomic traits; and/or (3) genetic variability related to site of origin.
Materials and methods
This study presents leaf isoflavone response to waterlogging from two published controlled glasshouse experiments that examined waterlogging tolerance in subclover (Trifolium subterraneum L.) (Table 1). Experiment 1 examined Yarloop and eight other subclover cultivars across the three subspecies (Enkhbat et al. 2021a), while Experiment 2 examined four cultivars (Yarloop, Larisa, Meteora and Trikkala) and 12 wild ecotypes of ssp. yanninicum collected from the Mediterranean region (Enkhbat et al. 2022). Both experiments measured the maintenance of shoot relative growth rate (RGR, in mg g−1 day−1), the increase in plant mass per starting unit mass and time, relative to non-waterlogged conditions, which is the most common approach to define waterlogging tolerance (Setter and Waters 2003).
Experiment 1 | Experiment 2 | |
Experimental period | August–November 2019 | June–August 2020 |
Experiment duration | 12 weeks | 7 weeks |
Soil pH (CaCl2) | 5.8 | 6.2 |
Pot size | 4.5 L | 2.8 L |
Seed and seedling characters measured | – | 21 days after sowing (DAS) |
Nutrient reapplication dates | 28 and 49 DAS | 14 DAS |
Waterlogging (WL) treatment commencement/date for initial harvest | 28 DAS | 21 DAS |
WL treatment duration | 35 days | 28 days |
Leaf size and petiole length | – | 49 DAS |
Recovery phase following WL | Prolonged for 21 days under free-drained condition (maintained at 80% field capacity) | None |
Destructive harvest | Harvest I (63 DAS) at end of WL phase and Harvest II (84 DAS) at end of recovery phase | Final Harvest (49 DAS) at end of WL treatment |
In Experiment 1, leaf isoflavone response to both a waterlogging (WL) phase (Harvest I) and a recovery phase (Harvest II) and its relationship with shoot RGR, as an indicator of waterlogging tolerance, were explored. In Experiment 2, leaf isoflavone response to waterlogging and its relationship with key agro-morphological traits (e.g. mean seed weight, seedling shoot and root dry weight, leaf size, petiole length and days to first flowering) were compared at a single Final Harvest. These traits were selected because of their purported relationship with waterlogging tolerance and leaf isoflavone content observed earlier in Enkhbat et al. (2021b) and Enkhbat et al. (2022). The relationship of isoflavone response to waterlogging with eco-geographic variables at ecotype sites of origin were also examined.
Plant material
For Experiment 1, nine registered cultivars, representing each of the three subclover subspecies (subterraneum, brachycalycinum and yanninicum), were selected from the studies of Enkhbat et al. (2021a) (Table 2). This comprised Yarloop (high formononetin content) and eight other cultivars low in formononetin. Enkhbat et al. (2021a) observed high variation for waterlogging tolerance across these cultivars (means shown in Supplementary Table S1 and Fig. S1). Notably, the ssp. yanninicum cultivars, Meteora, Trikkala and Yarloop, and cultivars Denmark (ssp. subterraneum) and Clare (ssp. brachycalycinum) maintained shoot RGRs under waterlogging, relative to their controls (no treatment effect), and are hereafter referred to as ‘waterlogging-tolerant’. The other ssp. subterraneum and brachycalycinum cultivars were vulnerable to waterlogging (hereafter referred to as ‘waterlogging-susceptible’), with significantly lower shoot RGRs relative to the controls (free-draining): Mt. Barker (P ≤ 0.01), Seaton Park (P ≤ 0.05), Antas (P ≤ 0.001) and Rosedale (P ≤ 0.001). Shoot RGRs were recovered for all cultivars, irrespective of their performance under waterlogging (Table S1 and Fig. S1).
Cultivar | Origin | DFF | Tolerance to waterlogging | |
ssp. subterraneum | ||||
Mt. Barker | (MB) | Australia (naturalised) | 137 | Susceptible |
Denmark | (D) | Italy | 142 | Tolerant |
Seaton Park | (SP) | Australia (naturalised) | 110 | Susceptible |
ssp. brachycalycinum | ||||
Clare | (C) | Australia (naturalised) | 130 | Tolerant |
Antas | (A) | Italy | 138 | Susceptible |
Rosedale | (R) | Turkey | 114 | Susceptible |
ssp. yanninicum | ||||
Trikkala | (T) | Australia (crossbred) | 112 | Tolerant |
Yarloop | (Y) | Australia (naturalised) | 110 | Tolerant |
Meteora | (M) | Greece | 148 | Tolerant |
Country of origin and days to first flowering (DFF) from an early May sowing in Perth, Australia for the cultivars, as presented in Nichols et al. (2013).
For Experiment 2, the 16 ssp. yanninicum genotypes comprised cultivars Yarloop, Larisa, Meteora and Trikkala and 12 ecotypes (coded A–L) collected from the wild in the Mediterranean basin (Table 3). Each of these ecotypes had associated latitude, longitude, altitude and other collection site information, known as ‘passport data’. In this study, ‘genotype’ is a collective term for both ecotypes and cultivars. Cultivars Larisa and Meteora are themselves ecotypes, originally collected from Greece (Oram 1989; Nichols et al. 2013). The ecotypes were selected on the basis of diversity for: (1) passport data; and (2) days to first flowering (DFF), measured as the number of days from sowing to appearance of the first open flower, as measured by Enkhbat et al. (2021b) at Shenton Park, Western Australia (31°57′S, 115°5′E) (Table 3). Enkhbat et al. (2022) observed high variation (P ≤ 0.001) for shoot RGRs among genotypes of ssp. yanninicum, with no significant WL treatment effect (Table S2 and Fig. S2). All genotypes maintained shoot RGRs under waterlogging, relative to their controls.
Genotype | Ecotype code | Classification | DFF | Country of origin | Passport data | ||
Latitude | Longitude | Altitude | |||||
(°N) | (°E) | (m a.s.l.) | |||||
Yarloop | Cultivar/Naturalised | 101 | Australia | ||||
Larisa | Cultivar/Ecotype | 125 | Greece | 39.58 | 21.99 | 90 | |
Meteora | Cultivar/Ecotype | 149 | Greece | 39.58 | 21.96 | 100 | |
Trikkala | Cultivar/Cross-bred | 119 | Australia | ||||
CIZ001YANN-A | A | Ecotype | 110 | Turkey | 38.64 | 27.28 | 25 |
CIZ011YANN-B | B | Ecotype | 106 | Turkey | 38.65 | 27.29 | 20 |
CPI 039314YB | C | Ecotype | 118 | Greece | 39.49 | 22.14 | 92 |
CPI 103925A | D | Ecotype | 111 | Spain | 37.4 | −6.22 | 50 |
CPI 103933 | E | Ecotype | 132 | Spain | 37.63 | −6.65 | 360 |
DGG046-A | F | Ecotype | 115 | Greece | 39.67 | 19.78 | 75 |
DGG047-C | G | Ecotype | 108 | Greece | 39.67 | 19.78 | 75 |
DGG048-C | H | Ecotype | 111 | Greece | 39.66 | 19.75 | 130 |
EP042WHITE-C | I | Ecotype | 122 | Italy | 40.21 | 8.79 | 370 |
EP053WHITE-C | J | Ecotype | 128 | Italy | 40.29 | 8.78 | 660 |
EP055WHITE-C | K | Ecotype | 130 | Italy | 40.44 | 8.71 | 420 |
EP059WHITE-C | L | Ecotype | 137 | Italy | 40.46 | 8.73 | 425 |
Days to first flowering (DFF) is from Enkhbat et al. (2021b), recorded from a sowing date of 21 May 2019 at Shenton Park, Western Australia (31°57′S, 115°5′E). Country of origin and passport data were obtained from the Australian Pastures Genebank and the GRIN Global record website: https://apg.pir.sa.gov.au/grin. Units for altitude are metres above sea level (m a.s.l.).
Climatic variables
Nineteen bioclimatic (BIOCLIM) variables (coded as BIO1-BIO19) for collection sites of the 14 ecotypes (including cultivars Larisa and Meteora) in Experiment 2 were extracted from the ‘WorldClim’ (ver. 2) data base at 2.5 arc-min spatial resolution (Hijmans et al. 2005) (Table S3). These 19 bioclimatic variables are a standard set of eco-geographic variables that have been used in previous diversity studies of subclover (Ghamkhar et al. 2015; Abdi et al. 2020; Enkhbat et al. 2021b, 2022).
Plant growth and experimental design
Details of plant growth, experimental design and harvests for both Experiments 1 and 2 are presented in Enkhbat et al. (2021a) and Enkhbat et al. (2022), respectively. Common conditions for both experiments are described in the following paragraphs and details of each experiment summarised in Table 1.
Both experiments were conducted in a naturally-lit glasshouse set at 20°C/15°C day/night at The University of Western Australia (31°98′S, 115°50′E). Plant were grown in sterilised, free-draining plastic pots with weed mat (Gale Pacific Limited) placed in the base of the pots to prevent soil loss. A mix of washed river sand and Gingin loam (1:1) was used in both experiments. Soil was steam pasteurised and dried at 40°C. Soil analyses were conducted at CSBP laboratories (Bibra Lake, Western Australia) (Table S4). Two days prior to transplanting seeds in both experiments, pots were watered to 60% of field capacity (FC) and all essential nutrients were applied (mg kg−1 soil−1): P 20.5; K 88.7; S 34.2; Ca 41.0; Cl 72.5; Mg 3.95; Mn 3.26; Zn 2.05; Cu 0.51; B 0.12; Co 0.11 and Mo 0.08.
In both experiments, seed coats were first scarified with a seed scarifier (Kimseed Australia Pty Ltd) and placed in Petri dishes (referred here as the day of sowing) containing double layers of filter paper (Whatman No. 1), moistened by 3.5 mL of deionised (DI) water for 36–39 h in a darkened temperature-controlled room at 15°C. Six seeds with newly-emerged radicles were transplanted into each pot (radicle placed downwards). All pots were then watered to 80% FC with 40 mL of Rhizobium leguminosarum bv. trifolii strain WSM1325 (Group C) inoculum (mixture of 2.8 g rhizobia in 1000 mL of water). Plants were later randomly thinned to three per pot in both experiments.
Both experiments had factorial randomised block design with two factors (treatment and genotype) comprising two treatments in four replicates. Each pot was an experimental unit. The two treatments in both experiments consisted of: (1) a free-draining (control) treatment, which was watered to 80% of FC for the duration of the experiment; and (2) a waterlogged (WL) treatment in which the water level was maintained 10 mm above the soil surface. In Experiment 1, a recovery phase followed after 35 days of waterlogging where waterlogged pots were allowed to drain and plants were grown for another 21 days under free-draining conditions, with watering to 80% FC.
Measurements
At every harvest (Table 1), biochanin A (BA), genistein (G) and formononetin (F) were measured using the technique of Francis and Millington (1965). Briefly, two healthy, young and fully opened leaves were folded and clipped with a disc punch, resulting in six leaf discs (6 mm diameter), and placed in test-tubes. Leaf discs were immediately crushed by a glass rod with a few grains of acid-washed sand, hydrolysed for 15 min and then extracted in 1 mL ethanol by shaking in a water bath at 60°C for 15 min. Using a capillary tube, 5 μL aliquot from each sample were spotted onto aluminium sheets (20 cm × 20 cm) coated with TLC silica gel (fluorescent indicator F254) and extracts were then subjected to thin-layer chromatography with 1 cm solvent depth (90 mL chloroform to 10 mL methanol). Individual isoflavones for each sample were assessed by a semi-quantitative method, which rated the intensity of sample spots against those from standards of known concentration under UV light at 254 nm. Duplicate clipped leaf samples were also taken and oven dried at 60°C for 48 h for dry weight (DW) measurement. Isoflavone content was then calculated as a percentage of DW. In Experiment 1, isoflavone content was analysed from the third trifoliate leaf at 63 days after sowing (DAS), corresponding to 35 days of treatment, and at 84 DAS (after 21 days of recovery phase). In Experiment 2, leaf isoflavone content was analysed in the fourth trifoliate leaf at 49 DAS (after 28 days of treatment).
In both experiments, initial DW was measured on the day of treatment commencement. Shoots and roots were oven-dried at 60°C for 4 days and weighed. RGRs were calculated using the standard equation of Hunt (1982): ; where DW2 = dry weight (g) at time 2, = mean of dry weight (g) at time 1, t = treatment time and t2 – t1 = number of days between sampling times (35 and 28 days for the WL phase in Experiment 1 (Harvest I) and in Experiment 2 (Final Harvest), respectively; and 21 days for the recovery phase in Experiment 1 (Harvest II)).
Details of measurement techniques for other plant traits are provided in Enkhbat et al. (2021a) for Experiment 1 and Enkhbat et al. (2022) for Experiment 2. A brief summary is as follows:
Root RGR was estimated by the same method for the same time periods used to calculate shoot RGR (see above).
Four replicates of 50 random seeds per genotype were counted using a Condator seed counter (Preuffer, Germany) and weighed to determine mean seed weight.
At 21 DAS, prior to the start of the WL phase, shoot and root DWs were measured under free-drained conditions for seedling removed when seedling numbers per pot where thinned to three. Shoots and roots were oven-dried at 60°C for 72 h and weighed.
At 49 DAS, during the WL phase, leaf size (cm2) was measured on every fourth trifoliate of all plants in each pot by comparing each leaf with photographic leaf size standards of subclover by Williams et al. (1964) and using their conversion formulae. Petiole length (cm) of the same leaves was measured using a ruler.
Data for DFF for the 16 genotypes of ssp. yanninicum are from Enkhbat et al. (2021b), as recorded in the field at Shenton Park, Western Australia.
Statistical analyses
Data were graphed using SigmaPlot 14 (Systat Software, Inc.). Data for both experiments were analysed using two-way ANOVA in R software (ver. 3.6.3), with genotype and treatment as main factors. The ANOVA assumptions were checked using the ‘reset’ test to determine whether the fitted model was plausible. The assumptions of equal group variance and the normality of residual distribution were tested by Bartlett and Shapiro–Wilk tests, respectively. An implemented robust ANOVA test with a robust covariance matrix, was conducted when heteroskedasticity was a problem. The dependent variable (leaf isoflavones) was log-transformed (log[x + 1]), when the error was not normally distributed. As the experiments were factorial designs in randomised blocks with four replicates, a two-factor model with a nested structure (randomised genotypes and replicates) for errors, was used by the ‘lme’ function in the ‘nlme’ package in R.
Tukey’s test was used to compare differences among means when interactions were significant. Least significant difference (l.s.d.) Fisher’s protected tests were used to compare means of shoot RGR in Experiment 1. Broad-sense heritability (H2) was also estimated for leaf isoflavones among genotypes in both experiments according to Falconer and Mackay (1996). Pearson correlation coefficients and their levels of significance were calculated between: (1) control and waterlogging/recovery treatments for total and individual isoflavones in both experiments; and (2) isoflavone contents under waterlogging with eco-geographic variables of collection sites in Experiment 2. Cultivars of ssp. yanninicum were excluded from the analysis of Experiment 2 to remove bias, as the more recent cultivars have undergone deliberate selection for low F levels.
Results
Experiment 1 (nine subclover cultivars from three subspecies)
Following the WL phase at Harvest I, total isoflavone content (i.e. the sum of BA, G and F,) was impacted by treatment and cultivar (both P ≤ 0.001), with estimated treatment means being 1.25% DW for the control and 2.22% DW for WL (Table S5). A large impact of cultivar was driven by low values for Rosedale, the only cultivar that flowered during the experiment. There was a strong positive relationship between control and WL (r = 0.78; P ≤ 0.001). Broad-sense heritability (H2) was 65.8% for the control and 33.8% for the WL. After the recovery phase at Harvest II, there was an impact of cultivar only (P ≤ 0.001), reflecting very low values for Rosedale (Table S5). Overall, WL considerably increased total isoflavone content relative to the control, but by the end of recovery phase it did not differ from the respective controls.
For the content of each individual isoflavone after the WL phase at Harvest I there was a weak significant treatment × cultivar interaction (P ≤ 0.05) only for F (Fig. 1; Table S5). Thus, the impact of WL generally did not differ among cultivars, with estimated means increasing from 0.32% DW (control) to 0.60% DW (WL) for BA, from 0.74% DW to 1.31% DW for G, and from 0.19% DW to 0.31% DW for F. Cultivar had a large impact on all isoflavones, reflecting distinctive differences in isoflavone profile among them. For instance, cultivars of ssp. brachycalycinum had high G and low or absent F under both control and WL treatments, F was absent in Denmark and very high for Yarloop under both treatments, and total isoflavone contents for Rosedale were low under both treatments. Notably, all subclover cultivars under control conditions had a mean F below 0.3% DW (the ‘safe’ level), apart from Yarloop (1.5% DW) (Fig. 1; Table S5). However, after the WL phase, F increased to above 0.3% DW in the ssp. yanninicum cultivars, Trikkala (0.39% DW) and Meteora (0.28%) (Fig. 1; Table S5). Among subclover cultivars, there were strong positive relationships (all P ≤ 0.001) between control and WL for BA (r = 0.91), G (r = 0.86) and for F (r = 0.94). H2 was high under both control and WL for BA (82.3% and 88.9%, respectively) and F (93.5% and 95.1%), but low for G (64.6% and 49.3%). After the recovery phase at Harvest II, there was an impact of cultivar only (P ≤ 0.001) for each individual isoflavone content, reflecting very low values for Rosedale (Table S5). It was notable that the content of each individual isoflavone was higher in the controls after the recovery phase than 21 days earlier, except for Mt. Barker, Rosedale, Yarloop and Meteora.
Leaf isoflavones, as a % of dry weight (DW), comprised of biochanin A (BA; yellow bars), genistein (G; orange bars), and formononetin (F; red bars), for nine cultivars from the three subspecies of Trifolium subterraneum following the waterlogging phase (WLP) (Harvest I) and the recovery phase (RP) (Harvest II) in Experiment 1. Treatments in the WLP, imposed at 28 days after sowing and prolonged for 35 days, were free-draining (control; plain bars) and waterlogged (WL; hatched bars; water level kept 10 mm above the soil surface). In the following RP, remaining control pots were maintained free-draining (control; plain bars) and WL pots were allowed to free-drain for 21 days (dotted bars: WL-R). Values for bars are total isoflavone content (means + s.e., n = 4). For each of the WLP and RP, results of two-way ANOVA are presented with significant effects for cultivar (C), treatment (T) or their interaction (C × T): *P ≤ 0.05; **P ≤ 0.01; ***P ≤ 0.001. A significant treatment difference between control and WL at **P < 0.01 is shown (Tukey’s test) when the interaction was significant. The horizontal blue line represents a formononetin ‘safe’ level for sheep reproduction of 0.3% DW.
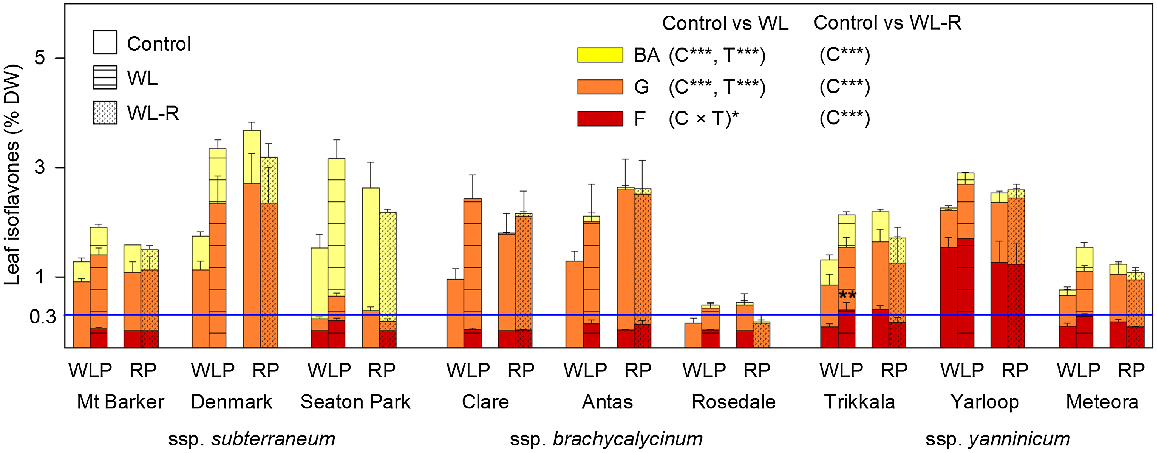
In summary, after the WL phase (Harvest I), total and individual isoflavone contents considerably increased in a similar manner for all cultivars, but only cultivars of ssp. yanninicum had F beyond 0.3% DW. After the recovery phase (Harvest II), both total and individual isoflavone contents did not significantly differ from their respective controls. This was, however, associated with increased isoflavone contents of the controls; indeed isoflavone contents had minor declines or remained unchanged (Fig. 1; Table S5).
After the WL phase at Harvest I, the relative proportions of the three isoflavones was strongly impacted by cultivar (all P ≤ 0.001), with a weaker treatment × cultivar interaction (all P ≤ 0.05), which reflected the impact of WL differing among cultivars (Table 4). Post hoc Tukey’s tests showed only a single instance where the proportion of an isoflavone differed between the control and WL, which was for Rosedale (P ≤ 0.01) where the G proportion decreased from 100% (control) to 87.6% (waterlogging) (Table 4). Among subclover cultivars, there were strong positive relationships (all P ≤ 0.001) between control and WL for the proportions of BA (r = 0.91), G (r = 0.73) and F (r = 0.93).
Cultivar | BA | G | F | |||
Control | WL | Control | WL | Control | WL | |
ssp. subterraneum | ||||||
Mt. Barker | 27.9 | 26.3 | 72.1 | 71.2 | 0.0 | 2.6 |
Denmark | 36.5 | 30.8 | 63.5 | 69.2 | 0.0 | 0.0 |
Seaton Park | 85.0 | 79.1 | 14.4 | 14.7 | 0.5 | 6.2 |
ssp. brachycalycinum | ||||||
Clare | 0.0 | 0.0 | 100 | 98.5 | 0.0 | 1.5 |
Antas | 0.0 | 2.9 | 100 | 92.1 | 0.0 | 5.0 |
Rosedale | 0.0 | 7.7 | 100 | 87.6** | 0.0 | 4.7 |
ssp. yanninicum | ||||||
Trikkala | 38.4 | 29.3 | 57.3 | 53.9 | 4.3 | 16.8 |
Yarloop | 1.9 | 6.9 | 30.7 | 33.5 | 67.4 | 59.5 |
Meteora | 10.7 | 28.4 | 77.8 | 53.8 | 11.5 | 17.8 |
P-value | ||||||
Cultivar (C) | ≤0.001 | ≤0.001 | ≤0.001 | |||
Treatment (T) | n.s. | ≤0.05 | ≤0.01 | |||
C × T | ≤0.05 | ≤0.05 | ≤0.05 |
Treatments, imposed at 28 days after sowing and prolonged for 35 days, were: free-draining (control) and waterlogged (WL; water level kept 10 mm above the soil surface).
The results of a two-way ANOVA are presented. n.s., not significant. Data in bold indicates the significant differences (Post hoc Tukey’s test) between control and WL: **P ≤ 0.01.
Experiment 2 (four cultivars and 12 ecotypes of ssp. yanninicum)
After the WL phase at the Final Harvest, there was a significant treatment × genotype interaction for total isoflavone content (P ≤ 0.001) among genotypes of ssp. yanninicum (Table S6), indicating that the effect of WL differed among genotypes. WL significantly increased (0.05 ≤ P ≤ 0.001) total isoflavone content in Larisa and Trikkala and five of the 12 ecotypes (Table S6). There was a strong positive relationship between control and WL (r = 0.62; P ≤ 0.001). H2 was 89.9% for the control and 73.5% for the WL. Overall, total isoflavone content increased under WL, but the extent of this varied among genotypes.
After the WL phase at the Final Harvest, for the content of each individual isoflavone, there were significant treatment × genotype interactions (all P ≤ 0.001) (Fig. 2; Table S6). Significant increases under WL included: (1) BA for cultivars Larisa and Trikkala and Ecotypes A, B, C, E, G and H; (2) G for cultivars Larisa and Trikkala and Ecotype C; and (3) F for Ecotypes A, B, D, G and H. Notably, only cultivars Larisa, Meteora and Trikkala and three ecotypes (C, E and L) had a mean F below 0.3% of DW under control conditions (Fig. 2; Table S6). However, after the WL treatment, F increased to greater than 0.3% DW in Meteora and Ecotype E. Among genotypes, there were strong positive relationships (all P ≤ 0.001) between control and WL for BA (r = 0.92), G (r = 0.71) and F (r = 0.83). H2 was under both control and WL for BA (91.1% and 95.1%, respectively), for F (91.7% and 94.9%) and for G (92.7% and 76.9%). Overall, individual isoflavone contents were increased under WL with genotype differences; and only cultivars Larisa and Trikkala and Ecotypes C and L satisfied the F ‘safe’ level 0.3% DW under WL.
Leaf isoflavones, as a % of dry weight (DW), comprised of biochanin A (BA; yellow bars), genistein (G; orange bars) and formononetin (F; red bars), for 16 genotypes (four cultivars and 12 ecotypes) of Trifolium subterraneum L. ssp. yanninicum following the waterlogging (WL) phase at Final Harvest in Experiment 2. Treatments, imposed at 21 days after sowing and prolonged for 28 days, were: free-draining (control; plain bars) and WL, with water level kept 10 mm above the soil surface (hatched bars). Values for bars are total isoflavone content (means + s.e., n = 4). The results of a two-way ANOVA are presented with significant effects for the interaction (G × T) of genotype (G) and treatment (T) at ***P ≤ 0.001. The significant differences between the control and WL treatments of each genotype are shown: *P ≤ 0.05; **P ≤ 0.01; ***P ≤ 0.001 (Tukey’s test). The horizontal blue line represents a formononetin ‘safe’ level of 0.3% DW for sheep reproduction. See Table 3 for ecotype codes.
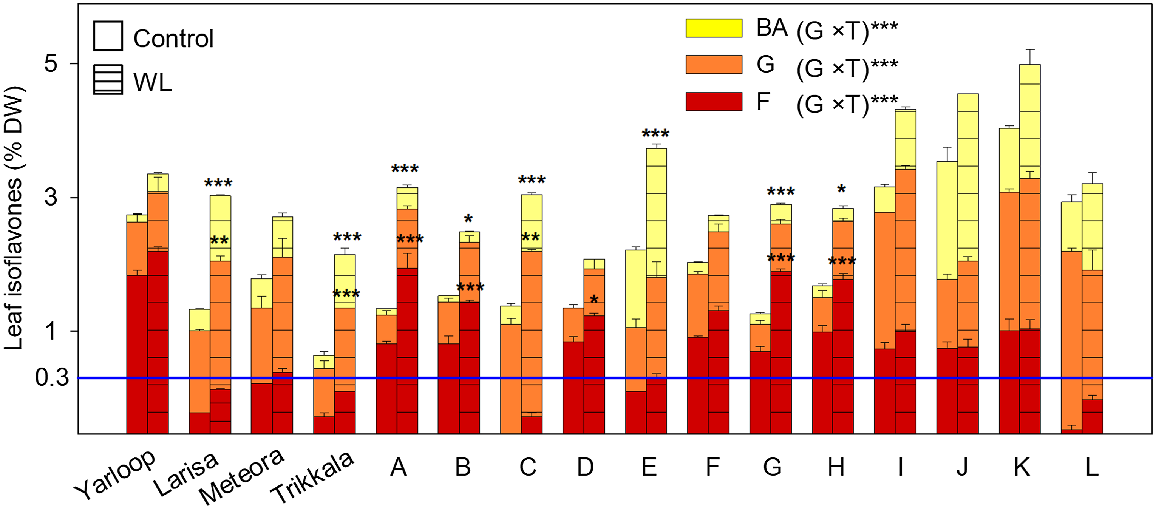
The relative proportion of the three isoflavones after the WL phase at the Final Harvest was impacted by a treatment × genotype interaction for BA, by both treatment and genotype for G, and by genotype only for F (all P ≤ 0.001) (Table 5). For BA, the interaction was due to significant increases in the proportion of BA under WL for three genotypes: Yarloop (P ≤ 0.01), Ecotype D (P ≤ 0.001) and Ecotype L (P ≤ 0.05) (Table 5). WL significantly decreased the proportion of G, where estimated treatment means were 49% (control) and 44% (WL). High variation was present for proportion of G among genotypes (P ≤ 0.001), with considerably lower estimated means for Yarloop and Ecotype G (27 and 28%, respectively) compared to Larisa and Ecotype C (68% and 75%, respectively). Highly significant variation for the proportion of F was evident (P ≤ 0.001), with the lowest estimated mean in Ecotype C (0.6%) and the highest in Yarloop (66%). Among genotypes, there were strong positive relationships (all P ≤ 0.001) between control and waterlogging for the proportion of BA (r = 0.88), G (r = 0.88) and F (r = 0.97).
Genotypes of spp. yanninicum | BA | G | F | |||
Control | WL | Control | WL | Control | WL | |
Yarloop | 4.1 | 8.3** | 28.9 | 25.3 | 67.0 | 66.5 |
Larisa | 23.6 | 32.3 | 72.7 | 63.6 | 3.8 | 4.1 |
Meteora | 24.7 | 22.3 | 62.9 | 62.9 | 12.5 | 14.8 |
Trikkala | 35.3 | 36.9 | 59.8 | 58.4 | 4.9 | 4.7 |
A | 7.6 | 10.3 | 31.2 | 28.8 | 61.2 | 60.9 |
B | 6.9 | 6.5 | 40.6 | 35.8 | 52.5 | 57.6 |
C | 20.0 | 28.0 | 80.0 | 70.8 | 0.0 | 1.2 |
D | 0.0 | 7.4*** | 38.1 | 32.7 | 61.9 | 59.9 |
E | 52.3 | 52.6 | 43.1 | 39.1 | 4.6 | 8.2 |
F | 8.6 | 8.7 | 46.3 | 43.0 | 45.1 | 48.3 |
G | 12.9 | 10.3 | 31.3 | 24.4 | 55.8 | 65.2 |
H | 10.4 | 7.0 | 29.9 | 30.4 | 59.7 | 62.5 |
I | 12.3 | 28.5 | 64.9 | 48.5 | 22.7 | 22.9 |
J | 49.6 | 51.5 | 29.5 | 32.3 | 20.9 | 16.2 |
K | 24.0 | 34.6 | 51.3 | 49.6 | 24.7 | 15.8 |
L | 24.4 | 41.3* | 75.2 | 56.5 | 0.4 | 2.2 |
P-value | ||||||
Genotype (G) | ≤0.001 | ≤0.001 | ≤0.001 | |||
Treatment (T) | ≤0.001 | ≤0.001 | n.s. | |||
G × T | ≤0.001 | n.s. | n.s. |
Treatments, imposed at 21 days after sowing and prolonged for 28 days, were: free-draining (control) and waterlogged (WL, water level kept 10 mm above the soil surface).
The results of a two-way ANOVA are presented. n.s., not significant. Data in bold indicates the significant differences (Post hoc Tukey’s test) between control and WL: *P ≤ 0.05; **P ≤ 0.01; ***P ≤ 0.001.
High variation (P ≤ 0.001) for shoot RGRs existed among genotypes of ssp. yanninicum, with no significant WL treatment effect (Table S2 and Fig. S2). All genotypes maintained shoot RGRs under waterlogging, relative to their controls. The relationships between isoflavone contents under WL with shoot RGR and agronomic traits among 12 ecotypes of ssp. yanninicum (Tables S2 and S7 and summarised means in Fig. S3) were analysed. Under WL, total and individual isoflavone contents were unrelated to either waterlogging tolerance (as determined by shoot RGR) or agronomic traits measured at 21 and 49 DAS (Table S8). Only, a weak positive correlation (r = 0.50; P ≤ 0.05) was found between G (WL) and leaf size at 49 DAS. DFF was positively correlated with total isoflavone (r = 0.67; P ≤ 0.05), BA (r = 0.85; P ≤ 0.001) and G (r = 0.67; P ≤ 0.05) contents under WL, but negatively correlated with F (r = −0.77; P ≤ 0.05) (Table S8).
Correlations of isoflavone contents for plants in the WL treatment after the WL phase at the Final Harvest with eco-geographic variables from their sites of origin (Table S3) were analysed for Larisa and Meteora and Ecotypes A-L (Table 6). BA under WL had a strong positive correlation with altitude, while neither G nor F were significantly correlated with any geographic variables (Table 6). Temperature variables were unrelated to individual isoflavone contents under WL, except for a weak negative correlation of BA with mean summer temperature. However, total isoflavone content in the WL treatment tended to be low in genotypes that originated from sites with hot summers (Table 6). Precipitation variables showed a relationship with both G and F under WL, but not with BA. Genotypes that originated from sites with high summer precipitation also had a general trend of increased G under WL, whereas genotypes from sites with high winter precipitation tended to have increased F under WL.
Eco-geographic variables | Code | Leaf isoflavone content (% dry weight) | |||
Total | BA | G | F | ||
Latitude (°N) | n.s. | n.s. | n.s. | n.s. | |
Longitude (°E) | n.s. | n.s. | n.s. | n.s. | |
Altitude (m) | 0.83*** | 0.90*** | n.s. | n.s. | |
Annual mean temperature (°C) | BIO1 | −0.67** | n.s. | n.s. | n.s. |
Mean diurnal range (°C) | BIO2 | −0.61* | n.s. | n.s. | n.s. |
Isothermality | BIO3 | −0.68** | n.s. | −0.70** | n.s. |
Temperature seasonality | BIO4 | n.s. | n.s. | n.s. | n.s. |
Maximum temperature of the warmest month (°C) | BIO5 | n.s. | n.s. | n.s. | n.s. |
Minimum temperature of the coldest month (°C) | BIO6 | n.s. | n.s. | n.s. | n.s. |
Temperature annual range (°C) | BIO7 | n.s. | n.s. | n.s. | n.s. |
Mean temperature of the wettest quarter (°C) | BIO8 | n.s. | n.s. | n.s. | n.s. |
Mean temperature of the driest quarter (°C) | BIO9 | −0.77** | −0.53* | n.s. | n.s. |
Mean temperature of the warmest quarter (°C) | BIO10 | −0.73** | n.s. | n.s. | n.s. |
Mean temperature of the coldest quarter (°C) | BIO11 | n.s. | n.s. | n.s. | n.s. |
Annual precipitation (mm) | BIO12 | n.s. | n.s. | n.s. | 0.59* |
Precipitation of the wettest month (mm) | BIO13 | n.s. | n.s. | n.s. | 0.74** |
Precipitation of the driest month (mm) | BIO14 | n.s. | n.s. | n.s. | n.s. |
Precipitation seasonality | BIO15 | n.s. | n.s. | −0.70** | 0.81*** |
Precipitation of the wettest quarter (mm) | BIO16 | n.s. | n.s. | n.s. | 0.74** |
Precipitation of the driest quarter (mm) | BIO17 | n.s. | n.s. | 0.56* | n.s. |
Precipitation of the warmest quarter (mm) | BIO18 | n.s. | n.s. | 0.70** | n.s. |
Precipitation of the coldest quarter (mm) | BIO19 | n.s. | n.s. | n.s. | 0.77** |
The WL treatment was imposed after 21 days after sowing and prolonged for 28 days, water level kept 10 mm above the soil surface.
n.s., not significant.
BOCLIM variables for each genotype are supplied in Table S2. *P ≤ 0.05; **P ≤ 0.01; ***P ≤ 0.001.
Discussion
Subclover leaf isoflavones increased in response to waterlogging, supporting the first hypothesis of this study, but the relative proportions of the three individual isoflavones were maintained compared to free-draining control conditions. The ratio of the three isoflavones for individual genotypes also remained similar among a large number of samples collected at different sites (Beck 1964). The high heritability of individual isoflavones under both free-draining and waterlogged conditions may explain the relatively stable isoflavone proportions under different environmental conditions.
The increases of leaf isoflavone contents under waterlogging are likely to be accompanied by subclover growth reductions. Francis and Devitt (1969) and Herms and Mattson (1992) suggest that growth reduction under waterlogging contributes to the increase in leaf isoflavones and to the magnitude of F increase in particular. Biosynthetic pathways for both proteins and isoflavone synthesis compete for the same precursor – the amino acid phenylalanine as a primary substrate (Margna et al. 1989; Jones and Hartley 1999). Waterlogging may induce an energy deficiency leading to a limitation in protein formation and declining growth rates; consequently, the surplus of phenylalanine increases secondary metabolites, resulting in an increase in isoflavone production (Harborne 1967; Rossiter 1970b, 1972; Herms and Mattson 1992). Thus, the second hypothesis is partially supported. But, it is worth noting that neither the amount nor proportion of isoflavones, particularly F, under waterlogging were related to shoot biomass production (e.g. shoot RGR) of subclover. Both high and low F contents under waterlogging were present among genotypes that exhibited superior waterlogging tolerance, whereas lower F content under waterlogging was found in genotypes vulnerable to waterlogging, particularly cultivars of ssp. brachycalycinum. Hence, high F content under waterlogging was a consequence of inherently high F of that particular genotype.
The commercially released ssp. yanninicum cultivars Meteora and Trikkala, with high waterlogging tolerance, had F contents above 0.3% DW under waterlogged conditions. Meteora is itself an ecotype, originally collected from Greece (Oram 1989). It was selected for release in 1981 as an interim cultivar, primarily because it had high resistance to clover scorch disease (Kabatiella cualivora), which was causing major problems in high rainfall areas in the 1970s (Nichols et al. 2014) in spite of it having a higher F content than 0.2% DW (Oram 1989; Nichols et al. 2013). Although it has been little sown as a cultivar, it has been widely used as a parent in crossing programs as a source of clover scorch resistance (Nichols et al. 2014). This has resulted in cultivars Riverina, Gosse, Monti, Napier, Rouse and Yanco, each of which has undergone selection for F contents ≤0.2% DW.
Cultivar Trikkala is derived from cross-breeding between Larisa and Neuchatel, an earlier flowering variant of Yarloop (Nichols et al. 2013). It has been widely sown in high rainfall areas after being first released in 1975 to replace the high-F cultivar, Yarloop (Francis 1976b). However, the magnitude of F increase in Trikkala under waterlogging, found in Experiment 1, indicates that further studies are needed to investigate the effects of waterlogging on livestock reproduction, as Trikkala is a widely sown cultivar and transient waterlogging is a common abiotic stress in southern Australia (Hamilton et al. 2000; Malik et al. 2002).
Leaf isoflavones increased considerably among ssp. yanninicum genotypes with waterlogging, even when shoot RGR was maintained under waterlogging. This increase could be associated with a reduction in root growth for ssp. yanninicum genotypes, since soil waterlogging has a direct negative effect on the root system (Colmer and Voesenek 2009). Reduced root growth is a common behaviour for ssp. yanninicum under waterlogging, as the plant maintains shoot growth through maintenance, or even enlargement, of leaves and elongation of petioles, enabling internal aeration to conduct oxygen from the atmosphere to the roots (Herzog and Pedersen 2014; Enkhbat et al. 2021a, 2022). Hence, maintenance of high aboveground biomass production under waterlogging, a common trait for determining waterlogging stress tolerance (Striker et al. 2008; Nakai et al. 2009), cannot be used as a proxy for leaf isoflavone response. However, relationship between leaf isoflavone response to waterlogging with other adaptive traits that contribute waterlogging tolerance to subclover such as aerenchyma formation and root porosity, chlorophyll content and leaf gas exchange parameters could be considered in future studies.
All three isoflavones showed a slightly decreasing trend after waterlogging ceased, that is, during the recovery phase (except G for Antas). In general, F tended to decrease during the recovery phase: this could be associated with improved growth rate (both shoot and root) as soils become aerated (Herms and Mattson 1992). However, leaf isoflavones in some waterlogging-susceptible cultivars (e.g. Clare and Antas) did not reduce as much during the recovery phase. This is of concern as leaf isoflavones, particularly F, which increased beyond the safe level during waterlogging could remain at high levels, even after water recedes.
Conclusions
This study has identified a leaf isoflavone response to waterlogging in subclover, a globally important annual pasture legume. Leaf isoflavones varied among subclover genotypes and increased with waterlogging, but the proportion of individual isoflavones was stable. Genotypes of subclover with inherently high F content are unsafe for grazing irrespective of their waterlogging tolerance. This new knowledge can be applied to support animal welfare and pasture management under waterlogging. Winter waterlogging can interact with other stresses, such as nutrient deficiencies, to exacerbate leaf F increases. It is possible that the impact of oestrogenic pastures on livestock fertility might be worse than expected at waterlogged sites. Field studies under waterlogged conditions are required to validate our results.
Data availability
The data that support this study are available in the article and accompanying online supplementary material.
Conflicts of interest
Megan Ryan is an Associate Editor of Crop & Pasture Science, but was blinded from the peer-review process for this paper. All other authors declare no conflicts of interest.
Declaration of funding
This research was conducted as part of the Annual Legume Breeding Australia (ALBA) Joint Venture between UWA and DLF Seeds. The Department of Primary Industry and Regional Development, Western Australia, is acknowledged for its role in the successful establishment and funding of ALBA. G.E. was supported by an Australian Government Research Training Program (RTP) scholarship and a Science Industry PhD Fellowship from the Government of Western Australia. KF was supported by the Meat & Livestock Australia Donor Company project P.PSH.1171 and P.PSH.1138.
Acknowledgements
We gratefully acknowledge Rosemarie Lugg, Ann Hamblin, Bradley Wintle, Robert Creasy, Bill Piasini, Elise Robinson and Robert Jeffery for technical advice and help.
References
Abdi AI, Nichols PGH, Kaur P, Wintle BJ, Erskine W (2020) Morphological diversity within a core collection of subterranean clover (Trifolium subterraneum L.): lessons in pasture adaptation from the wild. PLoS ONE 15, e0223699| Morphological diversity within a core collection of subterranean clover (Trifolium subterraneum L.): lessons in pasture adaptation from the wild.Crossref | GoogleScholarGoogle Scholar |
Adams NR (1990) Permanent infertility in ewes exposed to plant oestrogens. Australian Veterinary Journal 67, 197–201.
| Permanent infertility in ewes exposed to plant oestrogens.Crossref | GoogleScholarGoogle Scholar |
Adams NR (1995) Detection of the effects of phytoestrogens on sheep and cattle. Journal of Animal Science 73, 1509–1515.
| Detection of the effects of phytoestrogens on sheep and cattle.Crossref | GoogleScholarGoogle Scholar |
Adams NR (1998) Clover phytoestrogens in sheep in Western Australia. Pure and Applied Chemistry 70, 1855–1862.
| Clover phytoestrogens in sheep in Western Australia.Crossref | GoogleScholarGoogle Scholar |
Adams NR, Sanders MR, Ritar AJ (1988) Oestrogenic damage and reduced fertility in ewe flocks in south Western Australia. Australian Journal of Agricultural Research 39, 71–77.
| Oestrogenic damage and reduced fertility in ewe flocks in south Western Australia.Crossref | GoogleScholarGoogle Scholar |
Beck AB (1964) The oestrogenic isoflavones of subterranean clover. Australian Journal of Agricultural Research 15, 223–230.
| The oestrogenic isoflavones of subterranean clover.Crossref | GoogleScholarGoogle Scholar |
Beck AB, Gardiner MR (1965) Clover disease of sheep in Western Australia. Journal of the Department of Agriculture, Western Australia 6, 388–400.
Benassayag C, Perrot-Applanat M, Ferre F (2002) Phytoestrogens as modulators of steroid action in target cells. Journal of Chromatography B 777, 233–248.
| Phytoestrogens as modulators of steroid action in target cells.Crossref | GoogleScholarGoogle Scholar |
Bennetts HW, Uuderwood EJ, Shier FL (1946) A specific breeding problem of sheep on subterranean clover pastures in Western Australia. Australian Veterinary Journal 22, 2–12.
| A specific breeding problem of sheep on subterranean clover pastures in Western Australia.Crossref | GoogleScholarGoogle Scholar |
Braden AWH, Hart NK, Lamberton JA (1967) Oestrogenic activity and metabolism of certain isoflavones in sheep. Australian Journal of Agricultural Research 18, 355–348.
| Oestrogenic activity and metabolism of certain isoflavones in sheep.Crossref | GoogleScholarGoogle Scholar |
Brightling A (2006) ‘Livestock diseases in Australia: diseases of cattle, sheep, goats and farm dogs.’ (CH Jerram & Associates: Mount Waverley, Vic., Australia)
Chapman SC, Chakraborty S, Dreccer MF, Howden SM (2012) Plant adaptation to climate change-opportunities and priorities in breeding. Crop & Pasture Science 63, 251–268.
| Plant adaptation to climate change-opportunities and priorities in breeding.Crossref | GoogleScholarGoogle Scholar |
Cocks PS, Craig AD, Kenyon RV (1982) Evolution of subterranean clover in South Australia. II. Change in genetic composition of a mixed population after 19 years’ grazing on a commercial farm. Australian Journal of Agricultural Research 33, 679–695.
| Evolution of subterranean clover in South Australia. II. Change in genetic composition of a mixed population after 19 years’ grazing on a commercial farm.Crossref | GoogleScholarGoogle Scholar |
Collins WJ, Cox RI (1984) Oestrogenic activity in forage legumes. In ‘Forage legumes for energy efficient animal production. Proceedings of a trilateral workshop, Palmerston North, New Zealand’. (Eds RF Barnes, PR Ball, RW Brougham, GC Mearten, DJ Minson) pp. 268–276. (USDA-ARS: Springfield, VA, USA)
Colmer TD, Voesenek LACJ (2009) Flooding tolerance: suites of plant traits in variable environments. Functional Plant Biology 36, 665–681.
| Flooding tolerance: suites of plant traits in variable environments.Crossref | GoogleScholarGoogle Scholar |
Cox RI, Braden AWH (1974) The metabolism and physiological effects of phyto-oestrogens in livestock. Proceedings of the Australian Society of Animal Production 10, 122–129.
Davies LH (1986) Limitations to livestock production associated with phytoestrogens and bloat. In ‘Temperate pastures: their production, use and management’. (Eds JL Wheeler, CJ Pearson, GE Robards) pp. 446–456. (Australian Wool Corporation/CSIRO Australia: Melbourne, Vic., Australia)
Enkhbat G, Ryan MH, Foster KJ, Nichols PGH, Kotula L, Hamblin A, Inukai Y, Erskine W (2021a) Large variation in waterlogging tolerance and recovery among the three subspecies of Trifolium subterranean L. is related to root and shoot responses. Plant and Soil 464, 467–487.
| Large variation in waterlogging tolerance and recovery among the three subspecies of Trifolium subterranean L. is related to root and shoot responses.Crossref | GoogleScholarGoogle Scholar |
Enkhbat G, Nichols PGH, Foster KJ, Ryan MH, Inukai Y, Erskine W (2021b) Diversity for morphological traits, flowering time and leaf isoflavone content among ecotypes of Trifolium subterraneum L. ssp. yanninicum and their relationships with site of origin. Crop & Pasture Science 72, 1022–1033.
| Diversity for morphological traits, flowering time and leaf isoflavone content among ecotypes of Trifolium subterraneum L. ssp. yanninicum and their relationships with site of origin.Crossref | GoogleScholarGoogle Scholar |
Enkhbat G, Ryan MH, Nichols PGH, Foster KJ, Inukai Y, Erskine W (2022) Petiole length reduction is an indicator of waterlogging stress for Trifolium subterraneum ssp. yanninicum. Plant and Soil 475, 645–667.
| Petiole length reduction is an indicator of waterlogging stress for Trifolium subterraneum ssp. yanninicum.Crossref | GoogleScholarGoogle Scholar |
Falconer DS, Mackay TFC (1996) ‘Introduction to quantitative genetics.’ (Pearson/Longman: Burnt Mill, Harlow, England)
Foster KJ, Blache D, Watts TJ, Kontoolas M, Martin GB, Vercoe PE, Durmic Z, Kidd D, Wyrwoll CS, Smith JT, Ryan MH (2021) Clover disease across southern Australia: what we know now. Animal Production Science 61, Ivii
Francis CM (1976a) Observations on the ecology of subterranean clover in Greece and Crete: a report based on a seed collection tour 1976. Western Australian Dept. of Agriculture, Perth, WA.
Francis CM (1976b) Trikkala, a new safe clover for wet areas. Journal of the Department of Agriculture, Western Australia 17, 2–4.
Francis CM, Devitt AC (1969) The effect of waterlogging on the growth and isoflavone concentration of Trifolium subterraneum L. Australian Journal of Agricultural Research 20, 819–825.
| The effect of waterlogging on the growth and isoflavone concentration of Trifolium subterraneum L.Crossref | GoogleScholarGoogle Scholar |
Francis CM, Millington AJ (1965) Varietal variation in the isoflavone content of subterranean clover: its estimation by a microtechnique. Australian Journal of Agricultural Research 16, 557–564.
| Varietal variation in the isoflavone content of subterranean clover: its estimation by a microtechnique.Crossref | GoogleScholarGoogle Scholar |
Ghamkhar K, Snowball R, Bennett SJ (2007) Ecogeographical studies identify diversity and potential gaps in the largest germplasm collection of bladder clover (Trifolium spumosum L.). Australian Journal of Agricultural Research 58, 728–738.
| Ecogeographical studies identify diversity and potential gaps in the largest germplasm collection of bladder clover (Trifolium spumosum L.).Crossref | GoogleScholarGoogle Scholar |
Ghamkhar K, Nichols PGH, Erskine W, Snowball R, Murillo M, Appels R, Ryan MH (2015) Hotspots and gaps in the world collection of subterranean clover (Trifolium subterraneum L.). The Journal of Agricultural Science 153, 1069–1083.
| Hotspots and gaps in the world collection of subterranean clover (Trifolium subterraneum L.).Crossref | GoogleScholarGoogle Scholar |
Guggolz J, Livingston AL, Bickoff EM (1961) Forage estrogens, detection of daizein, formononetin, genistein, and biochanin A in forages. Journal of Agricultural and Food Chemistry 9, 330–332.
| Forage estrogens, detection of daizein, formononetin, genistein, and biochanin A in forages.Crossref | GoogleScholarGoogle Scholar |
Hamilton G, Bakker D, Houlebrook D, Spann C (2000) Raised beds prevent waterlogging and increase productivity. Australian Journal of Soil Research 43, 575–585.
Harborne JB (1967) ‘Comparative biochemistry of the flavonoids.’ (Academic Press: London, UK)
Herms DA, Mattson WJ (1992) The dilemma of plants: to grow or defend. The Quarterly Review of Biology 67, 283–335.
| The dilemma of plants: to grow or defend.Crossref | GoogleScholarGoogle Scholar |
Herzog M, Pedersen O (2014) Partial versus complete submergence: snorkelling aids root aeration in Rumex palustris but not in R. acetosa. Plant, Cell & Environment 37, 2381–2390.
Hijmans RJ, Cameron SE, Parra JL, Jones PG, Jarvis A (2005) Very high resolution interpolated climate surfaces for global land areas. International Journal of Climatology 25, 1965–1978.
| Very high resolution interpolated climate surfaces for global land areas.Crossref | GoogleScholarGoogle Scholar |
Hill MJ (1996) Defining the white clover zone in eastern mainland Australia using a model and a geographic information system. Ecological Modelling 86, 245–252.
| Defining the white clover zone in eastern mainland Australia using a model and a geographic information system.Crossref | GoogleScholarGoogle Scholar |
Hill MJ, Donald GE (1998) ‘Australian temperate pasture database [CD-ROM].’ (National Pasture Improvement Coordinating Committee, CSIRO Australia: Melbourne, Vic., Australia)
Humphries AW (1962) The growth of some perennial grasses in waterlogged soil. I. The effect of waterlogging on the availability of nitrogen and phosphorus to the plant. Australian Journal of Agricultural Research 13, 414–425.
| The growth of some perennial grasses in waterlogged soil. I. The effect of waterlogging on the availability of nitrogen and phosphorus to the plant.Crossref | GoogleScholarGoogle Scholar |
Hungerford TG (1975) ‘Diseases of livestock.’ (McGraw-Hill: Sydney, NSW, Australia)
Hunt R (1982) ‘Plant growth curves: the functional approach to plant growth analysis.’ (Edward Arnold: London, UK)
Jones CG, Hartley SE (1999) A protein competition model of phenolic allocation. Oikos 86, 27–44.
| A protein competition model of phenolic allocation.Crossref | GoogleScholarGoogle Scholar |
Katznelson J, Morley FHW (1965) A taxonomic revison of sect. Calycomorphum of the genus Trifolium. I. The geocarpic species. Israel Journal of Botany 14, 112–134.
Malik AI, Colmer TD, Lambers H, Setter TL, Schortemeyer M (2002) Short-term waterlogging has long-term effects on the growth and physiology of wheat. New Phytologist 153, 225–236.
| Short-term waterlogging has long-term effects on the growth and physiology of wheat.Crossref | GoogleScholarGoogle Scholar |
Margna U, Margna E, VainjÄrv T (1989) Influence of nitrogen nutrition on the utilization of L-phenylalanine for building flavonoids in buckwheat seedling tissues. Journal of Plant Physiology 134, 697–702.
| Influence of nitrogen nutrition on the utilization of L-phenylalanine for building flavonoids in buckwheat seedling tissues.Crossref | GoogleScholarGoogle Scholar |
Marley CL, McCalman H, Buckingham S, Downes D, Abberton MT (2011) A review of the effect of legumes on ewe and cow fertility. Institute of Biological, Environmental and Rural Sciences, Gogerddan, Aberystwyth University.
Marshall T, Millington AJ (1967) Flooding tolerance of some Western Australian pasture legumes. Australian Journal of Experimental Agriculture 7, 367–371.
| Flooding tolerance of some Western Australian pasture legumes.Crossref | GoogleScholarGoogle Scholar |
Millington AJ, Francis CM, McKeown NR (1964) Wether bioassay of animal pasture legumes. II. The oestrogenic activity of nine strains of Trifolium subterraneum L. Australian Journal of Agricultural Research 15, 527–536.
| Wether bioassay of animal pasture legumes. II. The oestrogenic activity of nine strains of Trifolium subterraneum L.Crossref | GoogleScholarGoogle Scholar |
Morley FHW, Francis CM (1968) Varietal and environmental variations in isoflavone concentrations in subterranean clover (Trifolium subterraneum L.). Australian Journal of Agricultural Research 19, 15–26.
| Varietal and environmental variations in isoflavone concentrations in subterranean clover (Trifolium subterraneum L.).Crossref | GoogleScholarGoogle Scholar |
Nakai A, Yurugi Y, Kisanuki H (2009) Growth responses of Salix gracilistyla cuttings to a range of substrate moisture and oxygen availability. Ecological Research 24, 1057–1065.
| Growth responses of Salix gracilistyla cuttings to a range of substrate moisture and oxygen availability.Crossref | GoogleScholarGoogle Scholar |
Nichols PGH (2004) Evolution in sown mixtures of subterranean clover (Trifolium subterraneum L.). PhD Thesis, University of Western Australia.
Nichols PGH, Collins WJ, Barbetti MJ (1996) ‘Registered cultivars of subterranean clover -their characteristics, origin and identification.’ Bulletin No. 4327. (Chief executive officer, Agriculture Western Australia)
Nichols PGH, Foster KJ, Piano E, Pecetti L, Kaur P, Ghamkhar K, Collins WJ (2013) Genetic improvement of subterranean clover (Trifolium subterraneum L.). 1. Germplasm, traits and future prospects. Crop & Pasture Science 64, 312–346.
| Genetic improvement of subterranean clover (Trifolium subterraneum L.). 1. Germplasm, traits and future prospects.Crossref | GoogleScholarGoogle Scholar |
Nichols PGH, Jones RAC, Ridsdill-Smith TJ, Barbetti MJ (2014) Genetic improvement of subterranean clover (Trifolium subterraneum L.). 2. Breeding for disease and pest resistance. Crop & Pasture Science 65, 1207–1229.
| Genetic improvement of subterranean clover (Trifolium subterraneum L.). 2. Breeding for disease and pest resistance.Crossref | GoogleScholarGoogle Scholar |
Oram RN (1989) ‘Register of Australian herbage plant cultivars.’ (Australian Herbage Plant Registration Authority, Division of Plant Industry, CSIRO: Melbourne, Vic., Australia)
Reed KFM (2016) Fertility of herbivores consuming phytoestrogen-containing Medicago and Trifolium species. Agriculture 6, 35
| Fertility of herbivores consuming phytoestrogen-containing Medicago and Trifolium species.Crossref | GoogleScholarGoogle Scholar |
Reed KFM, Schroder PM, Eales JW, McDonald RM, Chin JF (1985) Comparative productivity of Trifolium subterraneum and T. yanninicum in south-western Victoria. Australian Journal of Experimental Agriculture 25, 351–361.
| Comparative productivity of Trifolium subterraneum and T. yanninicum in south-western Victoria.Crossref | GoogleScholarGoogle Scholar |
Rossiter RC (1970a) Factors affecting the oestrogen content of subterranean clover pastures. Australian Veterinary Journal 46, 141–144.
| Factors affecting the oestrogen content of subterranean clover pastures.Crossref | GoogleScholarGoogle Scholar |
Rossiter RC (1970b) Physiological and ecological studies on the oestrogenic isoflavones in subterranean clover, (T. subterraneum L.) VIII. Phosphate supply in relation to temperature and to leaf development. Australian Journal of Agricultural Research 21, 593–600.
| Physiological and ecological studies on the oestrogenic isoflavones in subterranean clover, (T. subterraneum L.) VIII. Phosphate supply in relation to temperature and to leaf development.Crossref | GoogleScholarGoogle Scholar |
Rossiter RC (1972) Physiological and ecological studies on the oestrogenic isoflavones in subterranean clover (T. subterraneum L.). X.* Isoflavone formation and carbohydrate metabolism. Australian Journal of Agricultural Research 23, 419–426.
| Physiological and ecological studies on the oestrogenic isoflavones in subterranean clover (T. subterraneum L.). X.* Isoflavone formation and carbohydrate metabolism.Crossref | GoogleScholarGoogle Scholar |
Rossiter RC (1989) Evaluation of genotypes of subterranean clover in a Mediterranean environment; a personal and historical account. In ‘Proceedings of the 5th Australian agronomy conference. Perth, WA, Australia’. (The Australian Society of Agronomy, CSIRO: Perth, WA, Australia)
Rossiter RC, Beck AB (1966) Physiological and ecological studies on the oestrogenic isoflavones in subterranean clover (T. subterraneum L.) I. Effects of temperature. Australian Journal of Agricultural Research 17, 29–37.
| Physiological and ecological studies on the oestrogenic isoflavones in subterranean clover (T. subterraneum L.) I. Effects of temperature.Crossref | GoogleScholarGoogle Scholar |
Setter TL, Waters I (2003) Review of prospects for germplasm improvement for waterlogging tolerance in wheat, barley and oats. Plant and Soil 253, 1–34.
| Review of prospects for germplasm improvement for waterlogging tolerance in wheat, barley and oats.Crossref | GoogleScholarGoogle Scholar |
Shutt DA, Braden AWH (1968) The significance of equol in relation to the oestrogenic responses in sheep ingesting clover with a high formononetin content. Australian Journal of Agricultural Research 19, 545–553.
| The significance of equol in relation to the oestrogenic responses in sheep ingesting clover with a high formononetin content.Crossref | GoogleScholarGoogle Scholar |
Smith GR, Randel RD, Bradshaw C (1986) Influence of harvest date, cultivar, and sample storage method on concentration of isoflavones in subterranean clover. Crop Science 26, 1013–1016.
| Influence of harvest date, cultivar, and sample storage method on concentration of isoflavones in subterranean clover.Crossref | GoogleScholarGoogle Scholar |
Striker GG (2012) Time is on our side: the importance of considering a recovery period when assessing flooding tolerance in plants. Ecological Research 27, 983–987.
| Time is on our side: the importance of considering a recovery period when assessing flooding tolerance in plants.Crossref | GoogleScholarGoogle Scholar |
Striker GG, Insausti P, Grimoldi AA (2008) Flooding effects on plants recovering from defoliation in Paspalum dilatatum and Lotus tenuis. Annals of Botany 102, 247–254.
| Flooding effects on plants recovering from defoliation in Paspalum dilatatum and Lotus tenuis.Crossref | GoogleScholarGoogle Scholar |
Walker SK, Kleeman DO, Bawden CS (2002) Sheep reproduction in Australia – current status and potential for improvement through flock management and gene discovery. MS.009. South Australia Research and Development Institute No. MS.009, Meat and Livestock Australia, Sydney.
Williams RF, Evans LT, Ludwig LJ (1964) Estimation of leaf area for clover and lucerne. Australian Journal of Agricultural Research 15, 231–233.
| Estimation of leaf area for clover and lucerne.Crossref | GoogleScholarGoogle Scholar |