Informing the management of the post-COVID condition: insights from the Western Australian experience comparing those who tested positive and negative to early COVID-19 strains
Kristen Grove A , Vinicius Cavalheri

A
B
C
D
E
F
G
H
I
J
K
L
M
N
O
P
Q
R
S
T
U
Abstract
This study aimed to compare the relative physical recovery and symptoms after SARS-CoV-2 infection between groups confirmed positive or negative to early strains of COVID-19.
A prospective, longitudinal cohort study compared outcomes of metropolitan adults polymerase chain reaction-tested for COVID-19 between March and November 2020 in Western Australia. Control matching was attempted: inpatients (gender, age) and ambulatory clinic (gender, age, asthma, chronic pulmonary disease). One-year follow-up involved three repeated measures: physical function (grip strength and 1-min sit-to-stand) and patient-reported outcomes (Fatigue Severity Scale, modified Medical Research Council dyspnoea scale and Euroqol-5D-5L).
Three hundred and forty-four participants were recruited (154 COVID+, age 54 ± 18 years, 75 females [49%]); 190 COVID−, age 52 ± 16 years, 67 females [35%]) prior to national vaccination roll-out. No between-group differences in physical function measures were evident at any time point. Fatigue (OR 6.62, 95% CI 2.74–15.97) and dyspnoea (OR 2.21, 95% CI 1.14–4.30) were higher in the COVID+ group at second assessment (T2). On Euroqol-5D-5L, no between-group differences were evident in the physical function domains of self-care, mobility or usual activities at any time point. However, COVID+ participants were less likely to report an absence of anxiety or depression symptoms at T2 (OR 0.41, 95% CI 0.19–0.89).
Neither statistical nor clinically meaningful differences in physical function were evident between COVID+ and COVID− participants to 12-months after acute illness. Symptoms of fatigue, dyspnoea, anxiety or depression were more prevalent in the COVID+ group til ~8 months after illness with between-group differences no longer evident at 1 year.
Keywords: anxiety, control group, COVID-19 virus infection, depression, dyspnoea, fatigue, pain, primary care, quality of life.
Introduction
Coronavirus disease 2019 (COVID-19) and subsequent long-COVID is a multi-system illness with effects across multiple health domains1 with consensus that chronic, mild to profound negative effects exist in physical and mental health,2 evoking a broad range of symptoms.3–5 The World Health Organization (WHO) and the Australian Department of Health and Aged Care defined long-COVID as the presence of symptoms at 3 months from onset of COVID-19, lasting at least 2 months, not attributable to any other cause.6,7
Interpreting and applying the international findings from long-COVID studies is challenging due to wide variation in definitions 8–10 as well as heterogeneity of symptoms, assessment tools and contexts11,12 and study designs.10,13 Studies of COVID-19-related impairment with control conditions have been limited although emerging evidence indicates that more recent strains of COVID-19 exhibit similar patterns of recovery to common illnesses such as influenza.10,14,15 Common features of long-COVID reported worldwide include fatigue, headache, dyspnoea, insomnia, anosmia, brain fog, anxiety and depression, with symptoms evident to 4 months post-illness.8,16–18 In Australia, symptomatic long-COVID data is limited and prevalence estimates range from 2 to 20%.19,20 Thus, due to study heterogeneity, logistics and design challenges faced by researchers during the pandemic, it is unknown if the early COVID-19 strains, encountered by pathogen naive populations around the world, also displayed latent symptom patterns similar to more recent strains or other endemic community acquired illnesses.
The Western Australia (WA) situation during the first wave of COVID-19 provided a unique environment within which to study the impacts of the original lineages of SARS-CoV-2 on virus naive, metropolitan adults. With limited confounding factors, our study21 was designed to provide insights into the post-acute sequelae of COVID-19 (PASC) and inform future health and community service needs to support individuals returning to work and participation.22,23 Thus, the aim of this study was to compare physical function and symptom recovery to 12 months in adults who tested positive to early strains of COVID-19 (COVID+) prior to state and national vaccination roll-out, relative to those confirmed negative (COVID−).
Methods
Study design
The Life AfTER covid-19 (LATER-19) study was a prospective, longitudinal cohort study.21,24,25 Data collection occurred at three metropolitan tertiary hospitals in WA. Ethics and Governance approvals were granted by the South Metropolitan Health Service Human Research Ethics Committee (HREC) (RGS4040) and reciprocal approvals were obtained at all participating sites. The Australian and New Zealand Clinical Trial Registration (ANZCTR) was ACTRN12621001067864.
Recruitment
Participants were screened and recruited at each site either as inpatients; via sister trial provisions in the International Severe Acute Respiratory and emerging Infection Consortium (ISARIC) study;26 or at on-site COVID-19 testing clinics. Participants were eligible if they: were ≥18 years old; attended with respiratory symptoms suggestive of COVID-19; and underwent a reverse transcription polymerase chain reaction (PCR) serology test. Participants were excluded if they had pre-existing: mental health diagnoses; communication or cognitive impairment impacting their ability to self-report; or physical impairment limiting participation in active measures.
Group allocation
Due to low numbers of confirmed COVID-19 cases at the time of recruitment, all those in WA with a positive result by PCR (COVID+) between March and November 2020 were approached. The WA Department of Health confirmed that Wuhan lineage B strain was dominant (February–December), with cases of lineage A (February–May) and D (June–July) also described in WA.27 Due to Government border measures, variants of concern, including B1.1.7 (alpha) were not detected in WA before mid-December 2020, after the recruitment period.28 Simultaneously, a purposeful sample of those with confirmed negative PCR results (COVID−) were matched with positive cases and offered study participation. The non-hospitalised participants were proximity-matched at a ratio of 5:1 for age, gender, and asthma and/or chronic obstructive pulmonary disease (COPD) from a pool of ~30,000 ambulatory clinic patients. When designing the study, asthma was emergent as a factor that may influence outcomes.29 Recruitment for the hospitalised sub-groups was challenging. In 2020 in WA, hospitals were prepared for overwhelming numbers of COVID-19 admissions, as reported elsewhere, thus hospitalisation for those COVID− was minimised and those with pre-existing respiratory conditions were strongly encouraged to self-isolate at home. The community transmission of COVID did not eventuate in WA compared to other states,24 thus, recruited COVID− inpatients were matched at a ratio of 1:1 by age; gender; attended with respiratory symptoms; and admitted to hospital for treatment of a respiratory complaint, during the recruitment period.
Procedures
LATER-19 data collection commenced July 2020 and ended November 2021. In-hospital admission status, length of stay (LOS) and participants' age, gender, weight, height and co-morbidities were recorded. Assessments were planned for three time points: three (T1; initial), 6 months (T2; second) and 12 months (T3; final) after PCR swab date. However, due to the time taken to gain approvals and secure workarounds for infection prevention restrictions, initial assessments mostly occurred after 3 months, with second follow-up (T2) scheduled at least 6 weeks later. Matched patients were contacted and preferentially scheduled for face-to-face attendance at one of three tertiary hospital research clinics. Due to hostile research conditions and rate of recruitment of COVID+ participants, the planned ratio for matched controls was not able to be achieved before the T2 assessment period. This resulted in a matching ratio of 1 COVID+ : 1.2 COVID−. If participants were unable to attend face-to-face appointments, telehealth appointments with electronic survey distribution options were offered. Those who participated via telehealth completed electronic surveys and only grip strength was omitted from physical assessments. Detailed accounts of the LATER-19 methods have been described earlier.24,25 The study was conducted wholly prior to the availability of COVID-19 vaccines in WA, thus no participants reported being vaccinated during the follow-up period.
Outcomes and outcome measures
Specific details of the assessment tools are described in the LATER-19 protocol.21
Physical function was ascertained by the following:
(i) Impairment in grip strength after acute illness: is a sentinel marker of the systemic effect of prolonged immobility and is linked to poor functional outcomes, frailty, presence of infection and increased mortality.30–32 Thus, grip strength dynamometry was recorded (in kg) as best of three efforts on the right hand. The minimal clinically important difference (MCID) in grip strength was interpreted as 5 kg.33
(ii) Performance in the 1-min sit-to-stand test (1STS): is indicative of functional capacity, quadriceps strength, health-related quality of life (HRQoL) and mortality in various respiratory illness populations.34–39 Thus, the second physical measure recorded was the completed repetitions of 1STS,40 with MCID interpretation and three repetitions.34,41 Grip strength and 1STS repetitions were also presented as percentages of predicted norms.42,43
Symptoms and HRQoL were assessed by the following:
(i) The Fatigue Severity Scale raw sum ranges between 7 and 63, with higher scores indicating greater fatigue.44 The MCID applied was 10.8.45 Prevalence of clinically important fatigue was described as percentage of group scoring >35.46
(ii) The modified Medical Research Council dyspnoea scale (mMRC) which comprises five statements47 that reflect levels of limitation in activities of daily life due to dyspnoea. The score ranges between 0 and 4, with higher scores reflecting greater dyspnoea. The prevalence of dyspnoea was described as percentage of group scoring >0 on mMRC, while percentage score >1 indicated prevalence of clinically meaningful breathlessness.48
(iii) EuroQoL (EQ-5D-5L) responses were captured and ‘no impairment’ rates by domain were analysed. The percentage of participants reporting >1 on an EQ-5D-5L domain (i.e. mobility, self-care, usual activities, pain/discomfort, anxiety/depression) represented those with poorer outcomes and groupwise comparisons to Australian normative data were made.49
Statistical analysis
Participants were grouped according to their PCR test result into the COVID+ or COVID− group. Continuous data were reported as either mean ± standard deviation (s.d.) or median (interquartile range). Categorical data were reported as percentages. Comparability of demographics, LOS, physical function, symptoms and HRQoL assessments at T1 between groups was confirmed using independent samples t-tests (or Mann–Whitney test for skewed continuous variables) or Chi-squared tests for categorical variables (or Fisher’s Exact tests with high representation [>20%] of expected frequencies smaller than five). Between-group differences at T2 and T3 were assessed with adjustment of: corresponding variables at T1; gender (male/female); hospitalisation (yes/no); and chronic pulmonary disease (yes/no), using multiple linear regression models or logistic regression models (see also Supplementary Table S1). To explore any possible effects with hospitalisation, post hoc analyses on the between-group differences at T2 and T3 were performed comparing hospitalised and non-hospitalised patients (see Supplementary Table S2). Where feasible, adjusted coefficients, odds ratios (OR) and respective 95% confidence intervals (CI) were reported. Statistical significance was set at an alpha level of 0.05. All statistical analyses were performed using Stata 14.2 (StataCorp, TX, US).
Results
Sample characteristics
After matching controls, ~1000 people were contacted and 154 COVID+ and 190 COVID– (n = 344) consented within the study timeframe (Fig. 1), with 293 (85%) available for all three assessments. To address deficits in the matching subgroups after T1, 51 people were recruited and assessed at T2 and T3 only. The study included 31.9% of local COVID-19 cases to 1 April 2020 (n = 392).50 The median (interquartile range) time between PCR and T1, T2 and T3 was 171 (51), 230 (47) and 362 (39) days or 5.7, 7.7 and 12 months, respectively. Analysis of recruitment and engagement to T2 has been previously described.24 At T3, 304 of the 344 (88.3%) participants were retained. The WA COVID-19 vaccination schedule commenced 10 May 2021 for those aged >40 years, by which time 251 of 304 (82.6%) participants completed all study assessments. No participants reported subsequent COVID-19 infection after recruitment or during data collection.
The age distribution was similar for COVID+ and COVID− participants (Table 1). The COVID+ group had a greater proportion of females (49% vs 35%), people who were overweight (73% vs 65%) but less with chronic pulmonary disease (5% vs 14%) (Table 1). The COVID+ group had more participants (23% vs 14%) who were admitted to hospital and had a median 7 days longer stay (P < 0.001) than those in the COVID− group. Furthermore, intensive care unit (ICU) admissions only occurred in the COVID+ group, which accounted for 37% of the hospitalised COVID+ group. The higher levels of inpatient care required by the COVID+ group has been discussed previously.25
COVID+ (n = 154) | COVID− (n = 190) | P-value D | ||
---|---|---|---|---|
Patient characteristics | ||||
Age (years), mean ± s.d. | 54.0 ± 17.6 | 52.4 ± 15.9 | 0.362 | |
Sex female, n (%) | 75 (48.7) | 67 (35.3) | 0.012 | |
BMI status (kg/m2), n (%) | 0.043 | |||
Underweight: BMI < 18.5 | 1 (0.7) | 6 (3.2) | ||
Normal weight: BMI 18.5–24.9 | 41 (26.6) | 65 (34.2) | ||
Overweight: BMI: 25–29.9 | 73 (47.4) | 65 (34.2) | ||
Obese: BMI ≥ 30 | 39 (25.3) | 54 (28.4) | ||
Chronic cardiac disease n (%) | 17 (11.0) | 16 (8.4) | 0.412 | |
Hypertension, n (%) | 44 (28.6) | 42 (22.1) | 0.168 | |
Chronic pulmonary disease, n (%) | 7 (4.6) | 27 (14.2) | 0.003 | |
Asthma, n (%) | 21 (13.6) | 31 (16.3) | 0.490 | |
Diabetes, n (%) | 16 (10.34) | 14 (7.4) | 0.323 | |
Admission details | ||||
Hospitalised, n (%) | 35 (22.7) | 27 (14.2) | 0.041 | |
Days of hospital stay, median (25th–75th percentile) | 10 (5–22) B | 3 (2–5) B | 0.001 | |
ICU admission, n (%) | 13 (37.1) C | 0 | <0.001 | |
Days of ICU stay, median (25th–75th percentile) | 3.5 (0–17.5) | 0 | 0.029 | |
Isolation period, n (%) | <0.001 | |||
<2 days | 1 (0.7) | 108 (56.8) | ||
3–14 days | 38 (24.7) | 66 (34.7) | ||
≥14 days | 115 (74.7) | 16 (8.4) |
Physical outcomes
Grip analysed as percentage predicted for age and sex, demonstrated no between-group difference at any of the three time points (Supplementary Tables S1, S2). Adjusted regression models demonstrated no evidence of difference in grip strength between COVID+ and COVID− participants at T2 and T3 (Table 2).
Outcome measures | At T2 | At T3 | |
---|---|---|---|
ORA (95% CI); P-valueD | ORA (95% CI); P-value | ||
Percentage predicted grip strengthC | −0.03 (−0.06, 0.00); P = 0.083B | 0.02 (−0.03, 0.06); P = 0.426B | |
Percentage predicted 1STS repetitionsC | 0.02 (−0.03, 0.06); P = 0.488B | 0.04 (−0.01, 0.10); P = 0.115B | |
Fatigue Severity Scale (score ≥36) | 6.62 (2.74, 15.97); P < 0.001 | 1.50 (0.67, 3.34); P = 0.326 | |
Dyspnoea-mMRC (score ≥1) | 2.21 (1.14, 4.30); P = 0.018 | 0.41 (0.05, 3.29); P = 0.402 | |
Mobility (EQ-5D-5L: score = 1) | 0.94 (0.24, 3.67); P = 0.924 | Model does not converge | |
Self-care (EQ-5D-5L: score = 1) | 1.02 (0.32, 3.23); P = 0.971 | 2.02 (0.49, 8.33); P = 0.329 | |
Usual activities (EQ-5D-5L: score = 1) | 0.78 (0.39, 1.55); P = 0.481 | 0.48 (0.21, 1.11); P = 0.085 | |
Pain/discomfort (EQ-5D-5L: score = 1) | 1.96 (1.03, 3.72); P = 0.040 | 0.90 (0.47, 1.73); P = 0.749 | |
Anxiety/depression (EQ-5D-5L: score = 1) | 0.41 (0.19, 0.89); P = 0.023 | 0.77 (0.40, 1.47); P = 0.424 |
Symptoms: survey responses
There were no between-group differences in fatigue at T1 or T3, but at T2, fatigue was reported by 34% and 14% of participants in the COVID+ and COVID− group, respectively (P < 0.001) (Supplementary Tables S1, S2; Fig. 2). After adjusting for covariates, the COVID+ group were more likely to report clinically relevant levels of fatigue at T2 (OR 6.62, 95% CI 2.74–15.97, P < 0.001; Table 2).
Prevalence of fatigue and dyspnoea by COVID status at T1, T2 and T3, with available Australian normative data.48
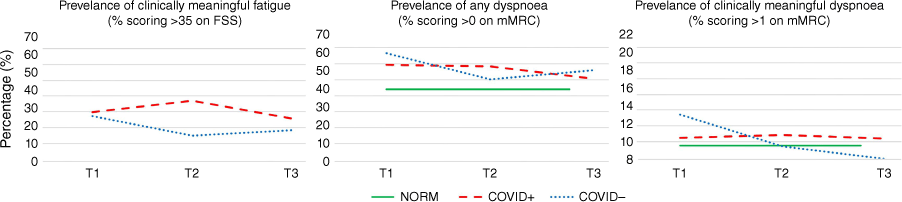
There were no between-group differences in dyspnoea at T1 or T3 (Supplementary Tables S1, S2). After adjustments, the COVID+ group were more likely to report dyspnoea at T2 (OR 2.21, 95% CI 1.14–4.30, P = 0.018). The trajectory of recovery of dyspnoea per mMRC scores is shown in Fig. 2.
Adjusted comparisons of all domains are presented in Fig. 3. EQ-5D-5L score between-group differences were not evident for self-care, mobility and usual activities domains at any time point (Supplementary Tables S1, S2). At T2, after adjustments, reported pain/discomfort was lower in the COVID+ group (OR 1.96, 95% CI 1.03–3.72, P = 0.040) as was an absence of anxiety/depression (OR 0.41, 95% CI 0.19–0.89, P = 0.023) (Table 2).
Percentage of no functional impairment on EuroQol EQ-5D-5L by COVID status at T2 and T3, with available Australian normative values.49
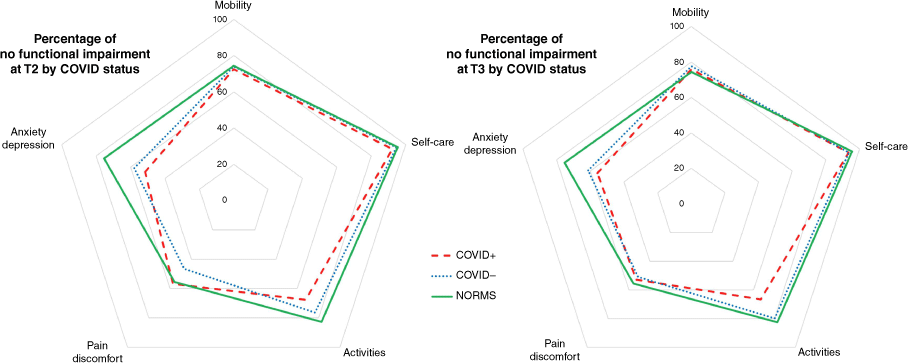
Discussion
Our findings show that there were no clinically meaningful or statistical differences in sentinel or reported physical function measures during the 12 months after acute infections. At 7–8 months (T2) after acute illness, significantly greater numbers in the COVID+ group reported symptoms of fatigue, dyspnoea and poorer mental health compared to the COVID− group and the normal population. However, reported pain was less prevalent in the COVID+ group. These results flag the negative impact of PASC symptoms on the ability of individuals to return to full capacity in previous employment and participation in leisure and social activities. At 1 year, all measures were comparable between groups. These results were observed regardless that the COVID+ group had less respiratory pre-morbid comorbidities and inpatients had longer hospital admissions where, unlike COVID− peers, 37% were admitted to ICU with an average stay of 4 days. In other words, after adjustment for known influencing factors, COVID-19 sufferers did not show meaningful differences in objective measures of physical function recovery trajectory compared to peers with other symptomatic respiratory illnesses. These COVID– flu-like community illnesses were not specified as a result of PCR testing.
Fatigue is often the most prevalent feature of long-COVID8,17,51,52 and dyspnoea is also often reported.17,53–56 Our COVID+ cohort reported three times greater prevalence (34%) of clinically meaningful fatigue ~8 months after illness, compared to peers. Interestingly, similar though lower rates of fatigue (25%) at ~12 months were described by others finding 28% in predominantly hospitalised55,57 and 33.1% in non-hospitalised58 cohorts. In addition, this study showed all participants at T1 and T2 reported higher than community norm symptoms of anxiety/depression, possibly demonstrating the effect of isolation and concurrently reduced physical activity on all participants. Furthermore, the COVID+ group reported prevalence of anxiety/depression 10% higher than comparable cohorts at T2.54,55,59,60 The findings indicate that virus naive, unvaccinated sufferers of early COVID-19 strains complained of PASC for much longer than reported in the subsequent systematic reviews of studies involving various viral mutations and vaccination status confounders.16,55,61 Recent literature suggests that the most prevalent negative PASCs are reported to be present irrespective of the COVID-19 virus strain.14,16,19,20 Moving forward, although there is a view that long-COVID be managed as per any other viral community illness,7,14,20 this study suggests it is reasonable to expect that, after COVID infection, some patients may require additional support and/or workplace adjustments for at least 8 months to accommodate negative PASC symptoms. Our results add weight to the notion of an impactful, prolonged, neurotoxic mechanism related to COVID-19 infection.62,63 Best practice for patients with neurological conditions includes access to early clinical input which poses challenges in current care models for those with PASC in Australia, where waiting periods of 6–9 months are common.
In Australia, Federal health authorities deliberated research-informed strategies64,65 and sought to optimise models of care to identify and manage those suffering impairing PASC and to facilitate timely effective management. Initially, ‘Long-COVID Clinics’ were established to manage a large, predicted volume of those requiring intervention. However, most jurisdictions have since ceased or evolved these services because the experience of many Long-COVID Clinics was that waitlists of >6–9 months were rapidly developed, and many patients recovered prior to their first assessment.66 Our results provide evidence to support this change with significantly more negative symptoms reported for ~7–9 months compared to COVID− peers and Australian norms. Prolonged presence of these symptoms influences HrQoL and likely have socioeconomic consequences, such as affecting capacity to return to work, as a high proportion of participants were of working age. However, we did not investigate this specifically in this study.
Strengths and limitations
The circumstances of the WA environment and COVID-19 responses reduced confounders evident in other research and strengthened this study. The geographical isolation and Government-imposed public health measures, including mandatory PCR testing for symptomatic individuals, resulted in negligible community spread of COVID-19. The WA healthcare system and formal COVID-19 testing capabilities were never overwhelmed. Thus, all citizens had access to active contemporary critical, in-hospital and ambulatory treatments during the entire study period, in contrast to many COVID-19 studies where multiple confounders existed.59,67–70 These system factors, and the desire of the WA community to help ‘fight’ COVID-19, drove the participants’ high groupwise return rate (85%) in this study. This enhances application of the findings as did prospective longitudinal data capture using valid and reliable consumer-centric self-reported measures. Although challenging, a clinically relevant comparison group was amassed prospectively, where this was not achieved in many other studies.70 No participants reported contracting subsequent COVID-19 or other illnesses during the study period; however, confounding is possible as we relied only on initial PCR results to identify COVID− and COVID+ cases and subsequent re-infection, while very unlikely and not reported by any participants, was possible. The study results were unlikely to be confounded by vaccination status as the roll out did not commence before the bulk of participants had completed their final assessment and vaccination may be protective for PASC.61
The higher incidence of mental health symptoms reported by our cohorts may be attributable to strong public health messaging and measures designed to minimise and contain COVID-19.71 The stigma towards COVID-19 among the WA community, and specifically for those who contracted COVID-19, may have influenced the results. Furthermore, the community messaging towards hospital avoidance for those with pre-existing diagnoses likely contributed to: the difficulty in achieving a matched, hospitalised control sub-group; the higher prevalence of COPD in the COVID– group; and COVID– participants not recruited in time to contribute data at T1. In 2024, females (8.6%) were reported to have long-COVID more than males (5.1%).72 Thus, while it is unclear if these recent results apply in a virus-naive context, it is possible that the higher proportion of females in the COVID+ group may over-estimate the reported symptoms compared to the COVID− group in this study. While analyses were adjusted for the imbalance of those with chronic pulmonary disease, this may impede clinical interpretation of results. The COVID− group prevalence of chronic pulmonary disease was higher than the national average.73 Furthermore, COVID-19 testing protocols at the time required hospital-based PCR testing of any person with respiratory symptoms. That said, selection bias likely led to underestimation of between-group differences as chronic pulmonary disease negatively effects dyspnoea, fatigue, physical function and mental health.74–76 While grip strength was recorded bilaterally, hand dominance was not recorded or adjusted for in right-grip statistical analyses. This was because while strength differs up to 10% for right-hand dominant persons, the difference for left-hand dominant persons is minimal.77,78 Lastly, this study reflects the outcomes after contracting early strains of SARS CoV2 in an unvaccinated, virus-naive population. Vaccination status was not recorded at the final assessment. Studies on the association of vaccination on the prevalence and severity of PASC are conflicting79–81 thus generalising the results of this research to vaccinated or partially vaccinated populations is cautioned.
Conclusion
In this study, there was no evidence of relative differences in physical function at any time point to 1-year post-infection. The COVID+ group reported more fatigue, dyspnoea and anxiety/depression than their COVID− peers at 8 months after acute illness. Both groups returned to similar symptom levels after 1 year on all domains. Our findings warrant that health authorities and employers must consider adding reasonable accommodations for up to 8 months in those reporting post-acute sequelae of COVID-19.
Data availability
The datasets generated and/or analysed during the current study are not publicly available as publication of clinical datasets was not included in the application for ethics approval. De-identified datasets are available from the corresponding author on request, which involves the execution of a data sharing agreement.
Declaration of funding
Funding for the LATER-19 Trial was sourced through a grant from the Department of Health in conjunction with the Western Australian Health Translation Network (WAHTN COVID-19 Research Grants Program, no # applicable). Open Access publication is funded jointly by South Metropolitan Health Service Open Access Publication Fund, Fiona Stanley Hospital Allied Health Department, and WA Department of Health/WAHTN COVID-19 Research Grants Program.
Acknowledgements
Consumers and patients: The LATER-19 investigative team are most grateful to JP and AW who joined the group as consumer representatives at the start of this journey. We acknowledge and thank them for their valuable input into the design of this study and taking the time to help with review and editing of all the approval documents, patient-facing collateral and study reports. We also thank all patients who participated and took the time to return for face-to-face follow-up, maintaining strong support for this project for a year after deciding to participate. Participant enthusiasm and willingness to help in the efforts to try and unravel the long-term issues faced after COVID-19 was palpable, and the LATER-19 team were constantly reminded of the generosity of spirit and time given by the patients who helped us. Thank you all.
Author contributions
DWE, VC: funding source and allocation. SM, KG, VN, EH, D WE: recruitment and data collection. KG: initial data cleaning and analysis. HJC: statistical analysis. KG, VN, DWE, VC, MH: drafting of manuscript. KG, DWE, VC, HJC, VN, MH, JT, SM, CW, AM, JP, EH: revision of manuscript. All authors reviewed all drafts and approved the final manuscript.
References
1 Nalbandian A, Sehgal K, Gupta A, Madhavan MV, McGroder C, Stevens JS, et al. Post-acute COVID-19 syndrome. Nat Med 2021; 27(4): 601-15.
| Crossref | Google Scholar | PubMed |
3 Huang C, Huang L, Wang Y, Li X, Ren L, Gu X, et al. 6-month consequences of COVID-19 in patients discharged from hospital: a cohort study. Lancet 2021; 397(10270): 220-32.
| Crossref | Google Scholar | PubMed |
4 Carfì A, Bernabei R, Landi F, Gemelli Against COVID-19 Post-Acute Care Study Group.. Persistent Symptoms in Patients After Acute COVID-19. JAMA 2020; 324(6): 603-5.
| Crossref | Google Scholar | PubMed |
5 The Writing Committee for the COMEBAC Study Group.. Four-Month Clinical Status of a Cohort of Patients After Hospitalization for COVID-19. JAMA 2021; 325(15): 1525-34.
| Crossref | Google Scholar | PubMed |
6 World Health Organization. A clinical case definition of post COVID-19 condition by a Delphi consensus, 6 October 2021. WHO; 2021. Available at https://www.who.int/publications/i/item/WHO-2019-nCoV-Post_COVID-19_condition-Clinical_case_definition-2021.1 [cited 12 February 2024].
7 Butler M. National Post-Acute Sequelae of COVID-19 (PASC) Plan. Canberra: Australian Government; 2024. Available at https://www.health.gov.au/resources/publications/national-post-acute-sequelae-of-covid-19-plan?language=en
8 Office for National Statistics. Prevalence of Ongoing Symptoms Following Coronavirus (COVID-19) Infection in the UK: 30 March 2023. London: ONS; 2023. Available at https://www.ons.gov.uk/peoplepopulationandcommunity/healthandsocialcare/conditionsanddiseases/bulletins/prevalenceofongoingsymptomsfollowingcoronaviruscovid19infectionintheuk/30march2023 [cited 12 Feburary 2024].
9 Ledford H. How common is long COVID? Why studies give different answers. Nature 2022; 606: 852-3.
| Crossref | Google Scholar | PubMed |
10 Australian Institute of Health and Welfare. Long COVID in Australia - a review of the literature. Canberra: AIHW; 2022. Available at https://www.aihw.gov.au/reports/covid-19/long-covid-in-australia-a-review-of-the-literature/summary [updated 16 December 2022]
11 Beauchamp M, Kirkwood R, Duong M, Ho T, Raina P, Kruisselbrink R, et al. Long-Term Functional Limitations and Predictors of Recovery after COVID-19: A Multicenter Prospective Cohort Study. Am J Med 2024;
| Crossref | Google Scholar | PubMed |
12 Naik H, Wilton J, Tran KC, Janjua NZ, Levin A, Zhang W. Long-term Health-related Quality of Life in Working-age COVID-19 Survivors: A Cross-sectional Study. Am J Med 2024;
| Crossref | Google Scholar | PubMed |
13 Munblit D, Nicholson TR, Needham DM, Seylanova N, Parr C, Chen J, et al. Studying the post-COVID-19 condition: research challenges, strategies, and importance of Core Outcome Set development. BMC Med 2022; 20(1): 50.
| Crossref | Google Scholar | PubMed |
14 Brown M, Gerrard J, McKinlay L, Marquess J, Sparrow T, Andrews R. Ongoing symptoms and functional impairment 12 weeks after testing positive for SARSCoV-2 or influenza in Australia: an observational cohort study. BMJ Public Health 2023; 1(1): e000060.
| Crossref | Google Scholar |
16 O’Mahoney LL, Routen A, Gillies C, Ekezie W, Welford A, Zhang A, et al. The prevalence and long-term health effects of Long Covid among hospitalised and non-hospitalised populations: a systematic review and meta-analysis. EClinicalMedicine 2023; 55: 101762.
| Crossref | Google Scholar | PubMed |
17 Lopez-Leon S, Wegman-Ostrosky T, Perelman C, Sepulveda R, Rebolledo PA, Cuapio A, et al. More than 50 long-term effects of COVID-19: a systematic review and meta-analysis. Sci Rep 2021; 11(1): 16144.
| Crossref | Google Scholar | PubMed |
18 Bliddal S, Banasik K, Pedersen OB, Nissen J, Cantwell L, Schwinn M, et al. Acute and persistent symptoms in non-hospitalized PCR-confirmed COVID-19 patients. Sci Rep 2021; 11(1): 13153.
| Crossref | Google Scholar | PubMed |
19 Australian Institute of Health and Welfare. Long COVID in Australia – a review of the literature. Canberra: Australian Government; 2022. Available at https://www.aihw.gov.au/reports/covid-19/long-covid-in-australia-a-review-of-the-literature/summary
21 Edgar DW, Gittings P, van der Lee L, Naylor L, Maiorana A, Jacques A, et al. Life AfTER covid-19 (LATER-19): a protocol for a prospective, longitudinal, cohort study of symptoms, physical function and psychological outcomes in the context of a pandemic. Tasman Med J 2021; 3(1): 1-10.
| Google Scholar |
22 Shah W, Hillman T, Playford ED, Hishmeh L. Managing the long term effects of covid-19: summary of NICE, SIGN, and RCGP rapid guideline. BMJ 2021; 372: n136.
| Crossref | Google Scholar | PubMed |
23 Duddy C, Powell T, Kirk-Wade E, Brione P, Hobson F, Long R. House of Commons Library briefing paper: Number 9112, 29 February 2024: Coronavirus: Long COVID. 2024. Available at https://commonslibrary.parliament.uk/research-briefings/cbp-9112/
24 Grove K, Harrold M, Mohd S, Natarajan V, Hurn E, Pearce J, et al. Research lessons during the COVID-19 pandemic: collecting longitudinal physical and mental health outcomes. Arch Public Health 2022; 80(1): 14.
| Crossref | Google Scholar | PubMed |
25 Grove K, Edgar DW, Chih H, Harrold M, Natarajan V, Mohd S, et al. Greater In-Hospital Care and Early Rehabilitation Needs in People with COVID-19 Compared with Those without COVID-19. J Clin Med 2022; 11(13): 3602.
| Crossref | Google Scholar | PubMed |
26 Sigfrid L, Cevik M, Jesudason E, Lim WS, Rello J, Amuasi J, et al. What is the recovery rate and risk of long-term consequences following a diagnosis of COVID-19? A harmonised, global longitudinal observational study protocol. BMJ Open 2021; 11(3): e043887.
| Crossref | Google Scholar | PubMed |
27 O’Toole Á, Scher E, Underwood A, Jackson B, Hill V, McCrone JT, et al. Assignment of epidemiological lineages in an emerging pandemic using the pangolin tool. Virus Evol 2021; 7(2): veab064.
| Crossref | Google Scholar | PubMed |
28 Hutchinson D, Williams H, Stone H. COVID-19 Variants of Concern in Australia, September 2020-April 2021. J Global Biosecur 2021; 3(1):.
| Crossref | Google Scholar |
30 Gale CR, Martyn CN, Cooper C, Sayer AA. Grip strength, body composition, and mortality. Int J Epidemiol 2007; 36(1): 228-35.
| Crossref | Google Scholar | PubMed |
31 Rantanen T, Volpato S, Ferrucci L, Heikkinen E, Fried LP, Guralnik JM. Handgrip strength and cause-specific and total mortality in older disabled women: exploring the mechanism. J Am Geriatr Soc 2003; 51(5): 636-41.
| Crossref | Google Scholar | PubMed |
32 Syddall H, Cooper C, Martin F, Briggs R, Aihie Sayer A. Is grip strength a useful single marker of frailty? Age Ageing 2003; 32(6): 650-6.
| Crossref | Google Scholar | PubMed |
33 Bohannon RW. Minimal clinically important difference for grip strength: a systematic review. J Phys Ther Sci 2019; 31(1): 75-8.
| Crossref | Google Scholar | PubMed |
34 Crook S, Büsching G, Schultz K, Lehbert N, Jelusic D, Keusch S, et al. A multicentre validation of the 1-min sit-to-stand test in patients with COPD. Eur Respir J 2017; 49(3): 1601871.
| Crossref | Google Scholar | PubMed |
35 Chorin F, Cornu C, Beaune B, Frère J, Rahmani A. Sit to stand in elderly fallers vs non-fallers: new insights from force platform and electromyography data. Aging Clin Exp Res 2016; 28(5): 871-9.
| Crossref | Google Scholar | PubMed |
36 Puhan MA, Siebeling L, Zoller M, Muggensturm P, ter Riet G. Simple functional performance tests and mortality in COPD. Eur Respir J 2013; 42(4): 956-63.
| Crossref | Google Scholar | PubMed |
37 Zanini A, Aiello M, Cherubino F, Zampogna E, Azzola A, Chetta A, et al. The one repetition maximum test and the sit-to-stand test in the assessment of a specific pulmonary rehabilitation program on peripheral muscle strength in COPD patients. Int J Chron Obstruct Pulmon Dis 2015; 10: 2423-30.
| Crossref | Google Scholar | PubMed |
38 Briand J, Behal H, Chenivesse C, Wémeau-Stervinou L, Wallaert B. The 1-minute sit-to-stand test to detect exercise-induced oxygen desaturation in patients with interstitial lung disease. Ther Adv Respir Dis 2018; 12: 1753466618793028.
| Crossref | Google Scholar | PubMed |
39 Gruet M, Peyré-Tartaruga LA, Mely L, Vallier JM. The 1-Minute Sit-to-Stand Test in Adults With Cystic Fibrosis: Correlations With Cardiopulmonary Exercise Test, 6-Minute Walk Test, and Quadriceps Strength. Respir Care 2016; 61(12): 1620-8.
| Crossref | Google Scholar | PubMed |
40 Bohannon RW, Crouch R. 1-Minute Sit-to-Stand Test: SYSTEMATIC REVIEW OF PROCEDURES, PERFORMANCE, AND CLINIMETRIC PROPERTIES. J Cardiopulm Rehabil Prev 2019; 39(1): 2-8.
| Crossref | Google Scholar | PubMed |
41 Vaidya T, de Bisschop C, Beaumont M, Ouksel H, Jean V, Dessables F, et al. Is the 1-minute sit-to-stand test a good tool for the evaluation of the impact of pulmonary rehabilitation? Determination of the minimal important difference in COPD. Int J Chron Obstruct Pulmon Dis 2016; 11: 2609-16.
| Crossref | Google Scholar | PubMed |
42 Werle S, Goldhahn J, Drerup S, Simmen BR, Sprott H, Herren DB. Age- and gender-specific normative data of grip and pinch strength in a healthy adult Swiss population. J Hand Surg Eur Vol 2009; 34(1): 76-84.
| Crossref | Google Scholar | PubMed |
43 Strassmann A, Steurer-Stey C, Lana KD, Zoller M, Turk AJ, Suter P, et al. Population-based reference values for the 1-min sit-to-stand test. Int J Public Health 2013; 58(6): 949-53.
| Crossref | Google Scholar | PubMed |
44 Valko PO, Bassetti CL, Bloch KE, Held U, Baumann CR. Validation of the fatigue severity scale in a Swiss cohort. Sleep 2008; 31(11): 1601-7.
| Crossref | Google Scholar | PubMed |
45 Impellizzeri FM, Agosti F, De Col A, Sartorio A. Psychometric properties of the Fatigue Severity Scale in obese patients. Health Qual Life Outcomes 2013; 11: 32.
| Crossref | Google Scholar | PubMed |
46 Learmonth YC, Dlugonski D, Pilutti LA, Sandroff BM, Klaren R, Motl RW. Psychometric properties of the Fatigue Severity Scale and the Modified Fatigue Impact Scale. J Neurol Sci 2013; 331(1-2): 102-7.
| Crossref | Google Scholar | PubMed |
47 Bestall JC, Paul EA, Garrod R, Garnham R, Jones PW, Wedzicha JA. Usefulness of the Medical Research Council (MRC) dyspnoea scale as a measure of disability in patients with chronic obstructive pulmonary disease. Thorax 1999; 54(7): 581-6.
| Crossref | Google Scholar | PubMed |
48 Poulos LM, Ampon RD, Currow DC, Marks GB, Toelle BG, Reddel HK. Prevalence and burden of breathlessness in Australian adults: The National Breathlessness Survey-a cross-sectional web-based population survey. Respirology 2021; 26(8): 768-75.
| Crossref | Google Scholar | PubMed |
49 McCaffrey N, Kaambwa B, Currow DC, Ratcliffe J. Health-related quality of life measured using the EQ-5D-5L: South Australian population norms. Health Qual Life Outcomes 2016; 14(1): 133.
| Crossref | Google Scholar | PubMed |
50 Department of Health. COVID-19 update – 1 April 2020. Government of Western Australia; 2020. Available at https://ww2.health.wa.gov.au/Media-releases/2020/COVID19-update-1-April-2020 [cited 2024 February 12].
51 Newman M. Chronic fatigue syndrome and long covid: moving beyond the controversy. BMJ 2021; 373: n1559.
| Crossref | Google Scholar | PubMed |
52 Stavem K, Ghanima W, Olsen MK, Gilboe HM, Einvik G. Prevalence and Determinants of Fatigue after COVID-19 in Non-Hospitalized Subjects: A Population-Based Study. Int J Environ Res Public Health 2021; 18(4): 2030.
| Crossref | Google Scholar | PubMed |
53 Seessle J, Waterboer T, Hippchen T, Simon J, Kirchner M, Lim A, et al. Persistent Symptoms in Adult Patients 1 Year After Coronavirus Disease 2019 (COVID-19): A Prospective Cohort Study. Clin Infect Dis 2022; 74(7): 1191-8.
| Crossref | Google Scholar | PubMed |
54 Hastie CE, Lowe DJ, McAuley A, Winter AJ, Mills NL, Black C, et al. Outcomes among confirmed cases and a matched comparison group in the Long-COVID in Scotland study. Nat Commun 2022; 13(1): 5663.
| Crossref | Google Scholar | PubMed |
55 Han Q, Zheng B, Daines L, Sheikh A. Long-Term Sequelae of COVID-19: A Systematic Review and Meta-Analysis of One-Year Follow-Up Studies on Post-COVID Symptoms. Pathogens 2022; 11(2): 269.
| Crossref | Google Scholar | PubMed |
56 van Kessel SAM, Olde Hartman TC, Lucassen P, van Jaarsveld CHM. Post-acute and long-COVID-19 symptoms in patients with mild diseases: a systematic review. Fam Pract 2022; 39(1): 159-67.
| Crossref | Google Scholar | PubMed |
57 Raman B, Cassar MP, Tunnicliffe EM, Filippini N, Griffanti L, Alfaro-Almagro F, et al. Medium-term effects of SARS-CoV-2 infection on multiple vital organs, exercise capacity, cognition, quality of life and mental health, post-hospital discharge. EClinicalMedicine 2021; 31: 100683.
| Crossref | Google Scholar | PubMed |
58 Tan S, Pryor AJG, Melville GW, Fischer O, Hewitt L, Davis KJ. The lingering symptoms of post-COVID-19 condition (long-COVID): a prospective cohort study. Intern Med J 2024; 54(2): 224-33.
| Crossref | Google Scholar | PubMed |
59 Hodgson CL, Higgins AM, Bailey MJ, Mather AM, Beach L, Bellomo R, et al. The impact of COVID-19 critical illness on new disability, functional outcomes and return to work at 6 months: a prospective cohort study. Crit Care 2021; 25(1): 382.
| Crossref | Google Scholar | PubMed |
60 Malik P, Patel K, Pinto C, Jaiswal R, Tirupathi R, Pillai S, et al. Post-acute COVID-19 syndrome (PCS) and health-related quality of life (HRQoL)-A systematic review and meta-analysis. J Med Virol 2022; 94(1): 253-62.
| Crossref | Google Scholar | PubMed |
61 Watanabe A, Iwagami M, Yasuhara J, Takagi H, Kuno T. Protective effect of COVID-19 vaccination against long COVID syndrome: A systematic review and meta-analysis. Vaccine 2023; 41(11): 1783-90.
| Crossref | Google Scholar | PubMed |
62 Holmes E, Wist J, Masuda R, Lodge S, Nitschke P, Kimhofer T, et al. Incomplete Systemic Recovery and Metabolic Phenoreversion in Post-Acute-Phase Nonhospitalized COVID-19 Patients: Implications for Assessment of Post-Acute COVID-19 Syndrome. J Proteome Res 2021; 20(6): 3315-29.
| Crossref | Google Scholar | PubMed |
63 Lawler NG, Gray N, Kimhofer T, Boughton B, Gay M, Yang R, et al. Systemic Perturbations in Amine and Kynurenine Metabolism Associated with Acute SARS-CoV-2 Infection and Inflammatory Cytokine Responses. J Proteome Res 2021; 20(5): 2796-811.
| Crossref | Google Scholar | PubMed |
64 Attwell K, Carlson S, Tchilingirian J, Harper T, McKenzie L, Roberts L, et al. Coronavax: preparing community and government for COVID-19 vaccination: a research protocol for a mixed methods social research project. BMJ Open 2021; 11(6): e049356.
| Crossref | Google Scholar | PubMed |
65 Roberts L, McKenzie L, Carlson SJ, Tomkinson S, Attwell K. Reopening to the world: how safety, normality and trust in government shape young adults’ COVID-19 vaccine intentions. Aust J Polit Sci 2022; 58(1): 105-23.
| Crossref | Google Scholar |
66 Menges D, Ballouz T, Anagnostopoulos A, Aschmann HE, Domenghino A, Fehr JS, et al. Burden of post-COVID-19 syndrome and implications for healthcare service planning: A population-based cohort study. PLoS One 2021; 16(7): e0254523.
| Crossref | Google Scholar | PubMed |
67 Burrell AJ, Pellegrini B, Salimi F, Begum H, Broadley T, Campbell LT, et al. Outcomes for patients with COVID-19 admitted to Australian intensive care units during the first four months of the pandemic. Med J Aust 2021; 214(1): 23-30.
| Crossref | Google Scholar | PubMed |
68 Hodgson CL, Higgins AM, Bailey MJ, Mather AM, Beach L, Bellomo R, et al. Comparison of 6-Month Outcomes of Survivors of COVID-19 versus Non-COVID-19 Critical Illness. Am J Respir Crit Care Med 2022; 205: 1159-68.
| Crossref | Google Scholar | PubMed |
69 Logue JK, Franko NM, McCulloch DJ, McDonald D, Magedson A, Wolf CR, et al. Sequelae in Adults at 6 Months After COVID-19 Infection. JAMA Netw Open 2021; 4(2): e210830.
| Crossref | Google Scholar | PubMed |
70 Haslam A, Prasad V. Comparability of Control and Comparison Groups in Studies Assessing Long COVID. Am J Med 2023;
| Crossref | Google Scholar | PubMed |
71 Jiang J, Akhlaghi H, Haywood D, Morrissey B, Parnis S. Mental health consequences of COVID-19 suppression strategies in Victoria, Australia: a narrative review. J Int Med Res 2022; 50(11): 3000605221134466.
| Crossref | Google Scholar | PubMed |
72 Fang Z, Ahrnsbrak R, Rekito A. Evidence Mounts That About 7% of US Adults Have Had Long COVID. JAMA 2024; 332: 5-6.
| Crossref | Google Scholar | PubMed |
73 Australian Bureau of Statistics. Chronic Obstructive Pulmonary Disease. Canberra: ABS; 2022. Available at https://www.abs.gov.au/statistics/health/health-conditions-and-risks/chronic-obstructive-pulmonary-disease/latest-release [cited 12 February 2024].
74 Lee J, Nguyen HQ, Jarrett ME, Mitchell PH, Pike KC, Fan VS. Effect of symptoms on physical performance in COPD. Heart Lung 2018; 47(2): 149-56.
| Crossref | Google Scholar | PubMed |
75 Clark LA, Reed R, Corazzini KN, Zhu S, Renn C, Jennifer Klinedinst N. COPD-Related Fatigue: A Scoping Review. Clin Nurs Res 2023; 32(5): 914-28.
| Crossref | Google Scholar | PubMed |
76 Martínez-Gestoso S, García-Sanz MT, Carreira JM, Salgado FJ, Calvo-Álvarez U, Doval-Oubiña L, et al. Impact of anxiety and depression on the prognosis of copd exacerbations. BMC Pulm Med 2022; 22(1): 169.
| Crossref | Google Scholar | PubMed |
77 Jarjour N, Lathrop JA, Meller TE, Roberts KS, Sopczak JM, Van Genderen KJ, et al. The 10% rule: grip strength and hand dominance in a factory population. Work 1997; 8(1): 83-91.
| Crossref | Google Scholar | PubMed |
78 Petersen P, Petrick M, Connor H, Conklin D. Grip strength and hand dominance: challenging the 10% rule. Am J Occup Ther 1989; 43(7): 444-7.
| Crossref | Google Scholar | PubMed |
79 Canas LS, Molteni E, Deng J, Sudre CH, Murray B, Kerfoot E, et al. Profiling post-COVID-19 condition across different variants of SARS-CoV-2: a prospective longitudinal study in unvaccinated wild-type, unvaccinated alpha-variant, and vaccinated delta-variant populations. Lancet Digit Health 2023; 5(7): e421-34.
| Crossref | Google Scholar | PubMed |
80 Du M, Ma Y, Deng J, Liu M, Liu J. Comparison of Long COVID-19 Caused by Different SARS-CoV-2 Strains: A Systematic Review and Meta-Analysis. Int J Environ Res Public Health 2022; 19(23): 16010.
| Crossref | Google Scholar | PubMed |
81 Byambasuren O, Stehlik P, Clark J, Alcorn K, Glasziou P. Effect of covid-19 vaccination on long covid: systematic review. BMJ Med 2023; 2(1): e000385.
| Crossref | Google Scholar | PubMed |