Water scarcity exacerbates feral ungulate use of ephemeral savanna waterholes in northern Australia
Helenna Mihailou


A
Abstract
Australian savannas evolved in the absence of hooved mammals and are therefore prone to disturbance from exotic ungulates. Several ungulate species have established feral populations in Australia’s northern savannas. Because most ungulate species have high water requirements, seasonal and interannual changes in water availability are likely to affect their behaviour and the extent of their impacts on native ecosystems. However, few studies have investigated how water scarcity affects feral ungulate use of waterpoints in Australia.
The aim of this study was to determine whether seasonal and interannual water scarcity affected the visitation behaviours of feral pigs, cattle and water buffalo at ephemeral savanna waterholes within Limmen National Park, Northern Territory, Australia.
We used motion-triggered wildlife cameras to study feral ungulate visitation and behaviour at 20 waterholes. Generalised linear mixed-effects models were used to investigate whether the number of visits, duration of visits and number of individuals visiting waterholes varied with year and dry season progression. We also investigated whether these factors affected the amount of time ungulates spent foraging, drinking and wallowing at waterholes.
All three species visited waterholes more often, for longer periods and in larger numbers during a drought year compared with an average rainfall year. Cattle and buffalo spent more time drinking from waterholes during the drought, and pigs and cattle spent longer periods foraging. Buffalo also wallowed more during the drought. Responses to dry season progression varied among species. Cattle visited waterholes more frequently, for longer durations and in larger herd sizes as the dry season progressed, whereas buffalo use did not change. Pigs only increased their visitation to waterholes when water scarcity was most extreme, at the end of the dry season during the drought.
Our results demonstrate that water scarcity exacerbates feral ungulate use of savanna waterholes. Management and control programs for feral ungulates in Australian savannas may benefit from targeting different species under specific water scarcity conditions.
Because climate change is predicted to reduce surface water availability in northern Australia, feral ungulate use of savanna waterholes may intensify, risking further biodiversity losses and irreversible ecosystem damage.
Keywords: cattle, drought, feral ungulates, invasive species, pig, tropical savanna, water buffalo, waterhole visitation.
Introduction
Northern Australia supports one of the largest savanna ecosystems in the world, which covers approximately 1 500 000 km2 (Woinarski et al. 2007). Australian savannas are thought to be particularly susceptible to disturbance from introduced ungulate species (Freeland 1990; Ash and McIvor 1998), because they did not evolve with native ungulates and have been devoid of large herbivores (>100 kg) since the extinction of the megafauna ~45 000 years ago (Skarpe 1991; Scogings and Sankaran 2019). However, several ungulate species have been introduced to the region since the 1800s (Ridpath 1991), and pastoralism is now a major land use (Woinarski and Ash 2002; Holmes 2010). Pigs (Sus scrofa), cattle (Bos taurus, B. indicus) and Asian water buffalo (Bubalus bubalis) have established widespread feral populations in tropical savanna habitats (Mihailou and Massaro 2021), including in conservation reserves, indigenous protected areas and national parks (Bayliss and Yeomans 1989; Kakadu National Parks Board of Management 2016). In addition, domestic cattle in pastoral areas of northern Australia are typically allowed to roam freely across vast areas (average paddock size in the Barkly region is 364 km2; Bubb 2004) of ‘unimproved’ native savanna rangeland until mustering (Karfs and Trueman 2005; Walsh and Cowley 2011; Chilcott et al. 2020). Fenced boundaries between pastoral properties and protected land are rarely effective barriers to ungulates, due to the high cost of maintenance and monitoring for breaks (Hunt et al. 2014). Therefore, domestic cattle often make their way into national parks (at least until the next tenured muster), where they likely cause the same damage to the landscape as feral populations, making the distinction between the two more academic than ecological.
The pervasiveness of pastoralism and the difficulty of controlling ungulates in protected areas in northern Australia raises concern that refugia for native wildlife may not be as extensive as it appears. Over the last few decades, many native mammal and bird species have suffered sudden and severe declines in their distribution and abundance in northern Australia, including in protected areas such as Kakadu National Park (Woinarski 2000; Woinarski et al. 2001, 2011; Franklin et al. 2005). These declines are often attributed to predation by feral cats (Felis catus) and the impacts of altered fire-regimes on native habitat (Pardon et al. 2003; Firth et al. 2010; Fisher et al. 2014; Lawes et al. 2015). However, researchers are increasingly considering the impacts of introduced ungulate species on vegetation communities as a factor that exacerbates the combined effect of both cats and fire on native wildlife (Legge et al. 2011, 2019; McGregor et al. 2014; Woinarski 2015). In addition, recent research has highlighted the potential competitive impacts of introduced ungulates on native herbivores in Australia, primarily due to dietary overlap and potential exclusion from resources (Reid et al. 2020a, 2020b, 2023; Mihailou et al. 2022).
Most ungulates, particularly grazing species, need to drink regularly due to their dry diets, so they are often distributed near waterpoints (Tomkins and O’Reagain 2007; Graz et al. 2012). Ungulate visitation to waterholes can reduce water quality through faecal contamination and by increasing turbidity through the overturning of benthic soils (Skeat et al. 1996; Finlayson et al. 1999; Pettit et al. 2012; Waltham and Schaffer 2017), reducing the suitability of waterholes for native wildlife. Additionally, ungulates can drink up to 10% of their body weight daily (Hunt et al. 2013; Bray et al. 2015) and can increase the evaporative potential of waterholes through pugging and wallowing (Skeat et al. 1996), directly reducing the amount of water available for native wildlife. In northern Australia, rainfall can vary greatly between years, and surface water availability declines as the dry season progresses each year, resulting in interannual (i.e. during years of below-average rainfall) and seasonal (i.e. as the dry season progresses each year) periods of water scarcity (Cook and Heerdegen 2001; Kanniah et al. 2013). Such periods concentrate animal activity around those areas where surface water is still available (Pettit et al. 2012). Both permanent and ephemeral waterholes provide important drought and dry season refugia for many savanna species (Thrash et al. 1995; Redfern 2002; Valeix 2011). Thus, ungulate visitation and use of savanna waterholes may have greater impacts on native biota when water scarcity is high compared with times when water is more readily available across the landscape, especially considering that some feral ungulates move in larger group sizes towards the end of the dry season (Reid et al. 2020b). Despite this, there is a lack of research in Australia on the behaviour of ungulates in the wild and whether water scarcity affects how they use waterholes in savanna environments. Understanding how feral ungulates respond to water scarcity may inform land managers and conservationists about potential threats to native biota due to changes in ungulate disturbance and allow targeted control and management of ungulates in protected areas.
In this study, we used remote-triggered wildlife cameras to investigate changes in the annual and seasonal visitation behaviours of three feral ungulate species (pigs, cattle and buffalo) at ephemeral savanna waterholes in Limmen National Park in northern Australia. Rainfall varied considerably over the 2 years the study was conducted. The first year received a relatively normal amount of rainfall, but the second year was a drought year with well below average rainfall, so surface water availability within the landscape varied considerably between years, as well as over the progression of the two dry seasons (i.e. with increasing time since last rainfall each year). We hypothesised that ungulates would respond to increasing water scarcity (both during the drought and as the dry season progressed) in the following ways: (1) visiting waterholes more frequently; (2) visiting waterholes for longer time periods; (3) visiting waterholes in larger numbers; and (4) spending more time drinking, foraging and wallowing at waterholes.
Materials and methods
Study area
This study was conducted in Limmen National Park, a large savanna reserve (>1 000 000 ha) in the Northern Territory, Australia. Vegetation communities in the region comprise a mosaic of grasses, shrubs and trees in a range of densities depending on fire history, soil type and landscape hydrology (Woinarski et al. 2007). Dominant flora species in savanna lowland areas of the park include native perennial grasses such as Chrysopogon fallax, C. latifolia, Eulalia aurea and Eriachne obtuse, and trees such as Eucalyptus microtheca, Bauhinia cunninghamii and Atalaya hemiglauca (Vincent and Mihailou 2023). Rainfall follows a monsoonal wet–dry cycle, whereby rain predominately falls between November and April (wet season), and the months of May to October (dry season) receive little or no rain. Rainfall can be highly variable between years (Taylor and Tulloch 1985; Haynes et al. 1991), so surface water availability can be restricted on both a seasonal (i.e. as the dry season progresses each year) and interannual basis (i.e. during years of below-average rainfall). On average, the park receives 859 mm of rain annually (as measured at Nathan River Ranger Station; Bureau of Meteorology 2021). The park received totals of 970 mm and 371 mm of rain over the 2017/2018 (hereafter 2018) and 2018/2019 (hereafter 2019) wet seasons respectively (Bureau of Meteorology 2021).
Data collection
Ephemeral waterholes within the park are typically small clay basins, which dry completely by the end of the dry season and are refilled by rain each wet season. To collect pig, cattle and buffalo visitation data, we selected 10 main waterholes of comparable size, depth, soil type and surrounding vegetation. Because pilot sampling in 2017 indicated pig numbers within the study area were lower than the other ungulates studied, we selected a further 10 sites for pig sampling only (following the same selection criteria). All waterholes were situated in open, mixed-savanna woodland. Those at the base of ridgelines or within 1 km of rivers and permanent billabongs were excluded.
Field work was conducted from April–October in 2018 and March–August in 2019. The length of the field season varied between these years due to the accelerated drying of waterholes during the drought (2019). Because data was collected from multiple sites per day, the total number of sampling days also varied between years and for different species (Table 1). All species were sampled for a total of 173 calendar days in 2018, and in 2019, cattle and buffalo were sampled for 138 calendar days, and pigs for 150. The duration of sampling in 2019 and the number of sampling days per year was higher for pigs than for cattle and buffalo because pigs were sampled over 20 sites instead of 10 sites. Four study sites were sampled each week on a 5-week rotation over the course of each dry season. Accessibility to sites at the start of the dry season determined their sampling order, which was maintained thereafter. To sample ungulate visitation to and use of study waterholes, three Reconyx Hyperfire HC600 motion-triggered wildlife cameras (‘camera traps’) were mounted to trees around waterholes at ~1.2 m above ground level and positioned to capture as much of the waterline as possible (see Fig. 1 for photo capture examples showing each ungulate species utilising study waterholes at various times of season and year). When triggered by movement or body heat, cameras captured three photos at 1-s intervals (key settings used: trigger, sensitive; night mode, max range; quiet period, no delay). Once set, cameras were left to sample waterhole visitation for ~5 continuous day/night cycles (i.e. average camera deployment = 5.17 days).
Variable | ||||||||||
---|---|---|---|---|---|---|---|---|---|---|
Species | Factor | Number of sampling days | Number of visits | Duration of visits (min) | Number of individuals | Time spent drinking (min) | Time spent foraging (min) | Time spent wallowing (min) | ||
Pig | Year | 2018 | 446 | 0.036 ± 0.257 (16) | 0.117 ± 1.342 (52) | 0.148 ± 1.339 (66) | – | 0.061 ± 0.628 (27) | – | |
2019 | 294 | 0.133 ± 0.467 (39) | 1.415 ± 8.889 (416) | 0.643 ± 3.105 (189) | – | 0.503 ± 3.133 (148) | – | |||
Dry season progression | Overall | 740 | 0.074 ± 0.358 (55) | 0.632 ± 5.729 (468) | 0.345 ± 2.228 (255) | – | 0.237 ± 2.043 (175) | – | ||
Cow | Year | 2018 | 226 | 0.159 ± 0.444 (36) | 1.580 ± 8.692 (357) | 0.482 ± 1.797 (109) | 0.181 ± 0.782 (41) | 0.637 ± 5.311 (144) | – | |
2019 | 168 | 0.512 ± 0.997 (86) | 6.655 ± 26.460 (1118) | 3.083 ± 7.988 (518) | 1.077 ± 3.682 (181) | 1.976 ± 8.967 (332) | – | |||
Dry season progression | Overall | 394 | 0.310 ± 0.752 (122) | 3.744 ± 18.630 (1475) | 1.591 ± 5.533 (627) | 0.564 ± 2.512 (222) | 1.208 ± 7.124 (476) | – | ||
Buffalo | Year | 2018 | 226 | 0.297 ± 0.664 (67) | 2.301 ± 8.460 (520) | 0.836 ± 2.524 (189) | 0.208 ± 1.329 (47) | 1.407 ± 6.509 (318) | 0.133 ± 0.952 (30) | |
2019 | 168 | 0.476 ± 0.868 (80) | 3.833 ± 14.420 (644) | 1.345 ± 3.521 (226) | 0.583 ± 2.260 (98) | 1.423 ± 5.166 (239) | 0.506 ± 2.445 (85) | |||
Dry season progression | Overall | 394 | 0.373 ± 0.762 (147) | 2.954 ± 11.400 (1164) | 1.053 ± 2.996 (415) | 0.368 ± 1.793 (145) | 1.414 ± 5.966 (557) | 0.292 ± 1.759 (115) |
Values in parentheses represent raw totals (over all sampling days). Dashes denote variables not included in analysis.
Collation and processing of data
Photos captured by cameras were processed using the database program CPW Photo Warehouse ver. 4.3.0.5 (Newkirk 2016). A visit was defined as any event where target species were caught on camera. A visit began when a camera was triggered by an ungulate and ended when the last individual of that species left the camera field of view. Multiple visits within a 30-min time frame were not considered to be independent events, unless the animals involved could be identified as different individuals/groups that appeared to be moving independently from each other within the 30-min time frame. For example, if a mixed group of female buffalo and calves entered the trigger zone from the right and began grazing for several minutes before eventually moving off to the left, and then 25 min later, a male buffalo entered from the right before exiting to the right – this series of triggers would be considered two distinct visits. The total number of individuals in a visit was determined by looking at all cameras stationed around a waterhole and counting the lowest possible number of individuals present, based on their identifiable markings (e.g. coat colour, horn/tusk shape, gender, size).
The behaviour of ungulates during visits was then classified into several categories, including drinking, foraging (either grazing or grubbing with head down posture moving slowly over fodder) and wallowing (for buffalo only). Other behaviours, such as moving through sites, resting (other than wallowing) and fighting were not included in the analysis. To account for slight variations in camera times and to prevent double counting of behaviours, a time buffer of 3 min was applied when multiple cameras stationed around a waterhole captured activity within a single visit. This buffer was determined by turning on all 20 cameras used for sampling at once and observing the maximum and minimum times displayed, then calculating the offset required so that no time overlap was possible. Where multiple cameras simultaneously captured activity in a visit and the 3-min buffer had to be applied, the configuration of cameras that captured the most activity was used.
Data were analysed at a daily scale per site (i.e. per sampling day). Visitation response variables included the number of visits, the total duration of visits and the total number of individuals visiting per sampling day. Behavioural response variables included time spent foraging (i.e. grazing in cattle and buffalo, grubbing in pigs), drinking (cattle and buffalo) and wallowing (buffalo). Although pigs were also observed drinking and wallowing, there were not enough records to allow analyses of these behaviours. The estimated time (in minutes) spent conducting a given behaviour in each sampling day was calculated as a function of camera triggers by dividing the number of camera triggers in which a given behaviour was observed by the total number of camera triggers, and then multiplying this by the total duration (in minutes) of all visits in that sampling day.
Data analyses
We used generalised linear mixed models (GLMMs) to analyse all data because they are well suited for modelling count data correlated by repeated sampling of study sites (Bolker et al. 2009; Bolker 2015). GLMMs modelled each response variable against two fixed factors (predictor variables): year, and days since the dry season started (i.e. year + days since the dry season started), as well as the interaction between the two (i.e. year × days since the dry season started). Days since the dry season started was included as a continuous variable. Study site was included as a random effect to account for repeated sampling. Given that much of the data was over-dispersed and zero-inflated (because there were many days when species did not visit waterholes), we tested the suitability of the two model variations for each response variable that included different model families (e.g. poisson, quasi-poisson, negative binomial, hurdle) and several zero-inflation factors (including year, days since the dry season started, year + days since the dry season started and year × days since the dry season started). The most parsimonious model was then determined using Akaike’s information criterion (AIC; Akaike 1973). If more than one model was supported (i.e. ΔAIC ≤ 2, Akaike weight of most parsimonious model <0.9), we produced a model that included all the variables (and their interactions) contained in the set of supported models (following Haslem et al. 2015). Full model component details and AIC values for the most parsimonious model for each response variable are presented in Supplementary Table S1. Results for co-efficients are presented for the conditional models using a quasi-poisson or negative binomial family with a log-link, and zero-inflation models used a logit-link. All data were analysed using the statistical program ‘R’ (R Core Team 2020), with library ‘glmmTMB’ (Brooks et al. 2017). Model dispersion was assessed using the ‘testDispersion’ and ‘simulateResiduals’ functions from the ‘DHARMa’ package (Hartig 2020). Figures were generated by using models to calculate predicted values for each response variable using the ‘ggpredict’ function from library ‘ggeffects’ (Lüdecke 2018) and plotted with ‘ggplot2’ (Wickham 2016).
Results
Number of visits
Both cattle and buffalo visited waterholes more frequently during the drought (2019) compared with the non-drought year (Table 2, Fig. 2a). Cattle also significantly increased their number of visits to waterholes as the dry season progressed, whereas buffalo did not. For pigs, the interaction between year and dry season progression was significant and positive − they visited waterholes more frequently towards the end of the dry season during the drought year (for full results, see Table S2).
Variable co-efficients | |||||||||
---|---|---|---|---|---|---|---|---|---|
Species | Model component | Factor | Number of visits | Duration of visits (min) | Number of individuals | Time spent drinking (min) | Time spent foraging (min) | Time spent wallowing (min) | |
Pig | Conditional | Year | −0.683 | −1.696 | −3.145* | – | −0.981 | – | |
Dry season progression | −0.100 | −0.0002 | −0.005 | – | 0.015 | – | |||
Interaction | 0.028** | 0.069** | 0.072*** | – | 0.060* | – | |||
Zero-inflation | Dry season progression | – | 0.261 | 0.245 | – | 0.334 | – | ||
Cow | Conditional | Year | 1.068*** | 1.319*** | 1.565*** | 3.578** | 1.316** | – | |
Dry season progression | 0.006* | 0.018*** | 0.018*** | 0.041*** | 0.012* | – | |||
Interaction | – | – | – | −0.019 | – | – | |||
Zero-inflation | Dry season progression | – | 0.022 | 0.014 | 0.115 | – | – | ||
Buffalo | Conditional | Year | 0.426* | 0.401* | 0.432* | 0.809* | 0.388 | 1.044** | |
Dry season progression | −0.001 | −0.001 | −0.001 | 0.004 | −0.005 | −0.001 |
All results represent values per sampling day sampled for different species (e.g. time spent drinking (minutes)/sampling day). Both conditional and zero-inflation components of models are presented where applicable. Significant values are presented in bold and significance levels are as follows: *** (P < 0.001), ** (P < 0.01), * (P < 0.05). Dashes denote factors not included in models. ‘Interaction’ denotes the interaction between year and dry season progression.
Effects of year and dry season progression on the waterhole visitation behaviours of feral ungulate species. (a) Number of visits by feral ungulate species per sampling day. (b) Duration of feral ungulate visits per sampling day (min). (c) Number of visiting individuals per sampling day. Solid lines represent predicted mean values and shaded areas represent upper and lower 95% confidence intervals. Colours of lines and shaded areas correspond to different years: blue (2018 – normal rainfall year) and red (2019 – drought year).
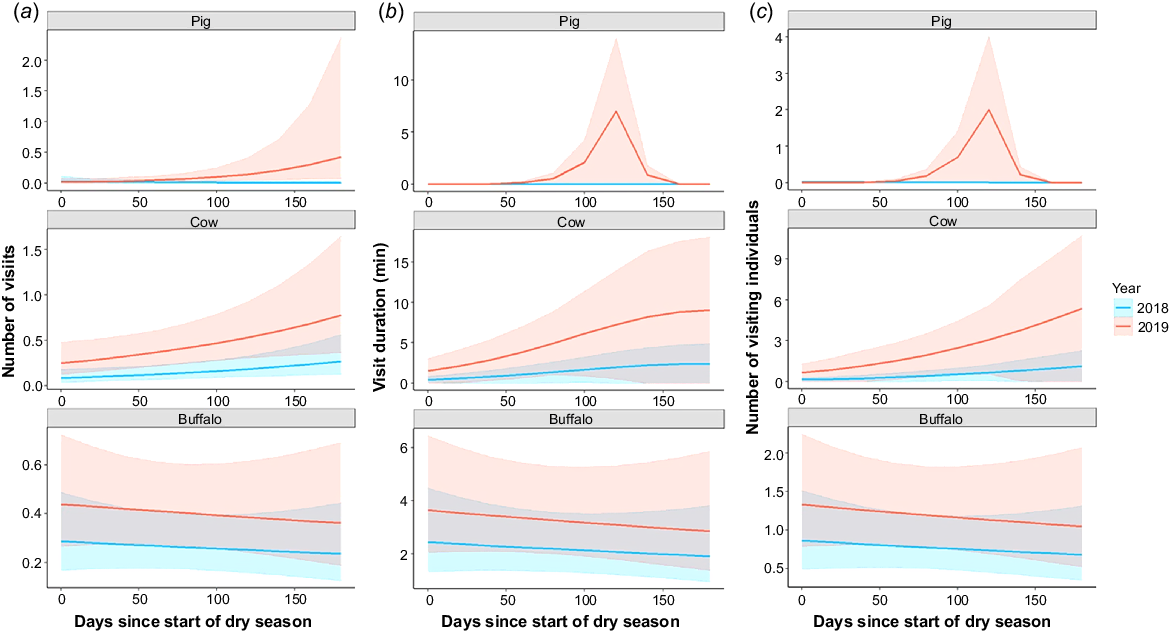
Visit duration
The duration of cattle and buffalo visits to waterholes increased during the drought (2019) compared with the non-drought year (Table 2, Fig. 2b). In addition, cattle significantly increased the duration of their visits as the dry season progressed, whereas buffalo did not. For pigs, the interaction between year and dry season progression was significant and positive − the durations of their visits increased towards the end of the dry season during the drought year. Dry season progression was included as a zero-inflation factor in the pig model but was not significant (for full results, see Table S3).
Number of individuals visiting
The numbers of cattle and buffalo visiting waterholes increased significantly during the drought year (Table 2, Fig. 2c). Cattle numbers also increased significantly as the dry season progressed, but buffalo numbers were not significantly affected. For pigs, the interaction between year and dry season progression was significant and positive − their numbers increased towards the end of the dry season during the drought year. Dry season progression was included as a zero-inflation factor in the pig model but was not significant (for full results, see Table S4).
Time spent foraging
Cattle spent significantly more time foraging around waterhole verges during the drought and as the dry season progressed, but buffalo did not alter their behaviour in response to either factor (Table 2, Fig. 3a). For pigs, the interaction between year and dry season progression was significant and positive − they spent more time foraging towards the end of the dry season during the drought year. Dry season progression was included as a zero-inflation factor in the pig model but was not significant (for full results, see Table S5).
Effect of year and dry season progression on the waterhole use behaviours of feral ungulates. (a) Time feral ungulate species spent foraging around waterholes per sampling day (min). (b) Time cattle and buffalo spent drinking at waterholes per sampling day (min). (c) Time buffalo spent wallowing per sampling day (min). Solid lines represent predicted mean values and shaded areas represent upper and lower 95% confidence intervals. Colours of lines and shaded areas correspond to different years: blue (2018 – normal rainfall year) and red (2019 – drought year).
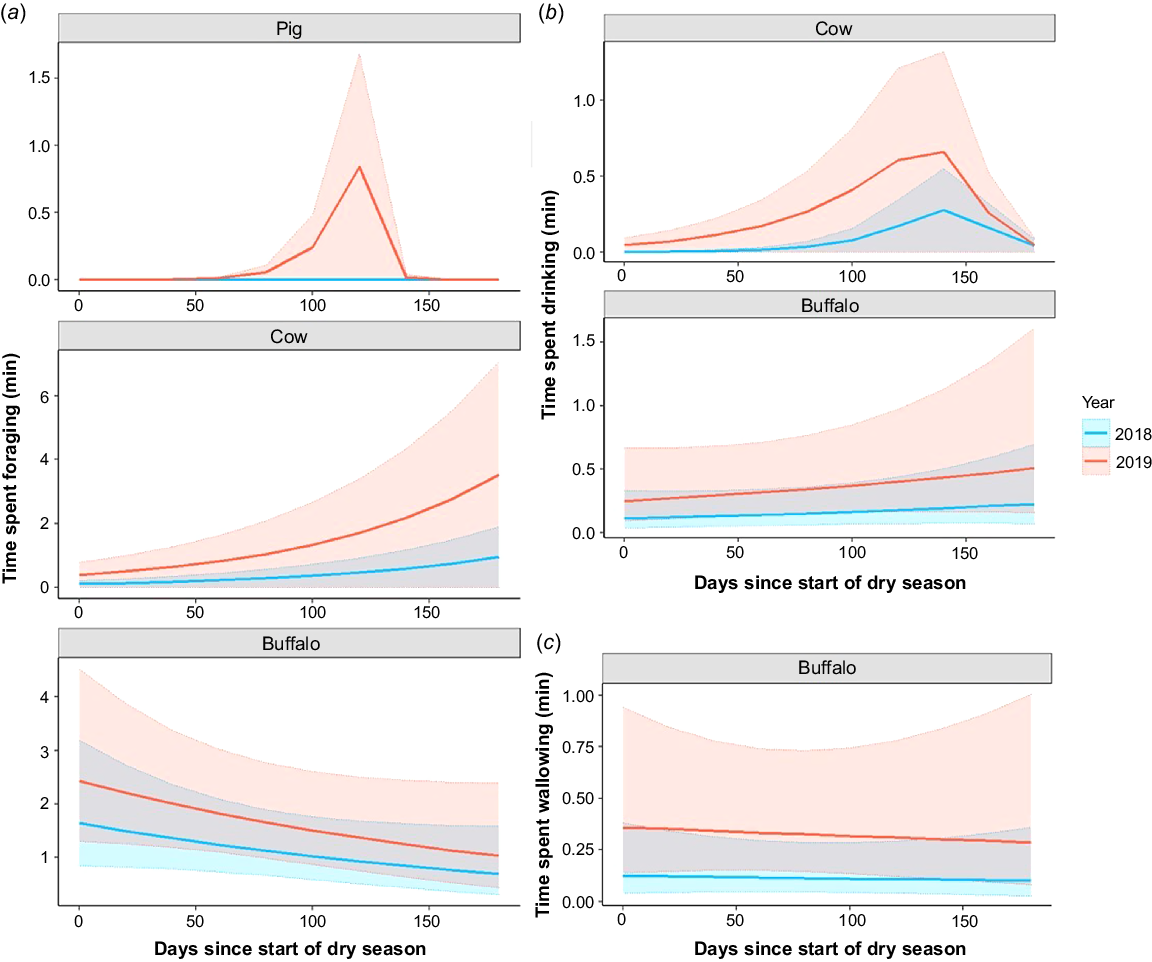
Time spent drinking
The time cattle and buffalo spent drinking at waterholes increased significantly during the drought year (Table 2, Fig. 3b). Cattle also spent significantly more time drinking as the dry season progressed, but buffalo did not. Model selection supported the inclusion of an interaction term between year and dry season progression for the cattle model, but this result was not significant (i.e. the time cattle spent drinking at the end of the dry season was not significantly different between years; for full results, see Table S6).
Discussion
Responses to drought
In this study, we found that water scarcity exacerbated feral ungulate use of ephemeral waterholes in Limmen National Park. All ungulates increased their number of visits to waterholes, visited for longer durations and visited in greater numbers during the drought year. We also found that the behaviours ungulates exhibited during visits were significantly affected by drought: cattle and buffalo spent more time drinking, pigs and cattle spent more time foraging and buffalo spent more time wallowing during the drought year. Our findings support the hypothesis of Illius and O’Connor (2000) that seasonal and climactic variability leads to the spatial concentration of ungulates around key resource areas, such as waterholes.
High waterhole use by ungulates can lead to severe defoliation of vegetation communities in a spherical gradient surrounding waterpoints, known as a piosphere (Thrash and Derry 1999; Illius and O’Connor 2000). Our findings suggest that drought may exacerbate ungulate impacts on vegetation communities surrounding savanna waterholes. Piospheres have been shown to have long lasting (>100 years) and possibly irreversible effects on vegetation and soil characteristics in Australian arid areas, even after grazing pressure is removed (Croft et al. 2007). Accordingly, further research defining how feral ungulates affect vegetation communities surrounding Australian savanna waterholes during drought, and how this may impact native fauna communities, is needed. Additionally, studies over longer time periods of fluctuating water availability (>2 years) are needed to verify our findings.
Responses to dry season progression
Dry season progression affected waterhole visitation and behaviour of cattle, pigs and buffalo differently. Cattle were the most responsive to water scarcity: they significantly increased their number of visits, they visited for longer durations and more individuals visited per sampling day as the dry season progressed. They also spent significantly more time drinking and foraging when visiting waterholes as the dry season progressed. Cattle were the most numerous feral ungulates observed during the study (Table 1) and were detected 4.6 and 1.5 times more per sampling day than pigs and buffalo, respectively. The number of cattle we detected across just 10 small waterholes is concerning, because each adult can drink ~40–80 L of water daily depending on sex and body condition (Hunt et al. 2013; Bray et al. 2015). Therefore, increased cattle visitation to savanna waterholes during dry and hot conditions (i.e. drought, end of dry season) may deplete already scarce water resources available to native fauna. In addition, cattle presence may affect patterns of waterhole visitation by native fauna (Mihailou et al. 2022), as has been observed with other feral ungulate species in water limited habitats (e.g. Perry et al. 2015; Hall et al. 2016; Gooch et al. 2017; Brim Box et al. 2019). For example, feral camel presence at waterholes in central Australia reduces the frequency of visits by native dingoes (Canis lupus dingo) and birds and impacts their temporal activity patterns (Brim Box et al. 2019).
Pigs only changed their visitation and behaviours at waterholes at the end of the dry season during the drought, when water scarcity was most extreme. They visited waterholes more, for longer periods and in greater numbers, and spent more time foraging at the end of the dry season during the drought than at any other times. This suggests that pigs are more reliant on larger waterbodies (e.g. lagoons, swamps and billabongs) than cattle and buffalo − they provide better foraging opportunities for pigs than the small, ephemeral waterholes studied here (Ridpath 1991). Lower surface water availability during the drought may have led pigs to move into less preferred habitat as the year progressed and many of the larger billabongs in the study area prematurely dried out. This also explains why pigs spent more time foraging around our 20 focal waterholes as the drought progressed, because larger waterbodies may have dried earlier than in the previous year (i.e. did not fill to capacity), forcing pigs to find alternative foraging sites.
Buffalo only changed their waterhole visitation and use in response to drought, and not dry season progression. This may be because buffalo evolved in hot, humid wetland habitats (Ridpath 1991) where evaporative cooling from sweating is impaired. Buffalo have highly vascularised skin and rely on frequent wallowing to keep cool (Tulloch and Litchfield 1981; Ridpath 1991), and their water requirements appear to be high year-round. This may explain why their visitation and use of waterholes was relatively consistent across the dry season. By contrast, drought may have had greater impacts on buffalo behaviour because reduced surface water availability would have increased their dependence on those waterholes still containing water (e.g. our study waterholes). Unlike cattle, buffalo did not spend more time grazing riparian vegetation on waterhole verges with increasing water scarcity. This may be explained by the wider dietary niche of buffalo, whose diet consists of <30% grasses (Bowman et al. 2010), compared with ~50% grasses for cattle (Reid et al. 2020a). Unlike cattle, buffalo may selectively browse more woody vegetation with higher moisture content than desiccated grasses in the wider savanna as water scarcity increases (Reid et al. 2020a), limiting their reliance on riparian food resources.
Broader implications of feral ungulate aggregations at ephemeral waterholes
Feral ungulates have extensive impacts on Australian savanna habitats and cause substantial detrimental flow-on effects for native wildlife, particularly the destruction or degradation of primary habitat (Mihailou and Massaro 2021). Our study indicates that water scarcity increases ungulate presence and activity at savanna waterholes, and that these areas are particularly vulnerable to ungulate disturbance. Negative flow-on effects of this disturbance for wildlife are potentially worsened at critical times, when water sources are limited. In a related study, we found that waterhole visitation by native macropods (Family Macropodidae, including kangaroos and wallabies) increased during periods of water scarcity, but their activity rapidly declined as cattle visitation peaked at the end of the dry season (Mihailou et al. 2022). This finding that feral ungulate presence at waterholes may deter visitation by native fauna is supported by other studies in Australia (cattle: Mihailou et al. 2022; Reid et al. 2020a, camels: Brim Box et al. 2019) and North America (horses: Ostermann-Kelm et al. 2008; Hall et al. 2018). Vulnerable fauna already suffering declines in Australia’s northern savannas, such as small mammals (Woinarski et al. 2011; Woinarski 2015) and granivorous birds (Franklin 1999; Franklin et al. 2005), may also be negatively affected by ungulate disturbance around waterholes during water scarcity events. Ungulate damage to understorey vegetation surrounding waterholes may be particularly detrimental for small species with frequent drinking requirements (e.g. granivores), because habitat simplification around watering points increases vulnerability of these species to predators, such as feral cats (Fisher et al. 2014; McGregor et al. 2014; Stobo-Wilson et al. 2020).
Our findings suggest that management and control programs for feral ungulates in northern savannas may benefit from targeting different species under different water scarcity conditions. To make the most out of aerial and ground-based control operations, cattle should be targeted around waterholes in the late dry season, irrespective of annual rainfall, because this is when their activity is most concentrated around waterpoints. By contrast, pigs are best targeted (at least around ephemeral water sources in savanna) during extreme water scarcity events (i.e. late dry season during drought years). Because buffalo utilise waterholes consistently across the dry season, land managers should instead focus control programs during years of low rainfall.
Climate change is predicted to reduce surface water availability in northern Australia (Dai et al. 2018; NESP Earth Systems and Climate Change Hub 2020), so increasing feral ungulate use of waterholes may exacerbate stress on native flora and fauna communities. This could lead to the loss of resilience of ecosystems to recover from severe water scarcity events and potentially lead to irreversible damage to sensitive habitats. Therefore, more research is warranted to assess how different densities of feral ungulates impact native species. Furthermore, the social and economic value of ungulates complicate conservation efforts in the region (Robinson et al. 2005; Spear and Chown 2009; Sloane et al. 2021). Many local stakeholders view feral ungulates as a source of income (e.g. tourism operators and safari hunters – buffalo), or as a self-sustaining food source (e.g. recreational hunters – pigs and buffalo; some traditional Aboriginal landowners – cattle and buffalo), rather than a threat to native ecosystems (Skeat et al. 1996; Robinson et al. 2005; Ens et al. 2016). Further research is needed to assess the impacts of feral ungulate populations on native flora and fauna in northern Australia, especially in national parks and conservation areas intended to provide protection for native species.
Declaration of funding
This work was supported by the Hermon Slade Foundation (HSF 17/1), the Ecological Society of Australia (Holsworth Wildlife Research Endowment, 0000102503), Birdlife Australia (2017 Stuart Leslie Bird Research Award) and the former Institute for Land, Water and Society at Charles Sturt University. Helenna Mihailou was also funded by an Australian Government Research Training Program scholarship (Charles Sturt University).
Code availability
The modelling used for the statistical analysis is described in detail in the text and supplementary material, including the ‘R’ packages used.
Author contributions
All authors conceived and designed the study: Melanie Massaro and Helenna Mihailou secured funding for the project; Helenna Mihailou conducted the field data collection and collation; Helenna Mihailou and Dale Nimmo conducted the statistical analysis; Helenna Mihailou and Melanie Massaro drafted the manuscript; and all authors contributed to the review and editing of the final manuscript.
Acknowledgements
The authors thank the Hermon Slade Foundation, the Ecological Society of Australia, Birdlife Australia and the Institute for Land, Water and Society (Charles Sturt University) for their funding of the project that led to this manuscript. We also thank the Parks and Wildlife Commission of the Northern Territory for their support of this study. Finally, we thank the reviewers and editors for their helpful comments to improve the manuscript. This paper forms part of the PhD thesis of Helenna Mihailou (2022).
References
Ash AJ, McIvor JG (1998) How season of grazing and herbivore selectivity influence monsoon tall-grass communities of northern Australia. Journal of Vegetation Science 9, 123-132.
| Crossref | Google Scholar |
Bayliss P, Yeomans KM (1989) Distribution and abundance of feral livestock in the ‘Top End’ of the Northern Territory (1985-86), and their relation to population control. Wildlife Research 16, 651-676.
| Crossref | Google Scholar |
Bolker BM, Brooks ME, Clark CJ, Geange SW, Poulsen JR, Stevens MHH, White J-SS (2009) Generalized linear mixed models: a practical guide for ecology and evolution. Trends in Ecology & Evolution 24, 127-135.
| Crossref | Google Scholar | PubMed |
Bowman DMJS, Murphy BP, McMahon CR (2010) Using carbon isotope analysis of the diet of two introduced Australian megaherbivores to understand Pleistocene megafaunal extinctions. Journal of Biogeography 37, 499-505.
| Crossref | Google Scholar |
Bray S, Walsh D, Hoffmann M, Henry B, Eady S, Collier C, Pettit C, Navarro J, Corbet D (2015) Desktop research project to provide data on liveweight and liveweight gain in the beef cattle sector in Queensland and the Northern Territory. Department of Agriculture and Fisheries, Rockhampton, QLD, Australia.
Brim Box J, Bledsoe L, Box P, Bubb A, Campbell M, Edwards G, Fordyce JD, Guest T, Hodgens P, Kennedy B, Kulitja R, McConnell K, McDonald PJ, Miller B, Mitchell D, Nano C, O’Dea D, Richmond L, Stricker AC, Caron V (2019) The impact of camel visitation on native wildlife at remote waterholes in arid Australia. Journal of Zoology 309, 84-93.
| Crossref | Google Scholar |
Brooks ME, Kristense K, van Benthem KJ, Magnusson A, Berg CW, Nielsen A, Skaug HJ, Mächler M, Bolker BM (2017) glmmTMB balances speed and flexibility among packages for zero-inflated generalized linear mixed modeling. The R Journal 9, 378-400.
| Crossref | Google Scholar |
Bureau of Meteorology (2021) Climate data online. Available at http://www.bom.gov.au/climate/data/index.shtml
Cook GD, Heerdegen RG (2001) Spatial variation in the duration of the rainy season in monsoonal Australia. International Journal of Climatology 21, 1723-1732.
| Crossref | Google Scholar |
Dai A, Zhao T, Chen J (2018) Climate change and drought: a precipitation and evaporation perspective. Current Climate Change Reports 4, 301-312.
| Crossref | Google Scholar |
Ens EJ, Daniels C, Nelson E, Roy J, Dixon P (2016) Creating multi-functional landscapes: using exclusion fences to frame feral ungulate management preferences in remote aboriginal-owned northern Australia. Biological Conservation 197, 235-246.
| Crossref | Google Scholar |
Firth RSC, Brook BW, Woinarski JCZ, Fordham DA (2010) Decline and likely extinction of a northern Australian native rodent, the Brush-tailed Rabbit-rat Conilurus penicillatus. Biological Conservation 143, 1193-1201.
| Crossref | Google Scholar |
Fisher DO, Johnson CN, Lawes MJ, Fritz SA, McCallum H, Blomberg SP, VanDerWal J, Abbott B, Frank A, Legge S, Letnic M, Thomas CR, Fisher A, Gordon IJ, Kutt A (2014) The current decline of tropical marsupials in Australia: is history repeating? Global Ecology and Biogeography 23, 181-190.
| Crossref | Google Scholar |
Franklin DC (1999) Evidence of disarray amongst granivorous bird assemblages in the savannas of northern Australia, a region of sparse human settlement. Biological Conservation 90, 53-68.
| Crossref | Google Scholar |
Franklin DC, Whitehead PJ, Pardon G, Matthews J, McMahon P, McIntyre D (2005) Geographic patterns and correlates of the decline of granivorous birds in northern Australia. Wildlife Research 32, 399-408.
| Crossref | Google Scholar |
Freeland WJ (1990) Large herbivorous mammals: exotic species in northern Australia. Journal of Biogeography 17, 445-449.
| Crossref | Google Scholar |
Gooch AMJ, Petersen SL, Collins GH, Smith TS, McMillan BR, Eggett DL (2017) The impact of feral horses on pronghorn behavior at water sources. Journal of Arid Environments 138, 38-43.
| Crossref | Google Scholar |
Graz FP, Westbrooke ME, Florentine SK (2012) Modelling the effects of water-point closure and fencing removal: a GIS approach. Journal of Environmental Management 104, 186-194.
| Crossref | Google Scholar | PubMed |
Hall LK, Larsen RT, Westover MD, Day CC, Knight RN, McMillan BR (2016) Influence of exotic horses on the use of water by communities of native wildlife in a semi-arid environment. Journal of Arid Environments 127, 100-105.
| Crossref | Google Scholar |
Hall LK, Larsen RT, Knight RN, McMillan BR (2018) Feral horses influence both spatial and temporal patterns of water use by native ungulates in a semi-arid environment. Ecosphere 9, e02096.
| Crossref | Google Scholar |
Hartig F (2020) DHARMa: residual diagnostics for hierarchical (multi-level/mixed) regression models. Available at https://CRAN.R-project.org/package=DHARMa
Haslem A, Nimmo DG, Radford JQ, Bennett AF (2015) Landscape properties mediate the homogenization of bird assemblages during climatic extremes. Ecology 96, 3165-3174.
| Crossref | Google Scholar |
Holmes J (2010) The multifunctional transition in Australia’s tropical savannas: the emergence of consumption, protection and indigenous values. Geographical Research 48, 265-280.
| Crossref | Google Scholar |
Hunt LP, McIvor JG, Grice AC, Bray SG (2014) Principles and guidelines for managing cattle grazing in the grazing lands of northern Australia: stocking rates, pasture resting, prescribed fire, paddock size and water points – a review. The Rangeland Journal 36, 105-119.
| Crossref | Google Scholar |
Illius AW, O’Connor TG (2000) Resource heterogeneity and ungulate population dynamics. Oikos 89, 283-294.
| Crossref | Google Scholar |
Kanniah KD, Beringer J, Hutley LB (2013) Response of savanna gross primary productivity to interannual variability in rainfall: results of a remote sensing based light use efficiency model. Progress in Physical Geography: Earth and Environment 37, 642-663.
| Crossref | Google Scholar |
Karfs RA, Trueman M (2005) Tracking changes in the Victoria River District Pastoral District, Northern Territory, Australia – 2005. Report to the Australian Collaborative Rangeland Information System (ACRIS) Management Committee. Department of Natural Resources, Environment and the Arts, Northern Territory Government, Darwin, NT, Australia.
Lawes MJ, Murphy BP, Fisher A, Woinarski JCZ, Edwards AC, Russell-Smith J (2015) Small mammals decline with increasing fire extent in northern Australia: evidence from long-term monitoring in Kakadu National Park. International Journal of Wildland Fire 24, 712-722.
| Crossref | Google Scholar |
Legge S, Kennedy MS, Lloyd R, Murphy SA, Fisher A (2011) Rapid recovery of mammal fauna in the central Kimberley, northern Australia, following the removal of introduced herbivores. Austral Ecology 36, 791-799.
| Crossref | Google Scholar |
Legge S, Smith JG, James A, Tuft KD, Webb T, Woinarski JCZ (2019) Interactions among threats affect conservation management outcomes: livestock grazing removes the benefits of fire management for small mammals in Australian tropical savannas. Conservation Science and Practice 1, e52.
| Crossref | Google Scholar |
Lüdecke D (2018) ggeffects: tidy data frames of marginal effects from regression models. The Journal of Open Source Software 3, 772.
| Crossref | Google Scholar |
McGregor HW, Legge S, Jones ME, Johnson CN (2014) Landscape management of fire and grazing regimes alters the fine-scale habitat utilisation by feral cats. PLoS ONE 9, e109097.
| Crossref | Google Scholar |
Mihailou H, Massaro M (2021) An overview of the impacts of feral cattle, water buffalo and pigs on the savannas, wetlands and biota of northern Australia. Austral Ecology 46, 699-712.
| Crossref | Google Scholar |
Mihailou H, Nimmo DG, Massaro M (2022) Feral ungulate and macropod responses to resource scarcity and predation risk at savanna waterholes. Behavioral Ecology and Sociobiology 76, 23.
| Crossref | Google Scholar |
Ostermann-Kelm S, Atwill ER, Rubin ES, Jorgensen MC, Boyce WM (2008) Interactions between feral horses and desert bighorn sheep at water. Journal of Mammalogy 89, 459-466.
| Crossref | Google Scholar |
Pardon LG, Brook BW, Griffiths AD, Braithwaite RW (2003) Determinants of survival for the northern brown bandicoot under a landscape-scale fire experiment. Journal of Animal Ecology 72, 106-115.
| Crossref | Google Scholar |
Perry ND, Morey P, Miguel GS (2015) Dominance of a natural water source by feral horses. The Southwestern Naturalist 60, 390-393.
| Crossref | Google Scholar |
Pettit NE, Jardine TD, Hamilton SK, Sinnamon V, Valdez D, Davies PM, Douglas MM, Bunn SE (2012) Seasonal changes in water quality and macrophytes and the impact of cattle on tropical floodplain waterholes. Marine and Freshwater Research 63, 788-800.
| Crossref | Google Scholar |
Reid AM, Murphy BP, Vigilante T, Wunambal Gaambera Aboriginal Corporation, Barry LA, Bowman DMJS (2020a) Carbon isotope analysis shows introduced bovines have broader dietary range than the largest native herbivores in an Australian tropical savanna. Austral Ecology 45, 109-121.
| Crossref | Google Scholar |
Reid AM, Murphy BP, Vigilante T, Wunambal Gaambera Aboriginal Corporation, Bowman DMJS (2020b) Distribution and abundance of large herbivores in a northern Australian tropical savanna: a multi-scale approach. Austral Ecology 45, 529-547.
| Crossref | Google Scholar |
Reid AM, Murphy BP, Vigilante T, Wunambal Gaambera Aboriginal Corporation, Bowman DMJS (2023) Pyric herbivory and the nexus between forage, fire and native and introduced large grazing herbivores in Australian tropical savanna. Ecosystems 26, 610-626.
| Crossref | Google Scholar |
Robinson CJ, Smyth D, Whitehead PJ (2005) Bush tucker, bush pets, and bush threats: cooperative management of feral animals in Australia’s Kakadu National Park. Conservation Biology 19, 1385-1391.
| Crossref | Google Scholar |
Skarpe C (1991) Impact of grazing in savanna ecosystems. Ambio 20, 351-356.
| Google Scholar |
Sloane DR, Ens E, Wunungmurra Y, Gumana Y, Wunungmurra B, Wirrpanda M, Towler G, Preece D, Rangers Y (2021) Lessons from old fenced plots: eco-cultural impacts of feral ungulates and potential decline in sea-level rise resilience of coastal floodplains in northern Australia. Ecological Management & Restoration 22, 191-203.
| Crossref | Google Scholar |
Spear D, Chown SL (2009) Non-indigenous ungulates as a threat to biodiversity. Journal of Zoology 279, 1-17.
| Crossref | Google Scholar |
Stobo-Wilson AM, Stokeld D, Einoder LD, Davies HF, Fisher A, Hill BM, Mahney T, Murphy BP, Stevens A, Woinarski JCZ, Rangers B, Rangers W, Gillespie GR (2020) Habitat structural complexity explains patterns of feral cat and dingo occurrence in monsoonal Australia. Diversity and Distributions 26, 832-842.
| Crossref | Google Scholar |
Taylor JA, Tulloch D (1985) Rainfall in the wet-dry tropics: extreme events at Darwin and similarities between years during the period 1870–1983 inclusive. Australian Journal of Ecology 10, 281-295.
| Crossref | Google Scholar |
Thrash I, Derry JF (1999) The nature and modelling of piospheres: a review. Koedoe 42, a234.
| Crossref | Google Scholar |
Thrash I, Theron GK, Bothma JdP (1995) Dry season herbivore densities around drinking troughs in the Kruger National Park. Journal of Arid Environments 29, 213-219.
| Crossref | Google Scholar |
Tomkins N, O’Reagain P (2007) Global positioning systems indicate landscape preferences of cattle in the subtropical savannas. The Rangeland Journal 29, 217-222.
| Crossref | Google Scholar |
Tulloch DG, Litchfield RT (1981) Wallows for buffalo. Wildlife Research 8, 555-565.
| Crossref | Google Scholar |
Valeix M (2011) Temporal dynamics of dry-season water-hole use by large African herbivores in two years of contrasting rainfall in Hwange National Park, Zimbabwe. Journal of Tropical Ecology 27, 163-170.
| Crossref | Google Scholar |
Vincent JD, Mihailou H (2023) Above-average rainfall stimulates short-term, passive recovery of ungulate mediated soil scalds in Australian mesic savanna. Rangeland Ecology & Management 86, 26-34.
| Crossref | Google Scholar |
Walsh D, Cowley RA (2011) Looking back in time: can safe pasture utilisation rates be determined using commercial paddock data in the Northern Territory? The Rangeland Journal 33, 131-42.
| Crossref | Google Scholar |
Woinarski JCZ (2000) The conservation status of rodents in the monsoonal tropics of the Northern Territory. Wildlife Research 27, 421-435.
| Crossref | Google Scholar |
Woinarski JCZ (2015) Critical-weight-range marsupials in northern Australia are declining: a commentary on Fisher et al. (2014) ‘The current decline of tropical marsupials in Australia: is history repeating?’. Global Ecology and Biogeography 24, 118-122.
| Crossref | Google Scholar |
Woinarski JCZ, Ash AJ (2002) Responses of vertebrates to pastoralism, military land use and landscape position in an Australian tropical savanna. Austral Ecology 27, 311-323.
| Crossref | Google Scholar |
Woinarski JCZ, Milne DJ, Wanganeen G (2001) Changes in mammal populations in relatively intact landscapes of Kakadu National Park, Northern Territory, Australia. Austral Ecology 26, 360-370.
| Crossref | Google Scholar |
Woinarski JCZ, Legge S, Fitzsimons JA, Traill BJ, Burbidge AA, Fisher A, Firth RSC, Gordon IJ, Griffiths AD, Johnson CN, McKenzie NL, Palmer C, Radford I, Rankmore B, Ritchie EG, Ward S, Ziembicki M (2011) The disappearing mammal fauna of northern Australia: context, cause, and response. Conservation Letters 4, 192-201.
| Crossref | Google Scholar |