Influence of combined hydric and thermal stresses on Rosmarinus officinalis and Cistus albidus
Rawaa Jamaladdeen A , Bruno Coudour A * , Fabienne Dédaldéchamp B , Laurent Lemée
A Institut P’ - UPR 3346 CNRS, ENSMA, University of Poitiers, Chasseneuil, France. Email: rawaa.jamaladdeen@ensma.fr, jean-pierre.garo@ensma.fr, hui-ying.wang@ensma.fr
B EBI - UMR CNRS 7267, Ecologie et Biologie des Interactions, University of Poitiers, Poitiers, France. Email: fabienne.dedaldechamp@univ-poitiers.fr
C IC2MP - UMR CNRS 7285, Institut de Chimie des Milieux et Matériaux de Poitiers, University of Poitiers, Poitiers Cedex, France. Email: laurent.lemee@univ-poitiers.fr
International Journal of Wildland Fire 32(6) 968-978 https://doi.org/10.1071/WF22146
Submitted: 6 July 2022 Accepted: 9 April 2023 Published: 9 May 2023
© 2023 The Author(s) (or their employer(s)). Published by CSIRO Publishing on behalf of IAWF. This is an open access article distributed under the Creative Commons Attribution-NonCommercial-NoDerivatives 4.0 International License (CC BY-NC-ND)
Abstract
Background: Wildfires are a growing threat, especially in Mediterranean climate areas during periods of drought. The wildfire research community continues to investigate propagation mechanisms considering thermal transfer and fluid mechanics and sometimes a simplified chemistry taking into account the main combustion products such as CO, CO2, H2O, H2 and CH4. However, volatile organic compounds (VOCs) cannot be neglected and have a major role in vegetation ignition. Despite this, knowledge on the influence of wildfire on plant emissions remains lacking.
Aims: This work addresses the effect of combined hydric and thermal stresses on the physiology and emissions of Rosmarinus officinalis and Cistus albidus, which are widely consumed in Mediterranean wildfires.
Methods: Plants were submitted to hydric stress inside a greenhouse, then to thermal stress inside a heated hermetic enclosure while collecting emissions with adsorbent elements for analysis. Simultaneously, heating experiments were carried out with leaves sampled to analyse their emissions.
Key results: The heating tests showed significant variations in monoterpene, fatty acid and alkane emissions between stressed and unstressed plants with different results between Rosmarinus officinalis and Cistus albidus.
Conclusion/implication: Further experiments with other plant species and quantification of emissions may help to better know why Mediterranean wildfires are a particular threat and how to improve firefighting methods.
Keywords: alkanes, chlorophyll, combined hydric and thermal stresses, fatty acids, hydrocarbons, leaf and plant emissions, microscopy, physiology analyses, plant heating, Py-GC-MS, radiant panel, Rosmarinus officinalis, Cistus albidus, solid-phase extraction elements (SPEE), TD-GC-MS, terpenes, thermodesorption, water content.
Context and objectives
Wildfires are a growing issue, especially in Mediterranean climate areas during periods of drought where more and more uncontrollable fires are observed, such as megafires, too large and strong to be confined, and eruptive fires, too fast and unpredictable to be fought. These last years have been particularly disastrous with megafires widely reported in the worldwide media (Silveira et al. 2022). As for eruptive fires, these are sudden and impressive wildfire accelerations generally occurring with moderate wind or even without wind. They have caused more than 150 fatalities in Europe and the USA so far (Carbonell et al. 2005; Viegas 2009; Chetehouna 2014; Chatelon et al. 2014; Coudour et al. 2016b; Lahaye 2018), mainly among firefighters involved in extinguishing operations.
According to Schneider et al. (2021) ‘there is a strong need to increase the basic understanding of the propagation mechanisms of wildfires to improve the scientific tools needed for firefighting and fire prevention’. Classical propagation of a wildfire is mainly due to two correlated mechanisms: large heat transfers (energy) and formation of flammable mixtures ahead of the fire front (gases). Heat is transmitted principally by thermal radiation and convection. Flammable mixtures are formed from the only available fuel (vegetation) while it is heated, pyrolysed and burnt. The wildfire research community continues to investigate propagation mechanisms of fire fronts such as thermal radiation (Frangieh et al. 2020) or buoyant flame dynamics (Finney et al. 2015). Research on eruptive fires is also focusing on airflow considerations (Viegas and Caballero 2009; Chatelon et al. 2014) such as induced wind, flame attachment and inclination angle. The mentioned studies on wildfire propagation take into account large-scale mechanisms such as thermal and fluid mechanics ones, or take into account the main chemical compounds resulting from degradation and combustion (CO, CO2, H2O, H2 and CH4). However, there is a lack of investigation on the role of plants (Silveira et al. 2022) and the large range of molecules involved in wildfire propagation at the fire front, given that Mediterranean plants are particularly rich in volatile essences (Owen et al. 2001).
During the day, vegetation emits O2, water, CO2 and also volatile essences (Owen et al. 2001). Volatile essences contain mainly isoprenoids (Ormeño et al. 2007; Lavoir 2010; Ciccioli et al. 2014; Courty et al. 2014), which are produced by biosynthesis (Nagegowda and Gupta 2020) and emitted essentially by plant leaves (Lavoir 2010). Emitted isoprenoids include isoprene (C5H8), monoterpenes (C10H16), sesquiterpenes (C15H24) and their oxides, e.g. verbenone (C10H14O). These are very flammable compounds (Courty et al. 2012; Rudz et al. 2014) and their density, up to seven times higher than air for sesquiterpenes (C15H24), favours their accumulation at ground level. For a given plant species, emissions strongly depend on the season, time of day, meteorological conditions, plant history, drought, light, etc. (Lavoir 2010).
Among different plant stresses (Lemoine et al. 2013), thermal and hydric stresses are the main ones affecting vegetation during wildfires. Research has been carried out to estimate the main gases emitted by vegetation; studies have been carried out in situ with ordinary temperatures (Owen et al. 2001) or in laboratory conditions for higher temperatures (Chetehouna 2014). Results show that emissions increase quantitatively when the vegetation is heated, with a maximum around 180°C (Barboni 2006; Chetehouna 2014; Courty et al. 2014) corresponding to the boiling point of the main monoterpenes. Plant emissions have also been studied under hydric stress with conflicting results depending on plants and methodologies (Lavoir 2010). Hydric stress affects many functioning parameters of plant emission zones such as leaf temperature, stomatal conductance, photosynthesis and leaf surface (Lemoine et al. 2013). With hydric stress, stomata generally close in order to limit transpiration, thus inducing a decrease in absorption of carbon dioxide from the air, which involves a decrease in photosynthesis, but as far as we know, no explanation has been found concerning its influence on isoprenoid biosynthesis. According to experimental protocols and studied plant species, an increase or a decrease in volatile organic compounds emitted by plants can be seen under hydric stress, e.g. a decrease in sesquiterpenes emissions for Rosmarinus officinalis and an increase for Cistus albidus (Ormeño et al. 2007). Isoprenoid content generally increases in plant leaves with hydric stress for conifers and aromatic plants (Llusià and Peñuelas et al. 1998), which makes them more flammable. Indeed, even if emissions do not increase, once exposed to a fire front, a plant will necessarily release its isoprenoid reserves owing to thermal degradation.
Thermal stress also affects functioning parameters of plant emission zones such as stomata. As mentioned above, maximum emission of isoprenoid is generally found at ~180°C. At temperatures between 200 and 280°C, hemicellulose is degraded, which leads to an increase in emissions of CO and CO2 (Barboni 2006). Emission of acetic acid and methanol and dehydration of cellulose are also observed. Then, an exothermic phase occurs between 280 and 320°C with the emission of the aforementioned gases in addition to low molecular weight hydrocarbons like methane, ethane or ethylene. From 240 to 360°C, dehydrocellulose (Yang et al. 2018) is degraded to char and volatile material. Finally, lignin is degraded from 280 to 500°C with high emissions of char and phenolic compounds. Concerning plant emission flow rates of volatile organic compounds (VOCs), for Mediterranean vegetation emissions, data are principally available for seasonal temperatures, e.g. 50 kg/km2 h for Valencia (Spain) (Owen et al. 2001). Very few authors have quantified emissions from heated plants. We found data for pine twigs (Zhao et al. 2012) with emissions of 16.314 μg/g dry weight (DW) of terpenoids after heating for 15 min at 200°C versus 0.042 at normal temperature. We observed that emissions may be multiplied ~400 times when a plant is heated. Depending on the species and measurement methodology, emissions are multiplied by eight for Rosmarinus officinalis (Chetehouna 2014) and more than 4000 times for Cistus monspeliensis (Barboni 2006) when the plant is heated. Regarding the flow rate of fire front emissions, presenting heterogeneous temperatures between 40 and 1000°C, interesting values of emission factors (EF) are available in the literature (Akagi et al. 2011; Simpson et al. 2011), e.g. for boreal wildfires: 4.9 g of aromatics by kg of burnt vegetation (phenols 60%, other aromatics 40%) and 3.24 g of isoprenoids (Akagi et al. 2011).
Thus, in the literature, the influences of hydric and thermal stresses on plant emissions have been studied independently but, to our knowledge, no one has studied them in combination despite their major influence during wildfires. For a better understanding of wildfire propagation from biological and thermochemical points of view, this project studied the influence of combined hydric and thermal stresses on plant physiology and emissions at the leaf and plant scales.
The objective of this study was to understand the effect of combined hydric and thermal stresses on the emission behaviours of two Mediterranean species particularly affected by wildfires, Rosmarinus officinalis and Cistus albidus. To this end, the two species were subjected to hydric stress in a first phase with a well-watered (control) and a water-deficit group. Both were then subjected to thermal stress in a second phase by lateral thermal radiation to approximate the conditions of the arrival of a distant wildfire. During these tests, we studied plant behaviour by analysing their physiology and the composition of their emissions. The experiments were carried out at the plant scale for real conditions and at the leaf scale for more controlled conditions.
Material and methods
Plant growth conditions
Rosmarinus officinalis (Lamiaceae) and Cistus albidus (Cistaceae) (Fig. 1) were chosen as Mediterranean plants typically affected by documented eruptive fires (Courty et al. 2014) but also as physiologically different plants. The plants were grown in a mixture of potting soil and vermiculite (3/1, v/v) in pot of diameter 20 cm and height 16 cm for R. officinalis and of diameter 16 cm and height 12 cm for C. albidus. Twenty plants of each species grown under well-watered condition and 20 others were submitted to hydric stress by stopping watering for 16 days: water-deprived conditions. All the cultures were placed in a greenhouse whose average temperature during the period of the experiments was 22.5 ± 5°C, and a photoperiod of 16 h day/8 h night.
Leaf water and chlorophyll content
The fresh leaf fragment was weighed (fresh weight, FW), then dried for 24 h at 80°C to determine its dry weight (DW). Based on these measurements, the leaf water content (LWC) was calculated: LWC = (FW − DW)/FW. Approximatily 0.5 g of fresh leaf was used to extract chlorophyll using acetone, and the total chlorophyll, chlorophyll a (Ca) and chlorophyll b (Cb) of the centrifuged solution were measured using the classical spectrophotometric method (Mackinney 1941). To estimate the available water for the plant in the pot, the soil volumetric water content was measured with a portable Fieldscout TDR 150 soil moisture instrument (Spectrum Technologies Inc.) with 12-cm long rods.
Microscopic analyses
Leaf samples were immediately fixed for 1 h at 4°C in a mixture of 2% paraformaldehyde and 0.5% glutaraldehyde in 0.05 M phosphate buffer (v/v v), pH 7.2. After three washes for 20 min each in 0.2 M phosphate buffer, pH 7.2, supplemented with 7.5% sucrose, a post-fixation step in 1% osmium tetroxide for 5 min followed. The dehydrated samples were embedded in LR White resin according to Fleurat-Lessard et al. (2016). Cross sections were made using a microtome (EMUC6, Leica, Wetzlar, Germany), stained with toluidine blue and observed with light microscopy (Zeiss Axioplan, Berlin, Germany).
Heating tests at leaf scale
During the plant hydric stress conditioning, leaf samples were cut from both well-watered and water-deficient plants, and heated using a Multi-shot Pyrolyzer EGA/PY-3030-D (Frontier lab) coupled with a QP2010 Ultra (Shimadzu) gas chromatograph-mass spectrometer (GC-MS). The heating products were carried by helium gas flow at 1 mL/min to the GC and separated on a TR5-MS capillary column (30 m, 0.25 mm internal diameter, 0.25 µm phase thickness). The split/splitless injector was set to 250°C with a 50/1 split ratio. The temperature of the GC oven was programmed from 60°C (1 min isotherm) to 300°C (11 min isotherm) at a 5°C/min rate. The interface temperature was set to 280°C. The MS was a quadrupole analyser, the ionisation mode was electron impact (70 eV) and the temperature of the source was set to 200°C. Acquisition was carried out in full scan mode from m/z 50 to 600 at 0.2 amu/s.
Fifteen milligrams of leaves (equivalent to one rosemary leaf or a piece of cistus leaf) was placed into an stainless steel cup and heating was carried out in isothermal mode at 180°C for 1 min. This temperature was chosen as it produced the highest VOC emission yield (Courty et al. 2014). Leaves were stored inside glass tubes covered with aluminium foil at −21 ± 1°C between experiments.
Heating tests at plant scale
For this part, we used a 1 m3 compartment equipped with a radiant panel (Fig. 2a). The latter can provide a maximum of 84 kW m2 of radiative flux and is has a fire-resistant glazed window for plant observation. It was instrumented with thermocouples, fluxmeters and a cooled scale. Adsorbent tubes were used during heating to trap VOCs, which were thermally desorbed (TD), separated by GC and identified using mass spectrometry using TD-GC-MS (Fig. 2b). The thermodesorption products were identified by comparison of their mass spectra with the NIST (National Institute of Standards and Technology) mass spectral library by their retention times. The adsorbent tubes, known as SPEE (solid-phase extraction elements), were titanium tubes (30 mm × 1.2 mm i.d. × 1.6 mm o.d.) coated with a 500 µm thick phase of polydimethylsiloxane (PDMS). During the tests, the SPEE were placed at the bottom inside (Fig. 3a), at the top inside (Fig. 3b), and at the top outside (Fig. 3c) of the heating compartment. In the cases of ‘top’ and ‘outside’, we tried vacuum extraction for active sampling.
After hydric stress conditioning, both well-watered and water-deficit plants were retrieved from the greenhouse and introduced one by one into the heating compartment (Fig. 2a). Plants were cut at their bases and, depending on their size, one or two superimposed plants were attached to a stand at 50 cm from the radiant panel (Fig. 2a). From preliminary tests, we chose to impose a temperature/heat flux ramp of 30 min not to exceed 200°C at the plant stem level in order to avoid hemicellulose degradation and study only plant emissions. The temperature ramp rate was ~6°C/min. During each heating test, we monitored the temperature, heat flux and light gases concentration with a continuous analyser. Thermocouples were placed at the same distance from the radiant panel as the stem of the plants (Fig. 2a). SPEE were introduced in the TD-GC-MS device to desorb, separate, identify and quantify VOC emissions. The thermodesorption of adsorbed compounds was performed from 150 to 280°C with a heating rate of 500°C/min and 1 min at the final temperature. Reproducibility was checked with triplicates of SPEE thermodesorption.
Results
Physiological analyses
In the first step, all cistus and rosemary plants were well watered, then at the beginning of the experiment, half of the plants for each species were subjected to water stress by totally stopping water supply for 16 days. In parallel, control plants were watered normally for the same amount of time. Physiological analyses were made on 15 leaves by species and by culture conditions to get consistent results. The volumetric water content was 90% for well-watered soil and 30% without water supply. The LWC was 73% ± 7 and 70% ± 5 respectively in cistus and rosemary leaves under well-watered growing conditions. After 16 days without water supply, the LWC of leaves was reduced by 15% and 16% in cistus and rosemary respectively (Table 1). The total chlorophyll content (Ca + Cb) was 4.84 mg/g DW ± 0.72 and 5.64 mg/g DW ± 1.38 respectively in cistus and rosemary leaves under well-watered growing conditions (Fig. 4). After 16 days without water, the total chlorophyll content (Ca + Cb) of leaves was reduced by 52 and 51% (56 and 44% for total chlorophyll content by direct measurement at 652 nm) in cistus and rosemary respectively. The decrease in total chlorophyll content is due to both lower chlorophyll a and chlorophyll b.
Leaf water content (LWC, %) | Cistus albidus | Rosmarinus officinalis |
Well-watered plant | 73 ± 7 | 70 ± 5 |
Water-deficit plant | 62 ± 4 | 59 ± 3 |
Chlorophyll content per gram dry weight of Rosmarinus officinalis and Cistus albidus leaves. Total chlorophyll content by direct measurement at 652 nm wavelength: Chlorophyll a content (Ca); Chlorophyll b content (Cb); Total chlorophyll content calculated: Ca + Cb.
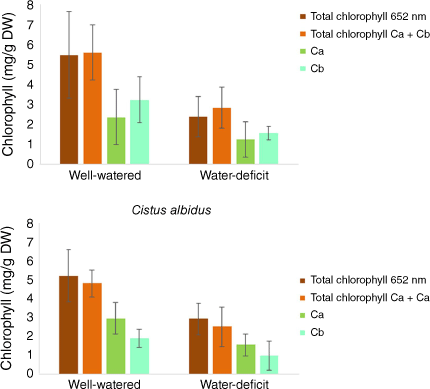
Light microscopy of cistus leaf sections under well-watered growing conditions shows the presence of an upper epidermis (adaxial) composed of bulky cells with thick cell walls (Fig. 5a, b). The palisade parenchyma and the lacunar parenchyma are composed of cells whose contents have a homogeneous aspect. The lower epidermis (abaxial) is composed of cells with a thicker cell wall than parenchyma cells. For both upper and lower epidermis, the cell walls are lignified (Fig. 5a, b). The presence of stomata is visible only on the lower surface of the leaves. After 16 days of water deprivation, the content of the cells appears denser and more heterogeneous, especially in the cells of the palisade parenchyma, which are plasmolysed (Fig. 5c, d). The volume of the palisade parenchyma cells is reduced. The presence of stomata is visible on the lower surface of the leaves (Fig. 5b, d). The different tissues are much more compact compared with the leaves under well-watered conditions (Fig. 5a, c). In the case of rosemary under well-watered conditions, there is a visible cuticular layer (Fig. 5e, f). The adaxial epidermis consists of a thin cell layer. The sclerenchyma is composed of large cells with thick cell walls and with visible pits. The palisade parenchyma and lacunar parenchyma show turgid cells where chloroplasts are pushed against the cell wall by the vacuole. After 16 days without water supply, the cell wall of the cells forming the adaxial epidermis are considerably thickened. Moreover, the sclerenchyma tissue is reduced and the cell wall is further stained by toluidine blue (Fig. 5g, h). Some cells of the palisade parenchyma show a turgid protoplast while others are empty with only the cell wall visible. The thickness of the leaves is reduced compared with the leaves under well-watered conditions and they also appear more compact (Fig. 5e, g).
Cross section of Cistus albidus (a–d) and Rosmarinus officinalis (e–h) leaves; plant growing conditions: well-watered (a, b, e, f), and without water supply for 16 days (c, d, g, h). Cu, cuticle; Eps, superior epidermis (adaxial); Epi, inferior epidermis (abaxial); Sc, Sclerenchyma; Pi, pit; Pp, Palisade parenchyma; Lp, lacunar parenchyma; Chl, Chloroplast; V, vacuolar; St, stomata; Sg, Secretory gland; Dc, Dead cell. Tannins, asterisk; cell plasmolysis, arrow. Semi-thin section, toluidine blue staining, optical microscopy.
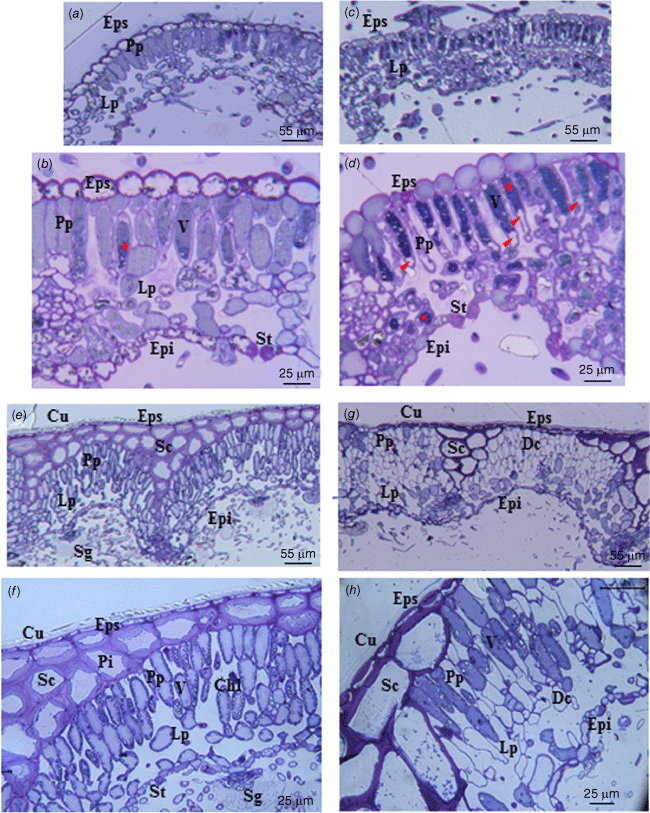
Emitted VOCs from heated sampled leaves
In this section, we heated the leaves sampled on both well-watered plants (control group) and water-deficit plants. The pyrolysis of cistus and rosemary samples was carried out in triplicate and showed good reproducibility (Fig. 6). During heating tests, C. albidus mainly emitted long chain alkanes, which accounted for 85% of the total emissions for the well-watered group leaves and 68% for the water-deficit plant ones (Fig. 7). Long C23 to C32 alkanes were observed for both well-watered and water-deficit group leaves with odd compounds being predominant. This alkane distribution, characteristic of plant origin, is generally associated with the epicuticular waxes and protective layer in vascular plants (Pascaud et al. 2017). Shorter C21 and C22 alkanes were only emitted by the stressed plant. Short fatty acids from C8 to C18, which represented 5% of the emissions for the well-watered group, increased to 27% for the stressed leaves while their distribution remained similar (Fig. 7). Terpenes were only emitted by well-watered group leaves, and accounted for 3% of the total emissions.
Reproducibility of relative abundance of products from leaf heating of well-watered Cistus albidus (180°C for 1 min). M is for molar mass (g/mol), FA is for fatty acid and Cn is for alkane, n corresponding to the number of carbon atoms.
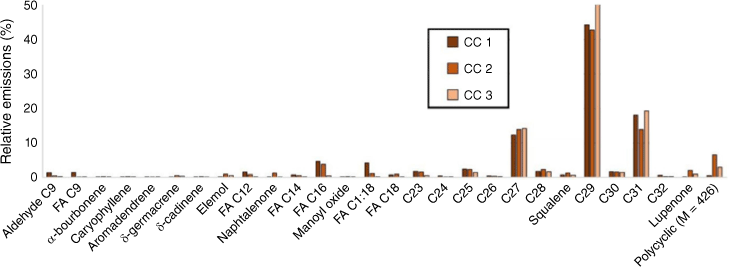
Relative abundance of products from leaf heating of well-watered and water-deficit Cistus albidus (180°C for 1 min). HCn, alkane; FAn, fatty acid; n, number of carbons.
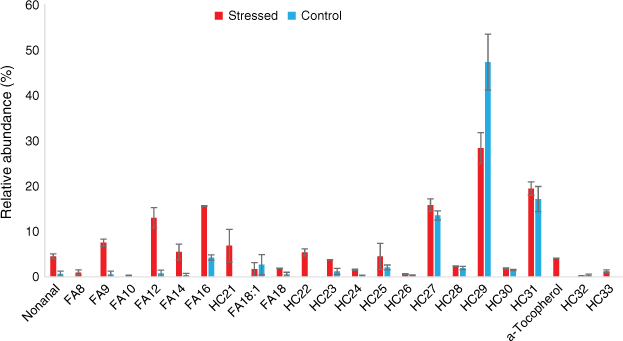
Rosemary mainly emitted hydrocarbons, terpenes, fatty acids and acetates (Figs 8 and 9). Alkanes accounted for respectively 69 and 34% and terpenes respectively 25 and 58% of the total emissions of well-watered and water-deficit rosemary. The main monoterpenes were α-pinene, eucalyptol, linalool, camphor, borneol and verbenone. Long-chain alkanes ranged from C27 to C33 and their distribution was not affected by hydric stress (Fig. 8). Short even-chained fatty acids were observed from 12 to 18 carbon atoms and slightly increased from 4 to 7% with hydric stress. Tetramethoxyflavone and ferruginol, which were observed as traces in the well-watered group, accounted respectively for 1 and 3.5% in water-deficit rosemary.
Emitted VOCs from heated plants
In order to optimise the adsorption of emitted VOCs, three locations were compared for the SPEE: at the bottom inside the heating compartment (bottom, Fig. 3a); at the top inside the heating compartment (top, Fig. 3b); at the top outside the heating compartment (outside, Fig. 3c). In the cases of ‘top’ and ‘outside’, we used active (vacuum extraction) or passive sampling. Fig. 10 compares the results of the three SPEE locations for heating tests on water-deficit Rosmarinus officinalis.
Alkanes distribution for different locations of SPEE during heating tests at the plant scale for water-deficit Rosmarinus officinalis.
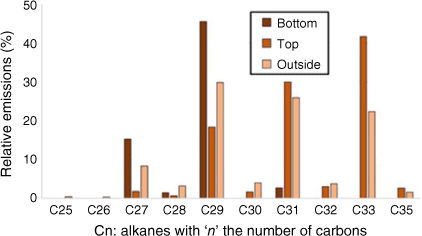
Active sampling with the adsorbent tube placed outside the heating compartment demonstrated the most representative results as the alkanes obtained were long odd-chained, which is characteristic of a plant distribution (Kolattukudy 1976).
For some tests, incandescence was observed for the leaf closest to the radiant panel. This seemed to have little impact on results because the results obtained while activating the pump at different moments before and after glowing were similar (Fig. 11).
Alkanes distribution trapped in SPEE placed outside the heating compartment during the tests at the plant scale for well-watered Rosmarinus officinalis with active sampling.
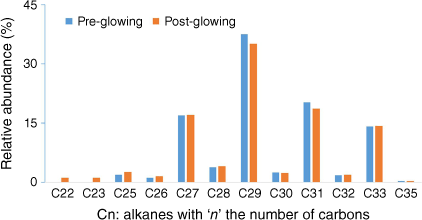
Long alcohol chained esters (octanoates, decanoates and dodecanoates) were observed amongst the heating products of R. officinalis at the plant scale. Long-chain esters can originate from the cutin, a biopolymer part of the cuticle of plants. These hydrophobic polyesters give their glossy aspect to leaves or non-woody stems and protect the plant from water loss, among other functions (Heredia 2003).
Discussion
The LWC is a good drought indicator because it is intimately connected with atmospheric aridity, soil water availability and plant drought tolerance (Zhou et al. 2021). In our greenhouse conditions, under well-watered growing conditions, we found that the LWC value of 70% ± 5 for rosemary was comparable with the literature (73%; Szumny et al. 2010) whereas for cistus, the mean value of 73% ± 7 was low compared with other studies (C. creticus 80.4%; Stępień et al. 2019). Also, we hypothesise that our cistus well-watered plants were probably under slight water stress. Otherwise, compared with the LWC of Arabidopsis thaliana in well-watered growth conditions (93.3 ± 1.1%; Slawinski et al. 2021), a species with no particular adaptation to drought and which is not a so-called Mediterranean plant, the LWC values of rosemary and cistus are low.
The leaves of rosemary and cistus are typical of Mediterranean species adapted to drought and they are characterised by the presence of a thick cuticle in the case of rosemary and a dense abaxial indumentum for cistus. In our experimental conditions, in cistus, the heterogeneous aspect in the vacuole is probably due to the presence of tannin, these cells exhibiting a blue–black colour with toluidine blue (Robil and Tolentino 2015). Indeed, tannins in vacuoles generally have polymorphic aspects that have been described as semifluid, with a granular, filamentous, or reticular network or as solid, with dense corpuscles with an amorphous arrangement (Fleurat-Lessard et al. 2016). The presence of polyphenols such as tannins in cistus under normal developmental conditions has been widely reported (Nicoletti et al. 2014) and, more generally, the positive effect of water deficit on these secondary metabolites (Munné-Bosch et al. 2003; Bucchetti et al. 2011).
In our experimental conditions, the anatomic studies of rosemary demonstrated that a water loss in the leaves of 15.5% modified the structure of the latter, with a thickening of the wall of the cells of the upper epidermis and modification of the cell wall of the sclerenchyma cells. The cell wall staining suggests an increase of lignification, an adaptation to drought that limits non-stomatal transpiration (O’Brien et al. 1964; Olmos et al. 2006; Herrera-Ubaldo and de Folter 2018). The lower thickness of the leaf and the density of the tissues is also a consequence of the loss of water in the cells (which reduces gas exchange). These changes in leaf anatomy affect CO2 diffusion (Chartzoulakis et al. 2002). Also, we observed a high amount of dead cells compared with rosemary grown in well-watered conditions. Our experimental conditions in terms of water deficit were quite drastic owing to the low volumetric soil water content (30%). All the dead cells present in the parenchyma no longer contained chlorophyll, which explains the sharp decrease in chlorophyll content in rosemary (56%). These cells no longer participate in the metabolism and thus in the synthesis of terpenes.
The rosemary and cistus responded in a similar way to water deficit with a decrease in total chlorophyll content (Fig. 4) due to the increased degradation of photosynthetic pigments (Enneb et al. 2020) and to the death of some cells (Fig. 5h) due to the low plant LWC (Table 1: 59% ± 3 and 62% ± 4 respectively for rosemary and cistus leaves). Water deficit affected chlorophyll a and chlorophyll b content in the same way and had an impact on photosynthesis and secondary metabolism (Azhar et al. 2011).
However, the relative percentage of terpenes and fatty acids emissions increased with hydric stress whereas the relative percentage of alkanes decreased for rosemary. The increase in relative percentage of fatty acids emission was observed for both plant species. Fatty acids are part of the cutin that acts as a barrier for water loss and pathogen protection (Cahoon and Li-Beisson 2020), which could explain their relative increase with hydric stress. Changes in relative percentages of the emitted compounds with hydric stress could impact plant flammability. Indeed, monoterpenes emitted by rosemary are the most flammable compounds, with a lower flammability limit (LFL) of ~0.7% by volume and an auto-ignition temperature (AIT) of ~250°C. There are few data for fatty acids about LFL or AIT because they have a high boiling point, e.g. 232°C for α-linolenic acid; they are considered less flammable than hydrocarbons with similar carbon numbers, e.g. the flash point of hexadecanoic acid is 206°C and for hexadecane, it is 135°C with an AIT of 201°C, although we found an AIT of 261°C ± 5°C in the safety data sheet of fatty acids for C10–18 and C12–22-unsatured, C14–18 and C16–18-unsatured alkyl esters (Mercuria 2015).
The compounds responsible for plant ignition must be emitted by the plants in quantities of the same order of magnitude as their LFL to compose a flammable mixture with the other compounds. The LFL of gaseous mixtures can be approximately estimated thanks to Le Chatelier’s mixing rule (Mashuga and Crowl 2004). To favour plant ignition, gaseous compounds also must have a low AIT. Finally, density may play a role, knowing that a density higher than air will favour accumulation at ground level during heating while the fire is approaching. As for the boiling point, the lower it is, the more easily a compound is emitted by the plant. These different properties are presented in Table 2 for the main compounds detected during wildland fires. Monoterpenes, long-chain alkanes and fatty acids have properties more favourable than the other molecules to trigger auto-ignition, with lower LFLs and AITs and higher densities. Sesquiterpenes may play a role too but we do not have enough data on AITs and emission factors. We also note that aromatics formed at higher temperatures – with vegetation degradation and combustion, and dispersed by fire smoke – have a higher AIT than the abovementioned molecules but can play a role in the stability of the flame because they have a low LFL (Coudour et al. 2015, 2016a).
Molecule | LFL% vol at 25°C (NF EN 1839 2017) | AIT (°C) | Density/air | Boiling point (°C) | Emission factor (EF, g/kg burnt) pine + oak (Akagi et al. 2013) |
Benzene C6H6 | 1.4 | 555 | 2.7 | 80 | 1.7 |
Toluene C7H8 | 1.2 | 480 | 3.1 | 111 | 0.9 |
Phenol C6H6O | 1.7 | 715 | 3.2 | 181 | ? |
Isoprene C5H8 | 1.5 | 220 | 2.3 | 34 | ? |
Monoterpenes C10H16 | 0.7 | 255 | 4.7 | 156 | 13.2 |
Sesquiterpenes C15H24 | 0.5 | ? | 7 | 245 | ? |
Alpha-kaurene C20H32 | 0.4 | ? | 9.5 | 344 | ? |
Tetradecane C14H30 | 0.5 | 200 | 6.83 | 253 | ? |
Lauric acid C12H24O2 | 0.6 | 261 | 6.91 | 225 | ? |
Pentacosane C25H52 | ? | ? | 12 | 403 | ? |
Carbon monoxide CO | 12.5 | 609 | 0.98 | −191 | 222 |
Carbon dioxide CO2 | – | – | 1.5 | −78.5 | 1305 |
Methane CH4 | 4.4 | 537 | 0.6 | −161 | 10.3 |
Total hydrocarbons | – | – | – | – | 57 (NMOC) |
Values are taken from safety data sheets except for LFL and Emission Factors.
Conclusion
Cistus albidus and Rosmarinus officinalis reaction to hydric and thermal stresses was monitored through biological, chemical and physical parameters. The physiological analyses showed that C. albidus, with its large leaves covered by trichomes, faced hydric stress better than R. officinalis, whose chlorophyll content decreased and whose cells began to die rapidly. The VOC emissions determined by pyrolysis coupled to GC-MS at the leaf scale and TD-GC-MS showed differences between the two species. Both emitted mainly long odd-chained alkanes and short fatty acids but R. officinalis also emitted monoterpenes, which increased strongly with hydric stress. For both species, fatty acids emissions increased relatively to alkanes, which could be aimed at limiting evapotranspiration. Tests at the leaf scale showed good reproducibility and the increase of monoterpene emissions when R. officinalis was hydrically stressed tends to make it more flammable. Alkane emissions of well-watered and water-deficit R. officinalis monitored at the plant scale using SPEE showed similar distribution to those observed at the leaf scale. Results of the composition of emissions at plant scale were in agreement with the ones at leaf scale. This work showed that plant species do not react equally with hydric stress or with combined hydric and thermal stresses. Several experiments were conducted in order to improve emission sampling with SPEE method in the compartment configuration. The active sampling from the outside of the compartment gave the best results. Concerning the influence of combined hydric and thermal stresses on fire propagation, these results suggest that both a lower content of water and a higher content of monoterpenes and fatty acids could induce an increase in plant flammability. Tests will be done in further work to determine experimentally the LFL of gaseous mixtures from wildfires to check the influence of the aforementioned compounds on flammability, theoretical LFL can be estimated thanks to Le Chatelier’s mixing rule but it is not accurate enough with these kinds of compounds. The resulting protocol will be used to measure emissions at the plant scale in compartment conditions in further study, to which we plan to add quantitative measurement of emissions. These experiments could be carried out with other plant species to help better know why Mediterranean wildfires are a particular threat and how to improve firefighting methods.
Declaration of funding
We acknowledge Labex Interactifs of P’ Institute, which gave us an internship grant of Master 2 to work on the biological part with Mrs Kenza Ait Ali Yahia. We acknowledge EUR-InTREE, a new training about interfaces from a physico-chemical aspect in Poitiers, which gave us an internship grant of Master 1 to workon the chemical part with Mr Axel Rigoulet. EUR-InTREE and Labex Interactifs are supported by the French government program ‘Investissements d’Avenir’ (EUR INTREE, reference ANR-18-EURE-0010). This work was supported by the French government program ‘Investissements d’Avenir’ (LABEX INTERACTIFS, reference ANR-11-LABX-0017-01). We acknowledge P’ Institute for a grant to finance heating compartment preparation, heating testing at leaf scale as well as plant and SPEE orders. We acknowledge EBI Laboratory for use of the greenhouse for the biology experiments.
Acknowledgements
The authors would like to thank Vincent Lebeurre for plant production. We also acknowledge Khaled Chetehouna from PRISME laboratory (Bourges-France) for the loan of the heating compartment equipped with a radiant panel. Finally, we acknowledge Masters students Kenza Ait Ali Yahia and Axel Rigoulet for their participation in this project as part of their internships.
References
Akagi SK, Yokelson RJ, Wiedinmyer C, Alvarado MJ, Reid JS, Karl T, Crounse JD, Wennberg PO (2011) Emission factors for open and domestic biomass burning for use in atmospheric models. Atmospheric Chemistry and Physics 11, 4039-4072.
| Crossref | Google Scholar |
Akagi SK, Yokelson RJ, Burling IR, Meinardi S, Simpson I, et al. (2013) Measurements of reactive trace gases and variable O3 formation rates in some South Carolina biomass burning plumes. Atmospheric Chemistry and Physics 13, 1141-1165.
| Crossref | Google Scholar |
Azhar N, Hussain B, Ashraf M, Abbasi K (2011) Water stress-mediated changes in growth, physiology and secondary metabolites of desi ajwain (Trachyspermum Ammi L.). Pakistan Journal of Botany 43, 15-19.
| Google Scholar |
Bucchetti B, Matthews MA, Falginella L, Peterlunger E, Castellarin SD (2011) Effect of water deficit on Merlot grape tannins and anthocyanins across four seasons. Scientia Horticulturae 128, 297-305.
| Crossref | Google Scholar |
Cahoon EB, Li-Beisson Y (2020) Plant unusual fatty acids. Current Opinion in Plant Biology 55, 66-73.
| Crossref | Google Scholar |
Chartzoulakis K, Patakas A, Kofidis G, Bosabalidis A, Nastou A (2002) Water stress affects leaf anatomy, gas exchange, water relations and growth of two avocado cultivars. Scientia Horticulturae 95, 39-50.
| Crossref | Google Scholar |
Chatelon FJ, Sauvagnargues S, Dusserre G, Balbi JH (2014) Generalized Blaze Flash, a ‘Flashover’ Behavior for Forest Fires – Analysis from the Firefighter’s Point of View. Open Journal of Forestry 4, 547-557.
| Crossref | Google Scholar |
Ciccioli P, Centritto M, Loreto F (2014) Biogenic volatile organic compound emissions from vegetation fires. Plant, Cell & Environment 37, 1810-1825.
| Crossref | Google Scholar |
Coudour B, Chetehouna K, Rudz S, Gillard P, Garo JP (2015) Investigation on minimum ignition energy of mixtures of α-pinene–benzene/air. Journal of Hazardous Materials 283, 507-511.
| Crossref | Google Scholar |
Coudour B, Chetehouna K, Halter F, Mounaïm-Rousselle C, Garo JP (2016a) Laminar burning speeds of α-pinene/benzene/air mixtures involved in the combustion in forest fires. Combustion, Science & Technology 188, 2128-2136.
| Google Scholar |
Coudour B, Chetehouna K, Conan B, Aubrun S, Kaiss A, Garo J-P (2016b) Experimental and numerical investigations of the geometry influence on gas accumulation using a V-shaped forest model. Atmospheric Environment 141, 67-79.
| Crossref | Google Scholar |
Courty L, Chetehouna K, Halter F, Foucher F, Garo JP, Mounaïm-Rousselle C (2012) Flame speeds of α-pinene/air and limonene/air mixtures involved in accelerating forest fires. Combustion Science and Technology 184(10-11), 1397-1411.
| Crossref | Google Scholar |
Courty L, Chetehouna K, Lemée L, Fernandez-Pello C, Garo JP (2014) Biogenic volatile organic compounds emissions at high temperatures of common plants from Mediterranean regions affected by forest fires. Journal of Fire Sciences 32(5), 459-479.
| Crossref | Google Scholar |
Enneb H, Ben Yahya L, Ilyas M, Asaram Dhale D, Bagues M, Nagaz K (2020) Influence of water stress on growth, chlorophyll contents and solute accumulation in three accessions of Vicia faba L. from Tunisian arid region. In ‘Abiotic Stress in Plants’ Chap. 4. (Eds S Fahad, S Saud, Y Chen, C Wu, D Wang) (IntechOpen) doi:10.5772/intechopen.94563
Finney MA, Cohen JD, Forthofer JM, et al. (2015) Role of buoyant flame dynamics in wildfire spread. Proceedings of the National Academy of Sciences 112(32), 9833-9838.
| Crossref | Google Scholar |
Fleurat-Lessard P, Béré E, Lallemand M, Dédaldéchamp F, Roblin G (2016) Co-occurrence of tannin and tannin-less vacuoles in sensitive plants. Protoplasma 253, 821-834.
| Crossref | Google Scholar |
Frangieh N, Accary G, Morvan D, Méradji S, Bessonov O (2020) Wildfires front dynamics: 3D structures and intensity at small and large scales. Combustion and Flame 211, 54-67.
| Crossref | Google Scholar |
Heredia A (2003[PMID: 12595066]) Biophysical and biochemical characteristics of cutin, a plant barrier biopolymer. Biochimica et Biophysica Acta (BBA) - General Subjects 1620(1–3), 1-7.
| Crossref | Google Scholar |
Herrera-Ubaldo H, de Folter S (2018) Exploring cell wall composition and modifications during the development of the gynoecium medial domain in Arabidopsis. Frontiers in Plant Science 9, 454.
| Crossref | Google Scholar |
Lemoine R, La Camera S, Atanassova R, Dédaldéchamp F, et al. (2013) Source-to-sink transport of sugar and regulation by environmental factors. Frontiers in Plant Science 4, 272.
| Crossref | Google Scholar |
Llusià J, Peñuelas J (1998) Changes in terpene content and emission in potted Mediterranean woody plants under severe drought. Canadian Journal of Botany 76(8), 1366-1373.
| Crossref | Google Scholar |
Mackinney G (1941) Absorption of light by chlorophyll solutions. Journal of Biological Chemistry 140, 315-322.
| Crossref | Google Scholar |
Mashuga CV, Crowl DA (2004) Derivation of Le Chatelier’s mixing rule for flammable limits. Process Safety Progress 19(2), 112-117.
| Crossref | Google Scholar |
Munné-Bosch S, Jubany-Mari T, Alegre L (2003) Enhanced photo- and antioxidative protection, and hydrogen peroxide accumulation in drought-stressed Cistus clusii and Cistus albidus plants. Tree Physiology 23, 1-12.
| Crossref | Google Scholar |
Nagegowda DA, Gupta P (2020) Advances in biosynthesis, regulation, and metabolic engineering of plant specialized terpenoids. Plant Science 294, 110457.
| Crossref | Google Scholar |
Nicoletti M, Toniolo C, Venditti A, Bruno M, Ben Jemia M (2014) Antioxidant activity and chemical composition of three Tunisian cistus: Cistus monspeliensis, Cistus villosus and Cistus libanotis. Natural Product Research 29, 223-230.
| Crossref | Google Scholar |
O’Brien TP, Feder N, McCully ME (1964) Polychromatic staining of plant cell walls by toluidine blue O. Protoplasma 59, 368-373.
| Crossref | Google Scholar |
Olmos E, Kiddle G, Pellny T, Kumar S, Foyer CH (2006[Epub. PMID: 16720601]) Modulation of plant morphology, root architecture, and cell structure by low vitamin C in Arabidopsis thaliana. Journal of Experimental Botanic 57(8), 1645-1655.
| Crossref | Google Scholar |
Ormeño E, Mévy JP, Vila B, Bousquet-Mélou A, Greff S, Bonin G, Fernandez C (2007) Water deficit stress induces different monoterpene and sesquiterpene emission changes in Mediterranean species. Relationship between terpene emissions and plant water potential. Chemosphere 67(2), 276-284.
| Crossref | Google Scholar |
Owen SM, Boissard C, Nicholas Hewitt C (2001) Volatile organic compounds (VOCs) emitted from 40 Mediterranean plant species: VOC speciation and extrapolation to habitat scale. Atmospheric Environment 35, 5393-5409.
| Crossref | Google Scholar |
Pascaud G, Soubrand M, Lemee L, Laduranty J, El Mufleh A, Rabiet M, Joussein E (2017) Molecular fingerprint of soil organic matter as an indicator of pedogenesis processes in Technosols. Journal of Soils and Sediments 17, 340-351.
| Crossref | Google Scholar |
Robil JLM, Tolentino VS (2015) Histological localization of tannins at different developmental stages of vegetative and reproductive organs in Medinilla magnifica (Melastomataceae). Flora - Morphology, Distribution, Functional Ecology of Plants 217, 82-89.
| Crossref | Google Scholar |
Rudz S, Chetehouna K, Strozzi C, Gillard P (2014) Minimum ignition energy measurements for α-pinene/air mixtures. Combustion Science and Technology 186, 1597-1605.
| Crossref | Google Scholar |
Schneider L, Betting B, Patterson M, Skowronski N, Simeoni A (2021[ISSN 0379-7112]) Experimental study of fire spread through discontinuous fuels without flame contact. Fire Safety Journal 120, 103066.
| Crossref | Google Scholar |
Silveira FAO, Rossatto DR, Heilmeier H, Overbeck GE (2022[ISSN 0367-2530]) Fire and vegetation: Introduction to the special issue. Flora 286, 151985.
| Crossref | Google Scholar |
Simpson IJ, Akagi SK, Barletta B, et al. (2011) Boreal forest fire emissions in fresh Canadian smoke plumes: C1–C10 volatile organic compounds (VOCs), CO2, CO, NO2, NO, HCN and CH3CN. Atmospheric Chemistry and Physics 11, 6445-6463.
| Crossref | Google Scholar |
Slawinski L, Israel A, Artault C, Thibault F, Atanassova R, Laloi M, Dédaldéchamp F (2021) Responsiveness of early response to dehydration six-like transporter genes to water deficit in Arabidopsis thaliana leaves. Frontiers in Plant Science 12, 708876.
| Crossref | Google Scholar |
Stępień AE, Gorzelany J, Matłok N, Lech K, Figiel A (2019) The effect of drying methods on the energy consumption, bioactive potential and colour of dried leaves of Pink Rock Rose (Cistus creticus). Journal of Food Science and Technology 56, 2386-2394.
| Crossref | Google Scholar |
Szumny A, Figiel A, Gutiérrez-Ortíz A, Carbonell-Barrachina ÁA (2010) Composition of rosemary essential oil (Rosmarinus officinalis) as affected by drying method. Journal of Food Engineering 97, 253-260.
| Crossref | Google Scholar |
Viegas DX (2009) Recent forest fire accidents in Europe. (Ed. DX Viegas) (Joint Research Centre, Institute for Environment and Sustainability) 10.2788/50781
Yang X, Zhao Y, Li R, Wu Y, Yang M (2018) A modified kinetic analysis method of cellulose pyrolysis based on TG–FTIR technique. Thermochimica Acta 665, 20-27.
| Crossref | Google Scholar |
Zhao FJ, Shu LF, Wang QH (2012) Terpenoid emissions from heated needles of Pinus sylvestris and their potential influences on forest fires. Acta Ecologica Sinica 32(1), 33-37.
| Crossref | Google Scholar |
Zhou H, Zhou G, He Q, Zhou L, Ji Y, Lv X (2021) Capability of leaf water content and its threshold values in reflection of soil–plant water status in maize during prolonged drought. Ecological Indicators 124, 107395.
| Crossref | Google Scholar |