Evaluating wildfire vulnerability of Mediterranean dwellings using fuzzy logic applied to expert judgement
Alba Àgueda



A Department of Chemical Engineering, Centre for Technological Risk Studies, Universitat Politècnica de Catalunya–BarcelonaTech, Barcelona, Spain. Email: pascale.vacca@upc.edu, eulalia.planas@upc.edu, elsa.pastor@upc.edu
International Journal of Wildland Fire 32(6) 1011-1029 https://doi.org/10.1071/WF22134
Submitted: 1 July 2022 Accepted: 10 May 2023 Published: 6 June 2023
© 2023 The Author(s) (or their employer(s)). Published by CSIRO Publishing on behalf of IAWF. This is an open access article distributed under the Creative Commons Attribution-NonCommercial-NoDerivatives 4.0 International License (CC BY-NC-ND)
Abstract
Background: Wildland–urban interface (WUI) fires pose great challenges to firefighting services and there is a growing need for self-protection and the creation of fire-adapted communities.
Aim: A tool that can aid homeowners and residents of the Mediterranean WUI was created so that they can identify vulnerabilities present on their properties and consequently reduce them in order to reduce the risk of fires igniting dwellings.
Methods: This Vulnerability Assessment Tool is based on a fault tree analysis that includes possible structural vulnerabilities as well as the different ways a fire could spread on a property to finally enter and ignite a dwelling. The probabilities of the different events are obtained from fuzzy preferences of WUI experts.
Key results: Our system was tested against real-world data taken from two WUI fires that occurred in 2021 and 2022 in Spain in which several dwellings were affected, with different degrees of damage (minor to major losses).
Conclusions: The tool is able to show the vulnerabilities of the properties and to account for differences in building characteristics and vegetation management at the parcel scale.
Implications: A planned use of this tool would be key to improving fire resilience at the community level.
Keywords: experts poll, fire risk awareness, fire vulnerability index, fuel management, fuzzy inference system, homeowners, probability of failure, wildland–urban interface.
Introduction
Fires at the wildland–urban interface (WUI) are increasing in frequency and severity, resulting in catastrophic events that take a heavy toll in human life and structure losses (Ganteaume et al. 2021). These events often overwhelm firefighter capacities owing to the need for a simultaneous response of wildfire suppression, community evacuation and structure protection, therefore highlighting the need for the creation of fire-adapted homes and communities, which can safely co-exist with fire (Vacca et al. 2020b). Risk reduction strategies that include preventive actions not only at the community scale (i.e. the WUI mesocale), but also at the parcel level (i.e. the WUI microscale) are needed to reach this goal, as case studies indicate that the construction characteristics (e.g. materials and design details) of a building as well as the characteristics of its immediate surroundings determine a home’s ignition potential in a WUI fire, thus influencing its chances of survival (Cohen 2000; Hakes et al. 2017).
The analysis of past fire events all over the world has resulted in the identification of the different pathways leading to building ignition, which have been summarised by Hakes et al. (2017). Consequently, some countries that have historically been affected by wildfires at the WUI have created building codes, standards and guidelines for new and/or existing buildings that include WUI parcel-scale risk reduction strategies related to both building and property characteristics. These include mainly general requirements based on experience, and knowledge on their effectiveness is limited. However, recent studies on WUI fires (e.g. Camp Fire, California) have led to the creation of a Hazard Mitigation Methodology in the USA in order to reduce structural losses by hardening structures and parcels, and to reduce mitigation costs (Maranghides et al. 2022). In European countries in particular, these requirements are frequently poorly supported by scientific evidence and studies, thus not taking into account the appropriate parameters and processes that explain fire behaviour and effects on assets at the relevant scale (Pastor et al. 2019). Moreover, European regulations and guidelines are often poorly implemented (Ganteaume et al. 2021) and they do not provide any clues for the identification of interventions that should be prioritised.
A selection of representative codes, standards and guidelines, along with the building and property elements for which strategies are required or suggested, are given in Table 1. Wildfire danger is primarily defined at the landscape scale (i.e. the WUI macroscale), based on topographic, vegetation and environmental factors, and measures for vegetation management are given for this scale (Intini et al. 2019). When it comes to the microscale, the codes, standards and guidelines analysed address the need for a defensible space around the house for which fuel (vegetation or artificial fuels) removal, reduction or substitution should be performed. The radius around the house of this defensible space varies from country to country, going from approximately 10 m (30 ft) in the USA (NFPA 2022) and Canada (Government of Alberta 2013) to 50 m or more in the case of the presence of steep slopes in France (Legifrance 2021) and Portugal (Autoridade Florestal National 2008). Additionally, Canada (Government of Alberta 2013) and the USA (NFPA 2022) divide the defensible space into different zones, defined as the non-combustible or immediate zone (within 1.5 m of the dwelling), Zone 1 or intermediate zone (1.5 to approximately 10 m), and Zone 2 or extended zone (from approximately 10 to 30 m). Canada defines a third zone that goes from 30 to 100 m as well. Many of the codes, standards and guidelines analysed in the present paper also cover different building elements for which strategies are to be implemented according to each country. These building elements are the roof and gutters, for which prescriptions are set for both materials and maintenance or cleaning, vents, semi-confined spaces (i.e. spaces attached to the main structure with a certain degree of confinement in which fuels may accumulate, such as balconies, porches or areas under decks), wall materials, glazing systems and shutters. It can be noted that Portugal and Italy provide regulations for defensible space, but do not provide any guidelines on building construction or maintenance. French guidelines mention some building elements; however, they are not analysed with the appropriate detail.
Code/standard/guideline | Defensible space | Roof | Gutters | Vents | Semi-confined spaces | Façade materials | Glazing systems | Shutters | |
Materials | Maintenance | ||||||||
International WUI Code (International Code Council 2021) | × | × | × | ×A | × | × | × | × | |
AS 3959 – Australia (Australian Standards 2018) | × | × | × | × | × | × | × | × | |
FireSmart – Canada (Government of Alberta 2013) | × | × | × | × | × | × | × | × | × |
California Building Code Chapter 7A (California Building Standards Commission 2019) | x | × | × | × | × | × | × | ||
Firewise – USA (NFPA 2022)B | × | × | × | × | × | × | × | × | × |
France (Ministère de l’Écologie et du Développement durable 2002) | × | × | × | × | × | × | |||
Portugal (Autoridade Florestal National 2008) | × | ||||||||
Italian regional guidelines: Piemonte (Regione Piemonte 2021), Sardegna (Regione Autonoma della Sardegna 2022) | × | ||||||||
Spanish regional guidelines: Generalitat Valenciana (Manca and López 2014), Catalonia (Generalitat de Catalunya 2019) | × | × | × | × | × | × | × | × | × |
ARequirements for vents refer to ignition-resistant Classes 1 and 2.
BGuideline for homeowners based on NFPA Standards such as NFPA 1140 (National Fire Protection Association 2022).
Whereas Australia and the USA are provided with national standards that address the issues of the WUI microscale (in the USA, the state or local jurisdiction is in charge of adopting a code), European countries delegate the management of these issues to regional and municipality levels. In France, Italy and Spain, regions are co-responsible for wildland fire prevention and have to articulate national laws by issuing local regulations and guidelines and by ensuring compliance of provisions at the municipal level (Pastor et al. 2019).
Some of the guidelines mentioned in Table 1 (Firewise, Guidelines from the regions of Valencia and Catalonia in Spain and FireSmart) also provide checklists for WUI homeowners and residents that focus on fuel management around a house and hardening vulnerable construction elements. Whereas Firewise (USA) provides a guidance document, the other analysed checklists also provide a scoring system that yields the hazard level of the building and property (FireSmart – Canada) or the vulnerability of the building to incoming fire (Spanish regional checklists). FireSmart provides a more in-depth scoring system, dividing the defensible space into three different zones and assigning different weights to different categories: for example, the characteristics of the home and its attachments account for approximately 34% of the total score, while the different zones (0–1.5, 1.5–10, 10–30 and 30–100 m) account respectively for 7, 29, 15 and 15% of the total score – based on the latest version of the Home Ignition Zone assessment score card (FireSmart Canada 2020). Scoring for the checklists of the region of Valencia is based on whether the answer to each question is affirmative or not (i.e. the more affirmative answers, the more vulnerable the house is), whereas scoring in the region of Catalonia is divided into three different levels (high, medium or low vulnerability). The degree of detail in these two checklists designed for the Mediterranean landscape and building characteristics is, however, scarce and none of these checklists enforces instructions (Pastor et al. 2019).
This summary highlights the lack of specific WUI microscale legislation, guidelines and quantitative tools in the most wildfire-prone European countries. The focus of this work lies, therefore, in creating a Vulnerability Assessment Tool (VAT) specific for Mediterranean WUI microscale settings, directed at WUI homeowners and residents, that is supported by scientific evidence and studies, and that can provide quantitative information on the vulnerability of a property to an incoming wildfire, and can thus identify the main issues that need to be addressed. The VAT is presented in the form of a checklist that can be easily filled in by the homeowners or residents themselves and provides the probability of fire entrance into the dwelling as well as which vulnerable elements are the most critical, so that those can be prioritised. The probability of fire entrance is derived from expert judgement, and a fuzzy logic elicitation method is used to aggregate the statements of the experts. The VAT is tested through data obtained from two recent WUI fire events in Spain and it is compared with another existing tool (Papathoma-Köhle et al. 2022) that deals with Mediterranean WUI microscale vulnerabilities. The tool developed by Papathoma-Köhle et al. (2022) presents a Physical Vulnerability Index (PVI) for dwellings subject to wildfire that is based on indicators obtained from the analysis of data collected from the fire in Mati (Greece) in 2018.
The manuscript is organised as follows: first, the rationale for building the VAT for the Mediterranean region is presented along with a description of the model; the results obtained from the experts to parameterise the model are then shown; afterwards, the tool is tested by analysing two different case studies of past WUI fires, and finally, the results are discussed and compared with the PVI tool.
VAT methodology
In order to reduce the risk of home loss at the WUI, a coupled approach that reduces the vulnerability of a home to fire along with the probability of its exposure conditions is necessary (Calkin et al. 2014; Caton et al. 2017). The causes of fire entering a dwelling located at the WUI can be summarised, therefore, into two intermediate events of a fault tree, as shown in Fig. 1: one involving the exposure conditions and the other involving structural vulnerabilities typical of Mediterranean constructions. For the fire to enter a dwelling, both of these events must occur. The different paths that lead to these two intermediate events in a Mediterranean environment, identified through a literature review of past events (Vacca et al. 2020b), are further explored to develop the sketch model in Fig. 2. Quantification of the probability of failure of each of the events has proved to be difficult, as information on paths that lead to ignition during past fires is not always available or reliable, as it is mostly collected without the possibility of thorough investigation owing to lack of information or resources. To deal with this difficulty, a model that combines fuzzy logic with classical logic has been developed. It is based on the use of fuzzy sets that provide means to model the uncertainties associated with lack of information (Sivanandam et al. 2007).
Scheme for vulnerability assessment to wildland fires for dwellings at the WUI. FIS, fuzzy inference system; POF, probability of failure; ORN, ornamental vegetation; ART, artificial fuels; WLD, wildland vegetation; _1, refers to zone 1 (area within a radius of 10 m from the dwelling); _2, refers to zone 2 (a 10–30 m ring around the dwelling).
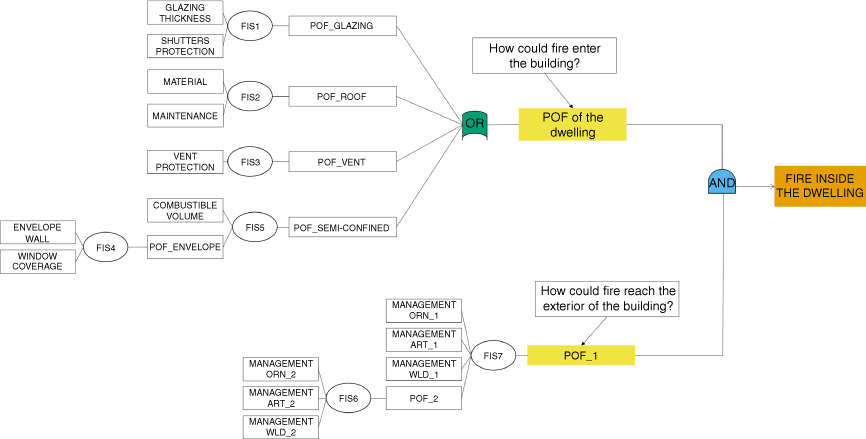
Fire exposure of the dwelling
When it comes to the ways a fire can reach a dwelling, two areas around the structure have been identified following the previously mentioned guidelines: Zone 1 includes the area in a radius of 10 m from the dwelling, while Zone 2 consists of a 10–30 m ring around the dwelling (Government of Alberta 2013; NFPA 2022). For Zone 2, a ring from 10 to 50 m was initially considered by the authors, therefore taking into account some of the European guidelines, but during the analysis of the case studies presented further in this work, it was noted that parcels do not often reach this size, and the selected zone would frequently include not only adjacent neighbours, but also those located further, adding too many variables that cannot directly be controlled by the homeowner.
In both of the selected zones, three types of fuels are considered: ornamental vegetation, artificial fuels (e.g. liquefied petroleum gas (LPG) tanks, garden furniture, sheds) and wildland vegetation. For each zone, a set of fuel management rules that can reduce the probability of the fire reaching the dwelling is established (Table 2); rules are stricter for Zone 1 owing to the close proximity to the dwelling. When it comes to vegetation, these rules are selected based on a review of existing guidelines for the management of vegetation specific to Mediterranean-type landscapes. Therefore, the analysis is limited to guidelines of the following countries or regions: France (Préfet de Vaucluse 2013; Préfet des Alpes-de-Haute-Provence 2013; Préfet de Bouches-du-Rhône 2014; Préfet des Alpes-Maritimes 2014; Préfet du Var 2015; Préfet de la Haute-Corse 2022), Portugal (Autoridade Florestal National 2008), Spain (Generalitat de Catalunya 2005; Manca and López 2014; Generalitat de Catalunya 2019), California (Cal Fire 2022), South Australia (South Australia Country Fire Service 2022) and Victoria (Victoria Country Fire Authority 2022). The Italian guidelines were also analysed; however, they do not include specific rules for the spacing of vegetation or artificial fuels within the defensible space. Overall, the strictest rules for distancing of wildland fuels are considered. When available, rules are derived from scientific findings, as is the case for the distancing of fuels from glazing systems or that between vegetation and artificial fuels. Information on spacing between artificial fuels or fuel packs (such as sheds, garden furniture, vehicles) to avoid fire spread through these types of fuels was not found in the analysed guidelines or in any scientific study. Therefore, the distance selected for these rules comes from an extrapolation of the case study of the Camp Fire in California, in which fire spread from artificial fuel to artificial fuel (or a dwelling with a façade made of combustible materials) was recorded (Maranghides et al. 2021).
Rules | Zone 1 | Zone 2 |
Wildland vegetation and/or crop landscape | ||
1. Separation between tree crowns/high shrubs should be at least 9 m (Cal Fire 2022). | × | |
2. Separation between tree crowns/high shrubs should be at least 9 m for landscapes such as canyons/ridges/hilltops and 6 m for flat or crop landscapes (Generalitat de Catalunya 2005; Cal Fire 2022). | × | |
3. Lower tree branches should be pruned at ⅓ of tree height (Generalitat de Catalunya 2005; Préfet de la Haute-Corse 2022). | × | × |
4. There should be a separation between groupings of shrubs (or regrowth forest or young trees) of at least 3 m (Generalitat de Catalunya 2005). | × | × |
5. The low surface fuel load should be maximum 10 cm deep (Morvan 2007). | × | × |
6. Trees should not overhang the roofline of the building (Préfet de Bouches-du-Rhône 2014; Cal Fire 2022; South Australia Country Fire Service 2022; Victoria Country Fire Authority 2022). | × | |
7. The vegetation should be separated by at least 4 m from any glazing system (Vacca et al. 2020a). | × | |
Ornamental vegetation | ||
1. Very flammable species (e.g. pine trees, eucalyptus, cypress, bay laurel, cherry laurel, rosemary, Japanese spindle, oleander, laurustinus) should not be present. Avoid vegetation with closely packed leaves and branches, fine textures, small, thin and narrow leaves, and high amounts of resins or oils (Manca and López 2014; Victoria Country Fire Authority 2022). | × | |
2. The vegetation should be separated by at least 4 m from any glazing system (Vacca et al. 2020a) | × | |
3. Trees should not overhang the roofline of the building (Préfet de Bouches-du-Rhône 2014; Cal Fire 2022; South Australia Country Fire Service 2022; Victoria Country Fire Authority 2022). | × | |
4. Trees and shrubs should be separated by at least 6 m (Generalitat de Catalunya 2005). | × | |
5. The distance between ornamental bushes and other vegetation should be larger than 3 m (Generalitat de Catalunya 2005). | × | |
6. Individual and groups of ornamental bushes should be less than 5 m wide (Préfet de la Haute-Corse 2022) | × | × |
7. There should be a non-continuous litter layer (Victoria Country Fire Authority 2022). | × | |
8. There should not be any dead vegetation or vegetative debris (Préfet de Vaucluse 2013; Préfet des Alpes-de-Haute-Provence 2013; Préfet de Bouches-du-Rhône 2014; Préfet du Var 2015; Cal Fire 2022). | × | |
9. Hedges should not be located within 2 m of other vegetation (Préfet de la Haute-Corse 2022). | × | × |
10. Hedges should not be aligned with the wind direction or the main slope (Vacca et al. 2020a). | × | × |
Artificial fuels | ||
1. No combustible materials should be located within 2 m of LPG tanks or canisters (Vacca et al. 2020a). | × | × |
2. No artificial fuels should be located within 5 m of glazing systems (Vacca et al. 2020a). | × | |
3. No artificial fuels should be located within 5 m of roof or gutters (Vacca et al. 2020a). | × | |
4. In the case of wild vegetation consisting mainly of trees, no artificial fuels should be placed at a distance of minimum 20 m from these trees (Cohen 2000). | × | × |
5. The distance between fuels or fuel packs should be at least 5 m (Maranghides et al. 2021). | × | × |
The impact of firebrands on the different types of fuels is indirectly included within the rules in Table 2. We assume that firebrand attack is likely to be an ignition source for fuels present in Zones 1 and 2. Now, if any fuel is ignited by firebrands and it is well managed, it will not cause fire spread to other fuels or impact the dwelling’s vulnerable elements. Failure occurs when compliance with the rules in Table 2 is not met.
Structural vulnerabilities
Structural vulnerabilities of the dwelling are also identified based on the different paths through which fire could enter. According to Vacca et al. (2020b), there are four main paths, which are associated with either gaps that already existed in the dwelling owing to bad maintenance, or that can be caused by the fire itself. These pathways involve glazing systems, roof, vents and structural damage to the dwelling’s envelope due to heat accumulation in semi-confined spaces. The failure sequence for each one of these elements is identified as shown in Fig. 2: the failure of a glazing system depends on the thickness of the glass pane (the type considered is annealed glass, given it is the most common in residential windows) and the type of shutters, with different degrees of protection depending on the material; the failure of the roof depends on the roof covering material, as well as the level of maintenance of the roof itself; the probability of fire entrance through vents is determined by the material and condition of their protection; the failure of the envelope of a semi-confined space is determined by the percentage of glazing systems present in the envelope, the amount of combustible materials stored in the space, and the material and thickness of the envelope that allows it to maintain the load bearing capacity of the structural element exposed to fire (Vacca et al. 2022). The impact of firebrands on the dwelling is implicitly considered in the failure of the four different building vulnerabilities. Given that firebrands can enter the building through gaps existing because of the building’s characteristics or gaps created owing to poor maintenance of the building itself, the presence of firebrands is strongly taken into account.
Model description
The structure of the developed model is given in Fig. 2. Note that, according to Figs 1 and 2, two system variables lead to the final output of the model (i.e. fire inside the dwelling) through a classical logical AND gate; these are: (i) probability of fire reaching the dwelling (POF_1) and (ii) probability of failure of the dwelling due to its vulnerabilities to fire (POF of the dwelling). In the same way, this last variable (ii) is obtained through a classical logical OR gate according to the values obtained for the probabilities of failure of the four elements that constitute pathways for the fire into the house, i.e. glazing systems, roof, vents and semi-confined spaces that can suffer structural damage due to heat accumulation. The probability (i) (POF_1) depends on compliance with the different rules set for fuels located in the two considered zones previously described (Table 2). All the probabilities of failure defined before the two classical logical gates are obtained through the use of fuzzy logic. This is a soft computing analytical technique that has already been used extensively in environmental risk assessments (e.g. Seguí et al. 2013) and has also been recently applied in resilience evaluation of flood-prone communities (Oladokun and Montz 2019), among other applications.
Seven fuzzy inference systems (FISs) are defined, as shown in Fig. 2. When a FIS is defined, the generic process shown in Fig. 3 must be followed. Initially, the variables that are relevant in the system must be identified (output and inputs). For example, to establish the probability of fire reaching Zone 1, i.e. ‘probability of failure of Zone 2’ because fire can spread through this zone (POF_2 in Figs 2 and 3), we identified three input variables associated with the quality of the ‘management of ornamental, artificial and wildland fuels in Zone 2’ (MANAGEMENT ORN_2, MANAGEMENT ART_2, MANAGEMENT WLD_2 in Figs 2 and 3, respectively). These variables must then be fuzzified, meaning that they need to be defined as fuzzy sets by identifying their universe of discourse (e.g. ‘complying rules percentage’, from 0 to 100%) and by selecting a set of linguistic terms (i.e. fuzzy subsets) that accurately describe them (e.g. ‘good’ and ‘bad’ are fuzzy subsets of the fuzzy sets denoted as ‘management of ornamental, artificial and wildland fuels in Zone 2’). Subsequently, a membership function for each fuzzy subset must be defined to quantify the degree of belonging of any value in the universe of discourse to each fuzzy subset (FUZZIFICATION block in Fig. 3) (Sivanandam et al. 2007). Then the inferring process is performed by using a set of rules that connect antecedents (input variables) with the consequent (output variable). These rules usually have a structure such as: ‘IF …, THEN …’. An aggregation process is required to take into account the different rules that activate according to the inputs. Then, because the output is also defined as a fuzzy set, a defuzzification process (centroid calculation) is necessary in order to transform the fuzzy results into a precise output.
Generic fuzzy inference process associated with the probability of fire reaching Zone 1, i.e. ‘probability of failure of Zone 2’ (POF_2 in Fig. 2). MANAGEMENT ORN_2, MANAGEMENT ART_2, MANAGEMENT WLD_2 refer to the management of ornamental, artificial and wildland fuels in Zone 2, respectively (based on Darbra et al. 2008).
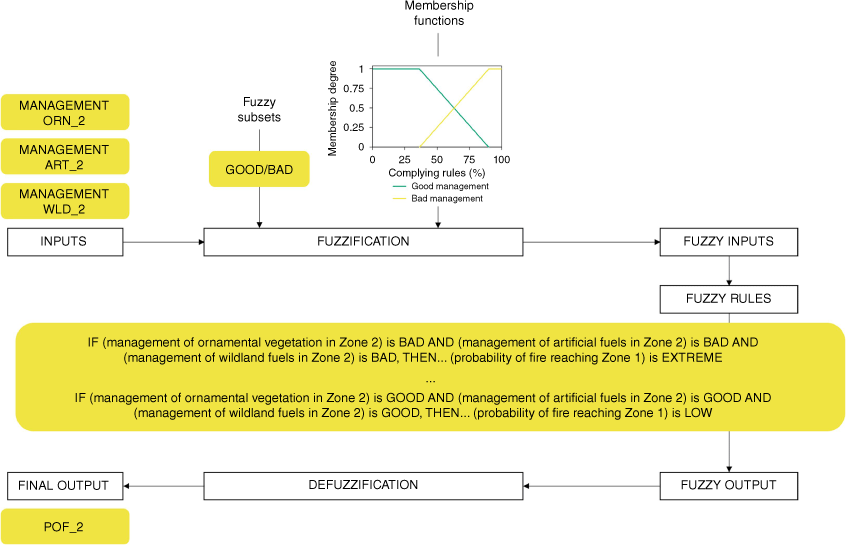
A very simple but complete example on how fuzzy inference works is given as Supplementary material. This case is about how to infer the probability of failure associated with the roof for one of the dwellings included in the first case study presented in this paper. A Python library for fuzzy logic (simpful) (Spolaor et al. 2020) was used to handle all the inference systems defined in the present work.
Parameterisation of the FISs
A poll for WUI fire experts was prepared to parametrise the FISs present in the model. A pre-selection of possible pollees was done according to their WUI expertise either because of their professional experience as WUI fire risk managers or because of their research activity evidenced by scholarly output. Then, the poll was delivered by email using an Excel file specifically designed for this purpose (available as Supplementary material). Other expert knowledge elicitation techniques incorporate group activities, such as the Delphi-like approach (Drescher and Edwards 2018). In our case, to minimise the volunteering time required from the experts, this type of approach was not considered. All the replies were evaluated using simple statistical approaches and results were validated taking into account whether the elicited outcomes were plausible and conformed to authors’ expectations (Drescher et al. 2013).
More specifically, the experts’ input helped to determine membership functions, as they were asked to provide a lower and upper bound, or a best estimate for the fuzzy subsets that defined each fuzzy set present in the model. The percentage of experts that considered the different values for each fuzzy subset was computed and then weighted average values were calculated to set membership functions. For a given fuzzy set (e.g. thickness of the glazing systems), triangular membership functions were defined for those fuzzy subsets that were not at the limits of the universe of discourse of the fuzzy set (e.g. medium thickness of the glazing systems), and trapezoidal functions for those at the limits (e.g. thin and thick glazing systems).
Additionally, the poll included the fuzzy rules, for which the experts could choose the linguistic value of the output variable (i.e. consequent). When more than one input variable was combined in a rule, the logical operator AND was used. An example of the selection for the rules’ consequent is shown in Fig. 4. To elicit each rule consequent (e.g. ‘If windows thickness is thin and shutters protection is PVC, then probability of failure is …’), a weighted average was calculated by assigning a number to each subset (probability of failure: Extreme, 4; High, 3; Medium, 2; Low, 1). Then, data were linearly transformed so that the result was in the range 0–1 and categorical values were set back according to the following ranges: [0, 0.25], Low; [0.25, 0.50], Medium; [0.50, 0.75], High; [0.75, 1], Extreme.
Homeowners questionnaire
The model presented previously has to be used together with a questionnaire that homeowners must fill in. There are different types of questions in the questionnaire: some are yes/no, others are percentages, others are multiple choice, etc. They include all the information needed to run the vulnerability assessment model for a specific property. The complete questionnaire is available as Supplementary material.
Once the questionnaire is completed, the replies are introduced into the model to calculate the probabilities of failure of the different elements considered, which are then used to calculate the probability of a fire entering a dwelling.
Results
Data required to parameterise the fuzzy inference systems present in the vulnerability assessment model were collected from experts and the outcomes obtained are described in this section. Afterwards, to evaluate whether the VAT can assess the vulnerability of dwellings characteristic of the Mediterranean WUI, the tool is tested using information gathered from houses affected by two fires that took place in Spain during the summers of 2021 (Lloret de Mar fire) and 2022 (Pont de Vilomara fire).
FIS parameterisation outcomes
The poll for WUI fire experts that was prepared to parametrise the fuzzy inference systems present in the model was completed by 13 experts who, according to personal data gathered through the questionnaire, came from six different countries: Spain (6), Australia (3), Chile (1), France (1), Portugal (1) and UK (1), and were working in civil defence (1), private companies (2) or research institutions (10).
In Fig. 5, an example of the procedure followed to define the membership functions of the fuzzy subsets that define two fuzzy sets of the model (‘window coverage’ and ‘probability of failure’) is shown. Poll replies (Fig. 5 top subplots) were used to specifically set these functions by taking into account the percentage of experts that considered the different values for each fuzzy subset (Fig. 5 middle subplots). Weighted average values were calculated (i.e. dashed vertical lines in Fig. 5 middle subplots) and vertices could be set accordingly to define membership functions (e.g. Fig. 5 bottom subplots).
Fuzzy subsets definition for ‘window coverage in the semi-confined space’ and ‘probability of failure’ fuzzy sets according to poll results. Top plots: answers given by the 13 experts. Middle plots: poll results (dashed lines indicate weighted averaged values). Bottom plots: fuzzy membership functions elicited according to middle plot results for the corresponding fuzzy subsets. SCS, semi-confined space.
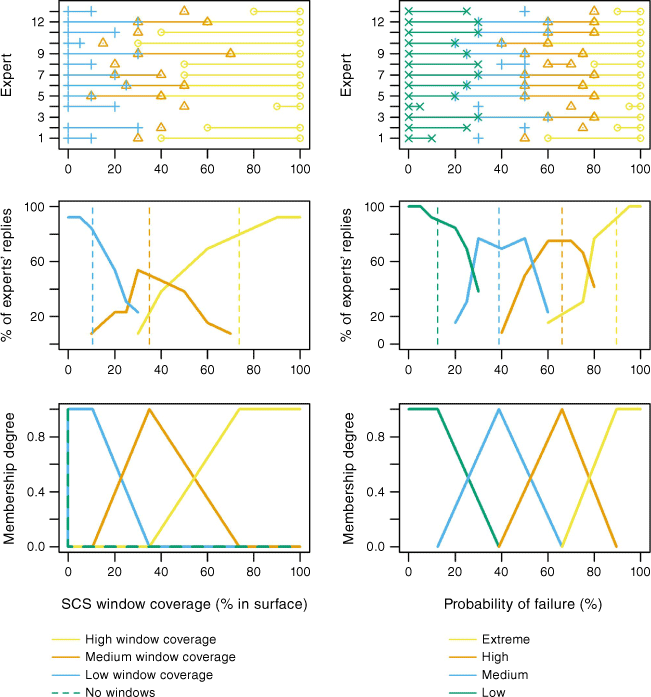
In Table 3, for each fuzzy set (i.e. first column in Table 3), specific weighted average values obtained from poll replies are shown. One specific result worth mentioning is related to the probability of failure associated with the vents. Experts considered that having non-combustible vents in bad condition gives a slightly higher probability of failure than having vents made out of combustible material (e.g. plastic).
Fuzzy sets | Description | Fuzzy subsets | ||||
Shutters | POF according to the type of shutter material | No shutters | PVC shutters | Wood shutters | Aluminium shutters | Fire-rated shutters |
Probability of failure (%) | 88.4 | 66.5 | 47.8 | 24.3 | 6.6 | |
Glazing systems | Thickness of glazing systems | Thin | Medium | Thick | ||
Thickness (mm) | 4.7 | 9.6 | 16.4 | |||
Roof material | Probability that a fire can affect the underlying roof structure owing to the type of roof covering material | Combustible | Non-combustible | |||
Probability of roof being affected (%) | 66.2 | 15.7 | ||||
Roof maintenance | Probability of a fire affecting the complete roof structure owing to maintenance performed | Badly maintained | Well maintained | |||
Probability of roof being affected (%) | 75.7 | 16.5 | ||||
Vents | Probability that a fire can enter the dwelling through vents owing to the type and condition of vent protection | Non-combustible, good-condition vent protection | Combustible vent protection | Non-combustible, bad-condition vent protection | No vent protection | |
Probability of fire entering (%) | 7.8 | 40.2 | 42.5 | 81.1 | ||
Windows in semi-confined space | Percentage of area of walls shared by a house and an SCS that is occupied by windows | High | Medium | Low | ||
Coverage (%) | 74.3 | 35.5 | 10.2 | |||
Envelope type in SCS | Probability of structural failure that each type of dwelling envelope could experience if exposed to heat accumulation from a fire in an SCS | Combustible | Non-combustible, thin (<15 mm) | Non-combustible, thick (≥15 mm) | ||
Probability of failure (%) | 78.4 | 30.0 | 8.4 | |||
Combustible material in SCS | Percentage of volume occupied by combustible materials in an SCS | High | Medium | Low | ||
Coverage (% by volume) | 61.4 | 29.0 | 6.2 | |||
Failure | Generic probability of failure | Extreme | High | Medium | Low | |
POF (%) | 89.5 | 66.1 | 38.9 | 12.4 | ||
Fuel managementA | According to a set of rules for management of fuels (all with the same weight), percentage of rules that should be complied withB | Bad | Good | |||
Complying with rules (%) | 36.2 | 90.1 |
SCS, semi-confined space; POF, probability of failure.
AThree different types of fuel are considered, i.e. wildland vegetation, ornamental vegetation, artificial fuels.
BRules are different according to the type of fuel, the defence zone of interest surrounding the dwelling (i.e. Zone 1: 10 m around the dwelling; Zone 2: 10–30-m ring around the dwelling) and the topography, as shown in Table 2.
Descriptions and/or questions posed to discriminate between the different subsets are presented in the questionnaire (available as Supplementary material). For example, the roof is assumed to be badly maintained if there are missing, displaced or broken tiles, if the underlying roof sheeting is exposed, or if there are unsealed spaces between the roof and the external walls or between the roof covering and the roof decking. Also, if a regular cleaning of debris piling up on the roof or gutters is not done, the roof is assumed to be badly maintained.
Another result of the poll was the consequent of the fuzzy rules associated with each fuzzy inference system. An example of the results obtained for several rules’ consequents of FIS1 (defined in Fig. 2) is shown in Fig. 6. The plot shows the distribution of replies given by the experts to the five rules of FIS1 associated with the presence of thin glazing systems, and on the right side of this figure, the final consequent assigned to each rule is shown.
Number of replies given by experts to several rules’ consequents of the fuzzy inference system associated with the ‘probability of failure of the glazing systems’ (i.e. FIS1 in Fig. 2). The subset finally assigned is shown on the right side of the plot.
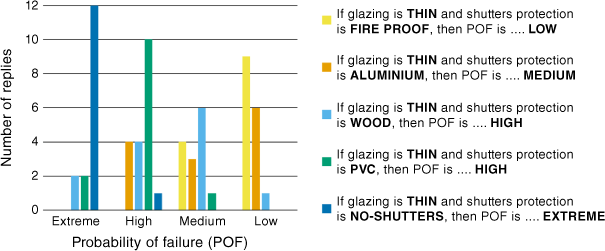
The model was constructed without considering different weights for the variables involved in each fuzzy inference system explicitly. An analytical hierarchy process could have been applied to acknowledge the different importances; however, according to the results obtained, we believe that experts are considering different weights implicitly when establishing rules consequents. For example, consequents defined according to experts’ responses for the three rules shown in Table 4 indicate that the probability of fire reaching Zone 1 is larger when wildland fuels are badly managed in comparison with badly managed ornamental vegetation or artificial fuels in Zone 2.
IF | Management of ornamental vegetation in Zone 2 is | AND | Management of artificial fuels in Zone 2 is | AND | Management of wildland fuels in Zone 2 is | THEN | Probability of fire reaching Zone 1 is |
BAD | GOOD | GOOD | MEDIUM | ||||
GOOD | BAD | GOOD | MEDIUM | ||||
GOOD | GOOD | BAD | HIGH |
Case study 1: Lloret de Mar, Spain (2021)
The owners of five dwellings (Fig. 7) affected by a WUI fire that took place in July 2021 in Lloret de Mar, Spain (Fig. 8) were interviewed during a working session that was organised several months after the fire in collaboration with the city council. During that session, clear instructions were given for the evaluation of properties as they were before the fire. The homeowners were very collaborative and were able to fill in the questionnaire with our help. Their answers (shown in Table 5) were used to quantify the probability of fire entering their dwellings using our VAT model (Table 6). They got our feedback on the different issues (fuel management, structural constraints, etc.) that needed to be addressed in order to reduce this probability. For comparison purposes, Tables 5 and 6 also include a hypothetical worst-case scenario, for which the worst characteristics of a dwelling and of fuel management are considered and the highest probabilities of failure are estimated.
Monitored properties (H11 to H15) affected by the WUI fire in Lloret de Mar, Spain (July 2021) (Google Earth, earth.google.com). Red line: 10 m around dwellings; yellow line: 30 m around dwellings. Green lines indicate elevation contours (Institut Cartogràfic i Geològic de Catalunya, https://www.icgc.cat/). The location of the burning van that initiated the upslope fire is shown.
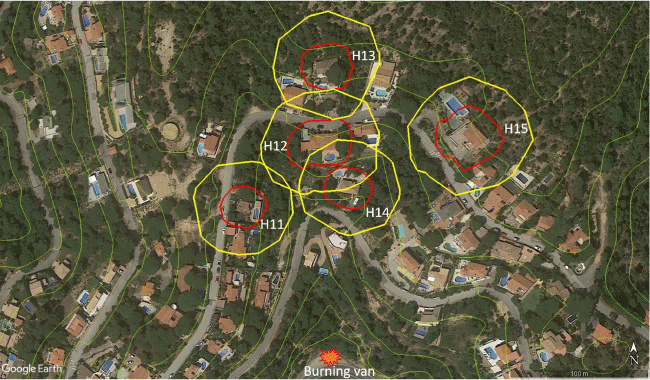
Photographs of the WUI fire in Lloret de Mar, Spain (July 2021): (a) burning van that initiated the upslope fire (O. Torres); (b) general view of the passage of the fire (Bombers de la Generalitat); photos of the dwellings after the fire: (c) H11; (d) H12; (e) H13; (f) H14; (g) H15.
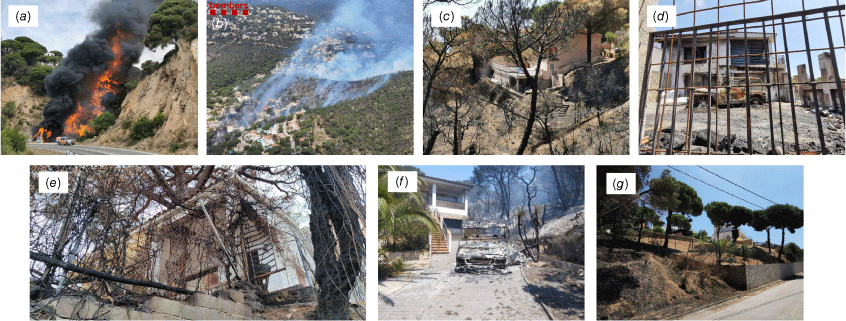
Characteristics | Worst-case hypothetical dwelling | WUI fire dwellings | ||||
H11 | H12 | H13 | H14 | H15 | ||
Shutters | No shutters | Wood | PVC (no shuttersB) | PVC | Aluminium | Aluminium |
Glazing thicknessA (mm) | 3 | 14 | 14 | 4 | 14 | 14 |
Roof material | Combustible | Non-combustible | Non-combustible | Non-combustible | Non-combustible | Non-combustible |
Roof maintenance | Bad | Good | Good | Bad | Good | Good |
Vents | Non-protected vents | None | None | Non-protected vents | None | None |
Windows coverage in SCS (%) | 100 | 20 | 0 | 50 | 70 | 70 |
Envelope type in SCS | Combustible walls | Non-combustible and thick | Non-combustible and thin | Non-combustible and thick | Non-combustible and thick | Non-combustible and thick |
Combustible material coverage in SCS (% by volume) | 100 | 10 | 70 | 20 | 10 | 10 |
Fuel management compliance in Zone 2 O/A/W (%) | 0/0/0 | 50/50/0 | 67/50/0 | 25/100/0 | 100/100/0 | 100/50/0 |
Fuel management compliance in Zone 1 O/A/W (%) | 0/0/0 | 57/100/100 | 56/25/0 | 11/25/0 | 44/100/17 | 86/25/100 |
O/A/W, Ornamental/Artificial/Wildland fuels; SCS, semi-confined space; H##, monitored dwellings as shown in Fig. 7.
AGlazing thickness was assumed to be 14 mm (4 mm glass + 6 mm air + 4 mm glass) if there were double panes and 4 mm if the glazing pane was single.
BDuring the fire, PVC shutters were open and as such did not provide any protection.
FIS/classical logic results (%) | Worst-case hypothetical dwelling | WUI fire dwellings | ||||
H11 | H12 | H13 | H14 | H15 | ||
POF_GLAZING | 88 | 39 | 48 (70A) | 65 | 16 | 16 |
POF_ROOF | 88 | 14 | 14 | 65 | 14 | 14 |
POF_VENT | 88 | 0 | 0 | 88 | 0 | 0 |
POF_ENVELOPE | 88 | 39 | 14 | 39 | 39 | 39 |
POF_SEMI-CONFINED | 88 | 21 | 41 | 32 | 21 | 21 |
POF_2 | 88 | 79 | 77 | 88 | 65 | 79 |
POF_1 | 88 | 54 | 75 | 88 | 63 | 66 |
POF of the dwelling | 100 | 59 | 74 (85A) | 99 | 42 | 42 |
Probability of fire entrance | 88 | 32 | 55 (63A) | 87 | 27 | 28 |
FIS, fuzzy inference system; POF, probability of failure; _1, Zone 1 (10 m around the dwelling); _2, Zone 2 (10–30-m ring around the dwelling).
AValues obtained considering no shutters, as PVC shutters were open during the fire.
The WUI fire under study started when a damaged van that had stopped on the hard shoulder of a road (Figs 7 and 8a) caught fire at ~11 a.m. with a sea breeze blowing. The flames from the van ignited the vegetation next to it, which was mainly composed of pine trees and a dense understorey. Topography and fuel continuity played an important role in the propagation and intensity of the fire. It spread rapidly upwards through a steep slope until fuel discontinuity due to the presence of houses triggered the main fire front to propagate following the left drainage area in between H11, and H12 and H14 (see in Fig. 7 the ‘V’-shaped contour lines pointing uphill).
This fire prompted evacuation orders and several homes were threatened, various elements present outdoors were burned (e.g. vehicles, fences and garden furniture), and one house was severely damaged (H12 in Figs 7 and 8d) owing to the fire entering the dwelling as a result of the breakage of a window pane.
All monitored houses were built with non-combustible materials such as concrete, stone or bricks. H11, H14 and H15 were built between 10 and 30 years ago, whereas H12 and H13 were over 30 years old. All homeowners stated that their house was well maintained when the fire occurred.
As can be seen in Table 5, all houses had shutters of different materials, but none had fire-rated ones. Moreover, H12 had PVC shutters but during the fire they were left open and as such did not provide any protection; therefore, both situations (i.e. no shutters and PVC shutters) were considered for the vulnerability assessment. All but H13 also had double glazing systems (a thickness value of 14 mm was assumed for this type of systems). As for the roof, all had a construction typical of Mediterranean houses, with a roof covering made out of clay tiles. All roofs, with the exception of the one of H13, were stated to be in good condition. H13 was also the only dwelling to have vents, which at the time of the fire were not protected. All houses had porches with different types of outdoor furniture. H12 also had a car shed made out of wood and clay tiles that was completely destroyed by the fire. When it comes to the two zones analysed surrounding the dwellings, it can be seen that there was no compliance at all with the rules for the management of wildland fuels in Zone 2, while only two houses complied with these rules completely in Zone 1. With regard to ornamental vegetation, all properties complied with at least some of the rules, with a minimum of 11% compliance for Zone 1 of H13. None of the dwellings reached 100% compliance in Zone 1, and it can be seen that, overall, compliance was higher in Zone 2. For the presence of artificial fuels, the lowest compliance was 25% in both zones, and H14 was the only property that fully complied in both zones with the rules set for artificial fuels.
According to information provided in Tables 5 and 6, the dwellings with the highest probability of fire entrance were H13 (87%) and H12 (63% considering no shutters). The probability value associated with H13 is quite large if we consider the values obtained for the other dwellings and that the worst value attainable using this method is 88% (see Table 6). H13 presented the highest probability of failure of the dwelling itself (99%). Vulnerable elements in the structure of H13 were the shutter material, glazing thickness and vent design. Moreover, regarding the probability of fire reaching the dwelling (POF_1), H13 had a value of 88%. This value was high because there was no compliance with the rules for management of wildland fuels in both zones and compliance was low for ornamental vegetation.
H12 had also a high probability of fire reaching the dwelling (75%) because compliance with fuel management rules was low in both zones (as can be seen in Table 5, no fuel type management section reached a compliance value of 100%). H12 presented a probability of failure of the dwelling itself of 74 or 85% (depending on consideration of shutters or not). Vulnerable elements in the H12 structure were shutter material and the presence of an SCS with a high amount of combustible fuel (the car was parked in the car shed when the fire occurred).
Of these two houses (H12 and H13) with the highest probability of fire entering, H13 suffered minor damages outside the house, while the fire entered H12. This is probably because fire behaviour was more intense and rapidly spreading in the drainage area between H11, and H12 and H14 (see elevation contours in Fig. 7). In contrast, fire was propagating more slowly in the area around H13 because it was not channelled there. Also, when the wildfire was threatening the area, people living in H12, who were at that moment inside the house, evacuated and did not close windows or PVC shutters nor placed their vehicle inside the garage. The car was located close to the porch as well as close to a window. These actions left artificial fuel (the car) available for burning and made windows more vulnerable. If evacuation had been well prepared, the probability of fire entering this house would have been lower (55% probability had the shutters been pulled down). In contrast, H13 was locked (shutters closed) because the residents were not at home that day.
This indicates that, although the house that was severely damaged was not the most vulnerable in the area, it was affected by fire owing to a badly prepared evacuation. Thus, it is important to keep in mind that houses will have a better chance of surviving a wildfire if people living in the WUI are well prepared and informed about the actions that need to be undertaken before evacuating.
Case study 2: El Pont de Vilomara, Spain (2022)
Six houses from the River Park residential development in El Pont de Vilomara, Spain, (Fig. 9) were also used to test the VAT tool. They were affected by a WUI fire that took place in July 2022 (Fig. 10), prompting evacuation orders and affecting many dwellings. Five of these houses were located on the same street, on the hilltop of Turó de Solanes (H21, H22, H23, H25, H26). Another was located further down on the same slope (H24). The main characteristics of the dwellings considered for this study are given in Table 7. These data were obtained through interviews with the homeowners performed informally after the fire, and also by inspection of photographs that were made available by the firefighters.
Monitored properties (H21 to H26) affected by the WUI fire in El Pont de Vilomara, Spain (July 2022) (Google Earth, earth.google.com). Red line: 10 m around dwellings; yellow line: 30 m around dwellings. Green lines indicate elevation contours (Institut Cartogràfic i Geològic de Catalunya, https://www.icgc.cat/).
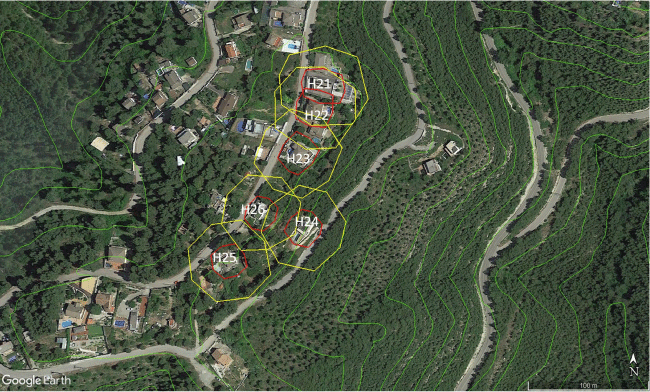
Photographs of the WUI fire in El Pont de Vilomara, Spain (July 2022): (a) overview of the area after the fire; (b) H21; (c) H22; (d) H23; (e) H24; (f) H25; (g) H26. Source: S. Boixader and Bombers de la Generalitat.
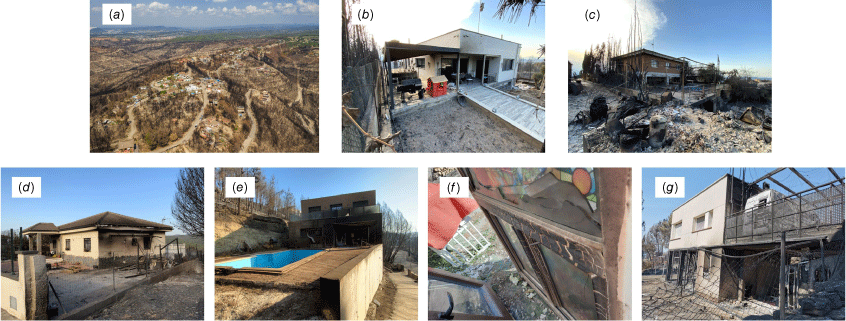
Characteristics | WUI fire dwellings | |||||
H21 | H22 | H23 | H24 | H25 | H26 | |
Shutters | Aluminium | No shutters | No shutters | No shutters | No shutters | No shutters |
Glazing thicknessA (mm) | 14 | 14 | 14 | 14 | 14 | 14 |
Roof material | Non-combustible | Non-combustible | Non-combustible | Combustible | Non-combustible | Non-combustible |
Roof maintenance | Bad | Good | Good | Good | Good | Bad |
Vents | Non-combustible in good conditions | Non-combustible in good conditions | Non-combustible in good conditions | Non-combustible in good conditions | Non-protected vents | Combustible |
Windows coverage in semi-confined space (%) | 80 | 5 | 25 | 70 | NA | 0 |
Envelope type in SCS | Non-combustible and thick | Non-combustible and thick | Non-combustible and thick | Non-combustible and thick | NA | Non-combustible and thick |
Combustible material coverage in SCS (% by volume) | 20 | 5 | 5 | 30 | NA | 50 |
Fuel management compliance in Zone 2 O/A/W (%) | 25/50/0 | 33/0/0 | 25/0/0 | 100/100/0 | 100/50/0 | 0/0/0 |
Fuel management compliance in Zone 1 O/A/W (%) | 44/75/100 | 44/75/100 | 67/50/33 | 100/75/17 | 86/50/60 | 57/25/33 |
O/A/W, Ornamental/artificial/wildland fuels; SCS, semi-confined space; NA, not applicable; H##, monitored dwellings as shown in Fig. 7.
AGlazing thickness was assumed to be 14 mm (4 glass + 6 air + 4 glass) if there were double panes and 4 mm if the glazing pane was single.
The fire propagated following seawinds channelled through the Llobregat river valley. The River Park residential development was positioned over the main propagating axis of the fire that impacted on this development only 2 h after its start. Owing to the location of the residential development (i.e. on top of the hill), several dwellings received the impact of the fire simultaneously. Moreover, although there were treated fringes mid-slope (see Fig. 9), they were not effective and the fire was propagating through the pine tree crowns.
According to Table 7, all houses had double-pane glazing systems and vents. However, most of the houses did not have shutters for protecting all the windows (except H21), and combustible or non-protected vents were observed in two of the houses (H26 and H25, respectively). Roofs were in general well maintained and all, except H25, had a semi-confined space with thick and non-combustible walls connecting the house. The percentage by volume occupied by combustible material in the SCSs was lower than 50% and the largest value was observed in H26 (50%). Two houses had a very high percentage of window coverage in the SCS (80% H21 and 70% H24). Regarding fuel management in the two zones surrounding the house, it can be seen that, as for the houses presented in Table 5, there was no compliance at all with the rules for the management of wildland fuels in Zone 2. However, in Zone 1, two houses (H21 and H22) complied with these rules completely. Regarding ornamental vegetation, all properties complied with at least 44% of the rules in Zone 1. For artificial fuels, the compliance level in Zone 1 was larger than 25%.
The results obtained using the VAT model are shown in Table 8. The dwellings with the highest probability of fire entrance were H26 (81%) and H23 (67%). For the other dwellings, the estimated probability was lower than 60%.
FIS/classical logic results (%) | WUI fire dwellings | |||||
H21 | H22 | H23 | H24 | H25 | H26 | |
POF_GLAZING | 16 | 70 | 70 | 70 | 70 | 70 |
POF_ROOF | 65 | 14 | 14 | 65 | 14 | 65 |
POF_VENT | 14 | 14 | 14 | 14 | 88 | 65 |
POF_ENVELOPE | 39 | 0 | 39 | 39 | 0 | 14 |
POF_SEMI-CONFINED | 32 | 14 | 14 | 40 | 0 | 35 |
POF_2 | 87 | 88 | 88 | 65 | 79 | 88 |
POF_1 | 70 | 71 | 83 | 63 | 58 | 83 |
POF of the dwelling | 83 | 81 | 81 | 95 | 97 | 98 |
Probability of fire entrance | 56 | 57 | 67 | 59 | 57 | 81 |
FIS, fuzzy inference system; POF, probability of failure; _1, Zone 1 (10 m around dwellings); _2, Zone 2 (10–30-m ring around dwellings).
Vulnerable elements in H26 were the unprotected glazing systems, the bad condition of the roof and the combustible vents. With regard to the probability of fire reaching the dwelling (POF_1), H26 had a value of 83%. This value was high because there was no compliance with the rules for the management of fuels in Zone 2, and the compliance was low (less than 60%) in Zone 1.
H23 presented a probability of fire reaching the dwelling (POF_1) similar to that obtained for H26, but the probability of failure of the dwelling itself was lower (81% vs 98%, respectively), resulting in a lower overall probability of fire entrance (67% vs 81%, respectively). The vulnerable elements identified for this dwelling were different, the most important being the presence of unprotected glazing systems and a moderate window coverage of the SCS (25% according to Table 7).
The fire affected H24 and H26 severely (see Fig. 10), both aligned with the slope of the terrain and next to absentee-owned parcels on two sides of the house aligned with fire propagation. According to the observed damage, the fire probably entered through breakage of the glazing systems. The results obtained with VAT showed that the probability of fire entering H24 was lower compared with H26 but still almost 60%, so we were able to determine that the degree of vulnerability of H24 was high.
Discussion
The methodology presented aimed at quantifying the vulnerability of dwellings at the WUI due to a fire entering them, as well as to point out the critical issues in the dwelling itself and those present on the property (within a 30-m radius).
In this regard, according to Valis and Pietrucha-Urbanik (2014), vulnerability can be defined as ‘the probability of the consequence occurring given an event’. In this case, we can reword this definition and state that the vulnerability assessment we performed refers to the probability of fire entering the dwelling given a set of characteristics of the house and its surroundings. The task of formalising the set of characteristics was deemed complex owing to uncertainty. However, as fuzzy logic is appropriate to model the vagueness and uncertainty of human expressions that have no precise boundaries (e.g. bad or good; thick or thin; high or low), it has been used instead of discrete approaches whenever possible. The way probabilities of failure were derived was based on the knowledge of 13 polled experts. This means that the outputs obtained using the VAT methodology rely heavily on human expert knowledge.
The authors are aware of another methodology, developed by Papathoma-Köhle et al. (2022), for the calculation of a vulnerability index (PVI) for dwellings located at the WUI. Those authors based their work on analysis of the dwellings affected by the Mati fire (Greece) in 2017. They identified eight indicators (roof material, structural type, slope, vegetation, roof leaf accumulation, shutter material, main ground covering, roof type) for the assessment of physical vulnerability related to building characteristics and surroundings. They constructed the index as a composite of the eight indicators, which were scored (1–5; 5 = worst in terms of vulnerability) and added according to their relative importance.
While these eight indicators are included in the events presented in this paper, indicators for vents, glazing thickness and SCSs are missing. Additionally, the maintenance level of the roof is not taken into account, while the shape of the roof is. In our view, these are important shortcomings as these building elements have been critical in home destruction in past WUI fire events in the Mediterranean region (Vacca et al. 2020b). Moreover, in terms of fuel availability near the dwelling, only vegetation within 20 m of the structure is considered. Artificial fuels (commonly found in WUI properties as well) are not considered, and neither is fire spread through the different types of fuels due to poor fuel distancing.
In addition, as stated by the authors, the indicators used to develop the index were ‘only those relevant to the dominant architectural style of the area’ and those that it was possible to collect after the fire, when ‘many features were already destroyed’. This methodological step prevents this tool from being directly applicable to other Mediterranean areas.
When applying the methodology presented by Papathoma-Köhle et al. (2022) to the Lloret de Mar case study, it was noticed that all the houses had the same value for all indicators, with the exception of the one for the shutters, which were made out of aluminium for houses H14 and H15, whereas in the other houses, they were made out of combustible materials such as wood or PVC. The PVI obtained for H11, H12 and H13 is of 2.84 out of 5, whereas houses H14 and H15 obtained a PVI of 2.72. Based on the results obtained using the VAT methodology presented here, a larger variability was observed between the analysed dwellings. For example, H11, H12 and H13 had different scores, ranging from 32 to 87. Therefore, we believe that the presented tool is more comprehensive regarding the different issues and vulnerabilities of dwellings and properties at the WUI (specifically within the Mediterranean regions).
Looking at the results from the two case studies, it is clear that it is not only the dwelling that plays a role, but the surrounding elements are also deemed to be very important. In this regard, it must be noted that the experts considered that good management of the surroundings consists of complying with 90.1% of the set rules. Moreover, it is important to note that, if we want to correlate vulnerability with house damage, there are many factors to take into account (e.g. firefighters actions (yes/no), evacuation/stay and defend procedures, position of the house with respect to the propagating front). The difficulty in obtaining this kind of relationship was presented by Dossi et al. (2022).
According to the results obtained for the worst-case hypothetical scenario (see Table 6), the methodology presented in this paper gives a maximum value of 88% for the probability of fire entering a dwelling. Mathematically, this is due to the fact that the ‘extreme’ fuzzy subset associated with the ‘probability of failure’ fuzzy set is defined as a trapezoidal function. However, this is still reasonable because 100% probability would mean that, according to our model, it is certain that the fire will enter the dwelling. However, as mentioned before, there are other factors (e.g. those related to emergency response) that prevent us from being able to ensure that maximum probability.
However, in order to validate the proposed methodology, more case studies should be analysed. This will also give a broader and better understanding of the presented tool, and highlight areas or issues that might not yet have been considered. The case studies required to validate the tool have to be carefully detailed because pre-fire relevant data from homeowners need to be collected. For this reason, databases of past large fires, such as the Cal Fire (DINS) database or the Pedrógrão Grande Fire Complex database, those used in Dossi et al. (2022), are not appropriate for this purpose.
The authors foresee a two-fold evolution for this tool, which could be used as a simple checklist for homeowners as well as part of an advanced home assessment program, linked to an EU genuine risk awareness and preparedness framework tailored for WUI communities in the Mediterranean region (inspired by FireSmart in Canada and Firewise in the USA). To this end, further work includes the implementation of the tool (i.e. questionnaire for the homeowners and vulnerability model calculation) in digital form (e.g. mobile app, web service). This entails a careful consideration of several methodological aspects (e.g. design and layout, communication, diffusion channels, data management) that have to be tackled through a multi-disciplinary approach involving both engineering and social sciences expertise.
Conclusions
The tool we have developed (i.e. VAT) considers the complex nature of the WUI microscale fire risk problem through the use of fuzzy logic. The end users of this tool are intended to be not only fire safety practitioners, but also homeowners and residents of the WUI. The use of this VAT is expected to lead to the improvement of fire safety practices at the microscale; i.e. it will help to increase the WUI fire risk awareness of homeowners by systematically identifying major problematic conditions present on their property and in its surroundings. Although building the tool is complex, the final product that is presented to the user is straightforward and easy to use. The tool considers four main building sub-systems that have proved to be vulnerable to fire, as well as three different types of fuels that can cause the fire to spread through a property towards a dwelling. As probabilities are calculated for each of these intermediate events, it is possible not only to obtain the probability of a fire entering the dwelling, but also to identify the critical events that lead to this. The homeowner will also obtain information on what the most critical issues of their property are (i.e. which event leads to a higher probability of fire entrance), and therefore which elements need to be improved to prevent fire entrance in the event of a wildfire. We foresee a systematic use of this tool through an EU global risk awareness program that has to be key for improving fire preparedness and protection in Mediterranean WUI vulnerable communities.
Data availability
The data that support this study will be shared on reasonable request to the corresponding author.
Acknowledgements
The authors would like to acknowledge all the experts who answered the questionnaire required to parameterise the fuzzy model, and all the homeowners who replied the questions about their property and the actions they took during the fire that affected their homes. We are also grateful to all the firefighters who helped us to gather information about the behaviour of the two fires and about the dwellings under study. Special thanks to R. Sauquet. We also would like to thank the reviewers for their helpful suggestions and comments. A. Àgueda is a Serra Húnter fellow.
References
Autoridade Florestal National (2008) Gestão de Combustíveis Para Protecção de Edificações - Manual. Available at https://fogos.icnf.pt/sgif2010/InformacaoPublicaDados/gestao_comb_final.pdf [accessed 30 June 2022] [In Portuguese]
Cal Fire (2022) Defensible Space. Available at https://www.fire.ca.gov/programs/communications/defensible-space-prc-4291/
Calkin DE, Cohen JD, Finney MA, Thompson MP (2014) How risk management can prevent future wildfire disasters in the wildland–urban interface. Proceedings of the National Academy of Sciences 111, 746-751.
| Crossref | Google Scholar |
Caton SE, Hakes RSP, Gorham DJ, Zhou A, Gollner MJ (2017) Review of Pathways for Building Fire Spread in the Wildland–Urban Interface Part I: Exposure Conditions. Fire Technology 53, 429-473.
| Crossref | Google Scholar |
Cohen JD (2000Available at https://www.fs.usda.gov/rm/pubs_other/rmrs_2000_cohen_j002.pdf [accessed 30 June 2022]) Preventing Disaster: Home Ignitability in the Wildland–Urban Interface. Journal of Forestry 98, 15-21.
| Google Scholar |
Darbra RM, Demichela M, Murè S (2008) Preliminary risk assessment of ecotoxic substances accidental releases in major risk installations through fuzzy logic. Process Safety and Environmental Protection 86, 103-111.
| Crossref | Google Scholar |
Dossi S, Messerschmidt B, Ribeiro LM, Almeida M, Rein G (2022) Relationships between building features and wildfire damage in California, USA, and Pedrógão Grande, Portugal. International Journal of Wildland Fire 32(2), 296-312.
| Crossref | Google Scholar |
Drescher M, Edwards RC (2018) A systematic review of transparency in the methods of expert knowledge use. Journal of Applied Ecology 56(2), 436-449.
| Crossref | Google Scholar |
Drescher M, Perera AH, Johnson CJ, Buse LJ, Drew CA, Burgman MA (2013) Toward rigorous use of expert knowledge in ecological research. Ecosphere 4(7), 83.
| Crossref | Google Scholar |
FireSmart Canada (2020) FireSmart Home Ignition Zone Assessment Score Card. Available at https://firesmartbc.ca/wp-content/uploads/2021/04/FSC-CRI-Home-Ignition-Zone-Assessment-Score-Card-Fillable.pdf
Ganteaume A, Barbero R, Jappiot M, Maillé E (2021) Understanding future changes to fires in southern Europe and their impacts on the wildland–urban interface. Journal of Safety Science and Resilience 2, 20-29.
| Crossref | Google Scholar |
Generalitat de Catalunya (2005) Decret 123/2005, de 14 de juny, de mesures de prevenció dels incendis forestals en les urbanitzacions sense continuïtat immediata amb la trama urbana. Available at https://portaljuridic.gencat.cat/ca/document-del-pjur/?documentId=384053 [In Catalan]
Generalitat de Catalunya (2019) Protegeos de los incendios forestales. Pla INFOCAT. Available at https://interior.gencat.cat/web/.content/home/030_arees_dactuacio/proteccio_civil/consells_autoproteccio_emergencies/incendi_del_bosc/documents/llibret_incendis_forestals_cast.pdf [In Spanish]
Government of Alberta (2013) FireSmart Guidebook for Community Protection: A Guidebook for Wildland/Urban Interface Communities. Available at http://wildfire.alberta.ca/firesmart/documents/FireSmart-GuideCommunityProtection-Nov2013.pdf
Hakes RSP, Caton SE, Gorham DJ, Gollner MJ (2017) A Review of Pathways for Building Fire Spread in the Wildland–Urban Interface Part II: Response of Components and Systems and Mitigation Strategies in the United States. Fire Technology 53, 475-515.
| Crossref | Google Scholar |
International Code Council (2021) International Wildland–Urban Interface Code (IWUIC). Available at https://codes.iccsafe.org/content/IWUIC2021P1
Intini P, Ronchi E, Gwynne S, Bénichou N (2019) Guidance on Design and Construction of the Built Environment Against Wildland–Urban Interface Fire Hazard: A Review. Fire Technology 56, 1853-1883.
| Crossref | Google Scholar |
Legifrance (2021) Code forestier. Available at https://www.legifrance.gouv.fr/codes/section_lc/LEGITEXT000025244092/LEGISCTA000025245889/#LEGISCTA000025248647 [In French]
Manca T, López M (2014) ‘Guía Metodológica de Actuaciones de Prevención, Defensa y Autoprotección en la Interfaz Urbano-Forestal’. pp. 1–60 (Conselleria de Governació i Justícia). Available at https://agroambient.gva.es/documents/162905929/162908876/Guía+metodológica+de+Actuaciones+de+Prevención%2CDefensa+y+Autoprotección+en+la+Interfaz+Urbana-Forestal+%286%2C8Mb%29/d24a0daf-d777-405b-8938-b4a54394831f [In Spanish]
Maranghides A, Mell W, Hawks S, Wilson M, Brewer W, Brown C, Vihnanek B, Walton W (2021) A Case Study of the Camp Fire – Fire Progression Timeline. NIST Technical Note 2135. 406 pp. (US Department of Commerce). Available at https://nvlpubs.nist.gov/nistpubs/TechnicalNotes/NIST.TN.2135.pdf
Maranghides A, Link ED, Quarles SL, Gorham DJ (2022) NIST Technical Note 2205 WUI Structure/Parcel/Community Fire Hazard Mitigation Methodology (NIST). Available at https://nvlpubs.nist.gov/nistpubs/TechnicalNotes/NIST.TN.2205.pdf
Ministère de l’Écologie et du Développement durable (2002) Plans de prévention des risques naturels (PPR): Risques d’incendies de forêt. Available at https://www.ecologie.gouv.fr/sites/default/files/PPR_feux_de_foret_complet_0.pdf [In French]
Morvan D (2007) A numerical study of flame geometry and potential for crown fire initiation for a wildfire propagating through shrub fuel. International Journal of Wildland Fire 16, 511-518.
| Crossref | Google Scholar |
NFPA (2022) Prepare Your Home Wildfire Risk Reduction: Steps That Can Make Your Home Safer During a Wildfire. Available at https://www.nfpa.org//-/media/Files/Firewise/Fact-sheets/FirewiseHowToPrepareYourHomeForWildfires.pdf
Oladokun VO, Montz BE (2019) Towards measuring resilience of flood-prone communities: a conceptual framework. Natural Hazards and Earth System Sciences 19, 1151-1165.
| Crossref | Google Scholar |
Papathoma-Köhle M, Schlögl M, Garlichs C, Diakakis M, Mavroulis S, Fuchs S (2022) A wildfire vulnerability index for buildings. Scientific Reports 12, 6378.
| Crossref | Google Scholar |
Pastor E, Muñoz JA, Caballero D, Àgueda A, Dalmau F, Planas E (2019) Wildland–Urban Interface Fires in Spain: Summary of the Policy Framework and Recommendations for Improvement. Fire Technology 56, 1831-1851.
| Crossref | Google Scholar |
Préfet de Bouches-du-Rhône (2014) ‘Arrête no. 2014316-0054 relatif au débroussaillement et au maintien en état débroussaillé dans les espaces exposés aux risques d’incendies de forêt.’ (Direction départementale des Territoires et de la Mer, Service de l’Agriculture et de la Forêt) Available at http://fransylva-paca.fr/wp/wp-content/uploads/2015/08/2014-11-12-Arrêté-OLD-13.pdf [In French]
Préfet de la Haute-Corse (2022) ‘Arrête DDT2B/SEBF/FORET/No 2B-2022-04-05-00006.’ (Direction départementale des territoires, Service Eau-Biodiversité-Forêt, Unité Forêt) Available at https://www.haute-corse.gouv.fr/IMG/pdf/arrete_2b-2022-04-05-00006_du_5_avril_2022.pdf [In French]
Préfet de Vaucluse (2013) ‘Arrêté no. 2013049-0002 relatif au débroussaillement légal autour des constructions, chantiers et installations de toute nature dans le cadre de la prévention et de la protection contre les feux de forêts.’ (Direction départementale des territoires, Service Eau et Milieux naturels) Available at http://fransylva-paca.fr/wp/wp-content/uploads/2015/08/2013-02-18-Arrêté-OLD-84.pdf [In French]
Préfet des Alpes-de-Haute-Provence (2013) ‘AP 2013-1473 relatif à la prévention des incendies de forêts et des espaces naturels dans le département des Alpes-de-Haute-Provence et concernant le débroussaillement.’ (Direction Departementale des Territoires, Service de l’Environnement et des Risques) Available at http://fransylva-paca.fr/wp/wp-content/uploads/2015/08/2013-07-04-Arrêté-OLD-04.pdf [In French]
Préfet des Alpes-Maritimes (2014) ‘Arrêté no 2014-452 portant règlement permanent du débroussaillement obligatoire et du maintien en état débroussaillé dans le département des Alpes-Maritimes.’ (Direction départementale des Territoires et de la Mer des Alpes-Maritimes, Service Économie Agricole, Ruralité, Espaces naturels) Available at http://fransylva-paca.fr/wp/wp-content/uploads/2015/08/2014-06-10-Arrêté-OLD-06.pdf [In French]
Préfet du Var (2015) ‘AP portant règlement permanent du débroussaillement obligatoire et du maintien en état débroussaillé dans le département du Var.’ (Direction départementale des Territoires et de la Mer du Var) Available at http://fransylva-paca.fr/wp/wp-content/uploads/2015/08/2015-03-30-Arrêté-OLD-83.pdf [In French]
Regione Autonoma della Sardegna (2022) Prescrizioni regionali antincendi. Available at http://www.sardegnaambiente.it/documenti/19_173_20220505140518.pdf [In Italian]
Regione Piemonte (2021) Piano regionale per la programmazione delle attività di previsione, prevenzione, e lotta attiva contro gli incendi boschivi. Available at https://www.regione.piemonte.it/web/sites/default/files/media/documenti/2021-03/piano_aib_2021_2025.pdf [In Italian]
Seguí X, Pujolasus E, Betrò S, Àgueda A, Casal J, Ocampo-Duque W, Rudolph I, Barra R, Páez M, Barón E, Eljarrat E, Barceló D, Darbra RM (2013) Fuzzy model for risk assessment of persistent organic pollutants in aquatic ecosystems. Environmental Pollution 178, 23-32.
| Crossref | Google Scholar |
South Australia Country Fire Service (2022) ‘Your guide to Bushfire Safety’ (Government of South Australia) Available at https://safecom-files-v8.s3.amazonaws.com/current/docs/brochure_your_guide_to_bushfire_safety_2014.pdf
Spolaor S, Fuchs C, Cazzaniga P, Kaymak U, Besozzi D, Nobile MS (2020) Simpful: A user-friendly python library for fuzzy logic. International Journal of Computational Intelligence Systems 13(1), 1687-1698.
| Crossref | Google Scholar |
Vacca P, Àgueda A, Muñoz JA, Planas E, Pastor E, Heymes F, Ismael E, Eyssette R, Cozzani V, Scarponi GE (2020a) WUIVIEW Deliverable 6.1 -Recommendations on structure survivability and sheltering capacity. Available at https://wuiview.webs.upc.edu/download/D6.1_F_Recommendationsonstructuresurvivabilityandshelteringcapacity.pdf
Vacca P, Caballero D, Pastor E, Planas E (2020b) WUI fire risk mitigation in Europe: A performance-based design approach at home-owner level. Journal of Safety Science and Resilience 1, 97-105.
| Crossref | Google Scholar |
Vacca P, Pastor E, Planas E (2022) Analysis of the load bearing capacity of concrete semi-confined spaces exposed to the combustion of two cars at the wildland–urban interface. In ‘Proceedings of the 10th International Seminar on Fire and Explosion Hazards’. (Eds K Vagsaether, AW Lach, D Bradley, J Lundberg) pp. 498–506. (University of South Eastern Norway)
Valis D, Pietrucha-Urbanik K (2014) Utilization of diffusion processes and fuzzy logic for vulnerability assessment. Eksploatacja i Niezawodnosc - Maintenance and Reliability 16(1), 48-55.
| Google Scholar |
Victoria Country Fire Authority (2022) Landscaping for Bushfire: Garden Design and Plant Selection. Available at https://www.cfa.vic.gov.au/ArticleDocuments/447/CFA%20Landscaping%20for%20Bushfire%20(Version%203).pdf.aspx?Embed=Y