Flexibility in reproductive attributes may facilitate the invasive capacity of the Mediterranean fanworm, Sabella spallanzanii (Gmelin)
Sarah C. Brand

A
B
C Present Address:
Abstract
The Mediterranean fanworm arrived as a non-indigenous species in parts of Australasia, where it has established and then rapidly spread from the initial points of arrival, despite marked differences in environmental conditions compared from its natal habitat in the Mediterranean Sea.
To better understand the invasive capacity of this species in New Zealand.
Maturation, reproductive cycle, and gametogenesis were investigated, with a focus on female gamete development, for a population in Auckland, and the results were compared with those of previous studies in the Mediterranean Sea and Australia.
Egg sizes in female fanworms were highly variable throughout the year; however, a reduced presence of larger eggs was observed from August to November, which could indicate spawning. This Auckland fanworm population also appeared to have a female-skewed sex ratio compared with previous studies where a 1:1 sex ratio was observed. Fanworm maturation was reached at ~6 cm in length, compared with 15 cm in natal populations. Fanworms with body size smaller than 5 cm were not mature and did not produce mature gametes, in contrast to an Australian fanworm population.
The observed Mediterranean fanworm population in New Zealand demonstrates reproductive flexibility and high fecundity, contributing to its invasive capacity.
The reproductive biology of a species is important in determining its invasive capacity, and may also be flexible between populations, with implications for effective biosecurity management strategies.
Keywords: fanworm, gametogenesis, invasive capacity, maturity, non-indigenous species, reproduction, Sabella spallanzanii, sex ratio.
Introduction
The Mediterranean fanworm, Sabella spallanzanii (Gmelin), is a filter-feeding marine polychaete that is native to the Mediterranean and Atlantic coasts of Europe, but it is also an invasive species, establishing in parts of Brazil and Indonesia, and more recently in Australia and New Zealand (Clapin and Evans 1995; Clapin 1996; Knight-Jones and Perkins 1998; Currie and Parry 1999; Currie et al. 2000; Fletcher 2014).
The Mediterranean fanworm was first detected in New Zealand in May 2008 in Port Lyttelton, in the South Island of New Zealand, where it persisted, despite extensive and expensive efforts to eliminate it (Read et al. 2011). Subsequently, this species has spread widely throughout eastern parts of New Zealand, most likely owing to a combination of their ability to hitchhike in ballast water, and as fouling on vessel hulls and sea chests (Molnar et al. 2008; Floerl et al. 2009; Inglis et al. 2010). After arriving in new port locations in New Zealand, this species has established and spread rapidly onto surrounding coastlines, despite attempts at intervention, which in total have cost millions of dollars (Fletcher 2014; Soliman and Inglis 2018).
The biological basis of the invasive capacity of this species is somewhat unclear. Within its native range in the Mediterranean Sea, the population reportedly remains fairly stable in Italy (Giangrande and Petraroli 1994; Giangrande et al. 2000; Giangrande et al. 2014; Giangrande et al. 2020). This species shows tolerance to a wide temperature range and is capable of establishing on soft-sediment seafloor habitats, as well as on hard rock substrates, plastic and cement surfaces (Holloway and Keough 2002; Giangrande et al. 2005; O’Brien et al. 2006; Davidson et al. 2011; Ross et al. 2013; Lee et al. 2018; Atalah et al. 2019; Douglas et al. 2020; Giangrande et al. 2020; Tait et al. 2020). A key factor in successful establishment of an invasive species in a new environment, apart from tolerance of varied environmental conditions, competitive ability and lack of predation pressure, is reproductive biology (Olyarnik et al. 2009; Dijkstra et al. 2017; Geburzi and McCarthy 2018).
The Mediterranean fanworm has separate sexes (i.e. dioecious), with no distinct ovaries or testes, where the gametes are produced within the coelom, floating freely in the coelomic fluid together with smaller coelomocytes (nutrient storage cells) that contribute to ripening of the gametes (Phillips Dales 1961; Currie et al. 2000; Giangrande et al. 2000; Read et al. 2014; Lee et al. 2018). The fanworm is thought to be a ‘sperm-caster’, where the sperm is released into the surrounding seawater but the eggs are retained inside the fanworm, where they are thought to be fertilised either internally or within the fanworm’s external protective tube (Currie et al. 2000; Giangrande et al. 2000; Stabili et al. 2009; Fletcher 2014; Read et al. 2014). The fertilised eggs are then released in long mucus strings into the water column (Currie et al. 2000; Bishop and Pemberton 2006; Stabili et al. 2009). The larvae of the fanworm are lecithotrophic with a pelagic phase of usually more than 2 weeks (Rouse and Fitzhugh 1994; Currie et al. 2000; Giangrande et al. 2000).
Previous studies on reproduction of the Mediterranean fanworm have used different classification systems to describe the maturation of gametes, which has made comparisons among different fanworm populations more difficult (Currie et al. 2000; Giangrande et al. 2000; Lee et al. 2018). Furthermore, oogenesis in this species appears to be variable, with ova of a range of sizes (20–220 μm in diameter) typically present simultaneously within the coelom (Read et al. 2014). The presence of some large ova among smaller ones may not be indicative of a pending spawning event, because some large ova may also be retained after a spawning event and fanworms that contain mature or ripe gametes might retain them while awaiting more favourable conditions for spawning (Currie et al. 2000; Giangrande et al. 2000; Read et al. 2014; Lee et al. 2018). In contrast to oogenesis, spermatogenesis is a fast process, where mature spermatozoa are produced within 3 months, where early spermatids range from small oval cells (7–10 μm) to when they reach maturity with a spherical head of 2 μm (Giangrande et al. 2000; Read et al. 2014). Currie et al. (2000) previously assessed maturation of male gametes by qualitative visual estimates of the percentage occurrence of gametes into four categories (0, no gametes; 1, low density; 2, moderate density; 3, high density), which is potentially dependant on which body segment has been sampled.
The aim of this research is to better understand the reproductive biology of an invasive population of S. spallanzanii in New Zealand, to determine how its reproductive behaviour has contributed to its rapid establishment and spread within New Zealand.
Methods
Field and laboratory sampling
To assess reproductive biology of an invasive population of S. spallanzanii in the Waitemata Harbour, Auckland, the progression of gametogenesis, spawning seasonality, sex ratio and the reproductive state of fanworms were assessed. The following two methods were used: (1) the development and size of eggs in relation to month and fanworm body size (i.e. body length) were measured in female fanworms, and (2) body size and progress of gametogenesis (according to classification of stages of gamete development and accumulation by Bybee et al. 2007) were noted for all sampled fanworms (i.e. male, female and indeterminate) over a 1-year period.
Each month for a year, 100 fanworms were sampled haphazardly from all of those available at the Orakei marina (36°50″59″S, 174°48″34″E) in the Waitemata Harbour, Auckland, New Zealand, from April 2018 until March 2019. The fanworms are abundant throughout the surfaces of the marina pontoons at a depth from 0.5 to 1 m. Individual fanworms of a variety of sizes (5–30-cm length) were haphazardly selected and carefully removed from their substrate by hand at 0.5-m depth from the top of the pontoon and were placed in a bucket filled with seawater and transported back to the laboratory for dissection.
Sampled fanworms were removed from their tubes and their length was measured from the thorax to the end of the abdomen, without considering the crown. Fanworms were then wet weighed without their tube (i.e. just the worm body and branchial crown). Sex was determined by visually examining fanworms under a binocular microscope and identifying the presence of either egg or sperm in the coelomic cavity. Where gametes were not present or identifiable, the specimen was classified as indeterminate. The sex ratio of sampled S. spallanzanii was calculated for each monthly sample and for the entire sampling period (i.e. 1 year).
To determine whether there was a seasonal component to the abundance of the different gametogenic stages and whether gametogenesis in the fanworms was synchronous, the coelomic fluid of a random subsample of ~50 fanworms per month was sampled and smeared on a microscope slide and the stage of gametogenesis was classified according to Bybee et al. (2007), which considers both the status of gametogenesis and the overall quantity of gametes present in the coelom. The length of each sampled fanworm was also recorded, to determine whether fanworms of a certain body size in a certain month fall into a specific gametogenesis stage.
From the 100 fanworms collected every month, a haphazardly selected subsample of 20–30 female fanworms (except for September 2018, when only 12 fanworms could be sampled) were selected for histological analysis to assess seasonal changes in egg development and fanworm size in relation to egg size and development, following the protocol of Currie et al. (2000) and Lee et al. (2018). Because the fanworms were collected haphazardly, there was not always a subset of exactly 20 female fanworms per month that had measurable eggs within them. At times gametes were absent from most of the coelomic cavities of the collected fanworms, which made sex determination or egg measurements impossible. A 2–3-cm longitudinal incision of the abdomen of female fanworms was used to provide a sample of coelomic fluid, containing gametes and coelomocytes, that was smeared on a microscope slide. The coelomic fluid was fixed, stained with eosin, and mounted with resin for further analysis under a Leica DMR fluorescent microscope. Egg size was measured and gamete development for both males and females was classified from the microscope slides, according to the method developed by Bybee et al. (2007) (Fig. 1). Digital images captured from the microscope were used to facilitate subsequent measurements of egg diameter of 100 haphazardly selected eggs per fanworm with image analysis software (ImageJ, ver. 1.49, see https://imagej.en.download.it/). For male fanworms no detailed measurements of the development of spermatozoa were taken beyond the classification of state of gametogenesis according to Bybee et al. (2007). This study was largely focused on measuring change in size and abundance of eggs in female fanworms, because oogenesis is energetically costly, affected by environmental factors such as food quantity, temperature and habitat condition, and egg-size difference and gametogenic development are more readily measurable in eggs than in sperm (Llodra 2002).
Histological images of the contents of the coelom of Mediterranean fanworm, illustrating the four stages of gametogenesis according to Bybee et al. (2007). Top left to right illustrate Stages 1 and 2 (1; 2), middle pictures from left to right illustrate Stages 3 and 4 with female gametes (3; 4) and bottom pictures from left to right illustrate Stages 3 and 4 with male gametes (5; 6).
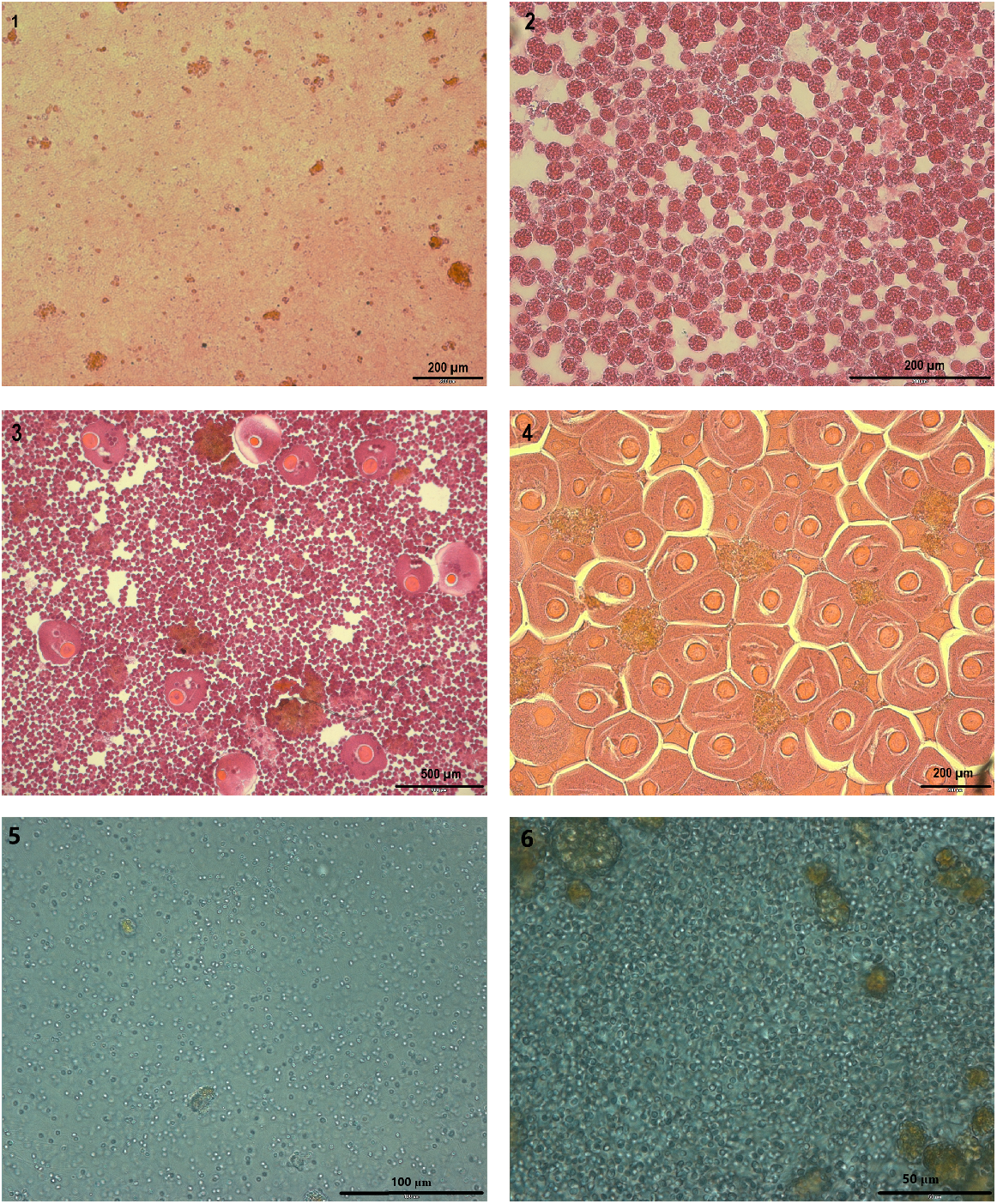
Statistical analysis
To test for differences in the body length and wet weight among the three different sex categories of sampled fanworms (i.e. female, male and of indeterminate sex), a linear regression analysis using the lm function in R and a Kruskal–Wallis test were performed, with a Bonferroni correction for multiple testing (ver. 4.2.2, R Foundation for Statistical Computing, Vienna, Austria, see https://www.r-project.org/). Departures of the sex ratio of fanworms from 1:1 for male : female was assessed using binomial tests for every monthly sample of fanworms, and for all monthly samples of fanworm for the year combined. Differences in body size and weight among fanworms that were male, female or of indeterminate sex were visualised using bar charts and boxplots (ggplot2, ver. 3.4.0, see https://CRAN.R-project.org/package=ggplot2; Wickham 2016). All descriptive statistics are reported as means ± standard deviations (s.d.).
To compare for effects of fanworm body length and months of sampling on egg size, a linear mixed effect model (random intercept model) was applied using the lme4 package (ver. 1.1.313.6.0, see https://CRAN.R-project.org/package=lme4; D Bates et al. 2015) and the lmer function in R). Model selection and best-fit model with the chosen parameters were informed by using Akaike information criterion (AIC), as well as by using maximum likelihood with Satterthwaite’s method (Burnham and Anderson 2004; Zuur et al. 2009). The final model was assessed using corresponding F statistics and restricted maximum-likelihood estimation (REML). The model used ‘month’ and ‘fanworm size’ as well as their potential interaction (month × fanworm size) as covariates and each individual fanworm (worm ID) as a random factor, with the 100 measured eggs nested within individuals. The model used April as the intercept. Tukey’s pairwise comparisons were used where the overall model was significant.
Prior to analyses, the normality and homogeneity of variances of the data were examined using residual plots and Levene’s test. Limited departures from these assumptions were deemed acceptable, given the large data set involved (Field et al. 2012). Least-square means were used for comparing the trends of differing egg sizes among the different months and fanworm size, and comparing different fanworm size among the months. Differences in egg diameter and fanworm size among months were visualised using bar charts (ggplot2, ver. 3.4.0).
The sex of fanworms cannot be determined in gametogenesis Stages 1 and 2, because there are only coelomocytes present, but no gametes to identify the sex (Bybee et al. 2007; Fig. 1). However, for Stages 3 and 4, the sex of fanworms could be determined because, in addition to the coelomocytes, male or female gametes were present in the coelom, allowing identification of sex (Fig. 1). Hence, the progress of gametogenesis, which can be inferred from egg size in female fanworms of Stages 3 and 4, was assessed for female fanworms in relation to time of year and to body size (Fig. 1). Since fanworms were selected haphazardly, for Stage 3 and Stage 4, there were also eight and nine male fanworms respectively, included in the visual stage classification. To test for any association among the numbers of female fanworms found in the different gametogenesis stages among the different months, a two-sided Fisher’s exact test was performed.
A two-way ANOVA was conducted to examine the effects of gametogenesis stage and month and their interaction with fanworm body size. Prior to analysis, the data were assessed for normality and homogeneity of variance by using residual plots and Levene’s test. For statistically significant ANOVA results, differences among means were identified using pairwise comparisons with a Bonferroni correction for multiple testing.
Results
Sex ratio
Of a total of 1193 fanworms that were examined over the year of sampling, there were more female (i.e. 661, 55.4%) than male (324, 27.2%) fanworms (χ2 = 74.037, d.f. = 11, P < 0.001), and 208 (17.4%) were of indeterminate sex. For 10 of 12 months of sampling, there were more female than male fanworms. Only in September and October, the ratio of female:male fanworms was at 1:1.
The body size of fanworms was different among the different sex categories (i.e. male, female and indeterminate), (R2 = 0.113, F(2, 1190) = 76.11, P < 0.001) with pairwise comparisons showing that both male and female fanworms were larger than were fanworms of indeterminate sex (P < 0.001). There was no difference in the size of female v. male fanworms (Fig. 2a). Sampled female fanworms ranged in size from 2 to 20 cm, with a mean of 10 ± 3 cm, male fanworms ranged in size from 3 to 19 cm, with a mean of 10 ± 3 cm and fanworms of indeterminate sex had a range of 2–19 cm, with a mean of 7 ± 3 cm (Fig. 2a). Fanworms also had a different body wet weight among the three sex categories (Kruskal–Wallis test, H(2) = 119.32, P < 0.001). Pairwise comparison showed that both male and female fanworms were heavier than were fanworms of indeterminate sex (P < 0.001), but there was no difference in body wet weight between male and female fanworms (Fig. 2b). Female fanworms ranged in weight from 0.2 to 9.4 g, with a mean weight of 3.5 ± 2.0 g, males ranged in weight from 0.1 to 8.1 g, with a mean of 3.3 ± 1.0 g, and fanworms of indeterminate sex ranged in weight from 0.1 to 8.7 g, with a mean weight of 2.0 ± 2.0 g (Fig. 2b). The size and wet weight of fanworms of indeterminate sex varied considerably among months, contributing to large standard deviations around the means (Fig. 2). For example, of the 208 indeterminate-sex fanworms, 78 were <5 cm in body length and 130 individuals ranged in body length from 6 to 19 cm. There tended to be greater numbers of larger fanworms of indeterminate sex (i.e. >5 cm long) in the months May (n = 19), August (n = 17), September (n = 17), October (n = 12), November (n = 15) and December (n = 17) (Fig. 3).
(a) Mean (±s.d.) fanworm size (i.e. body length), and (b) mean (±s.d.) wet weight in fanworms that were female (n = 661), male (n = 324) and of indeterminate sex (n = 208). Total fanworms (N = 1193) from monthly samples taken over a 1-year period. Different letters above error bars indicate comparative statistically significant results.
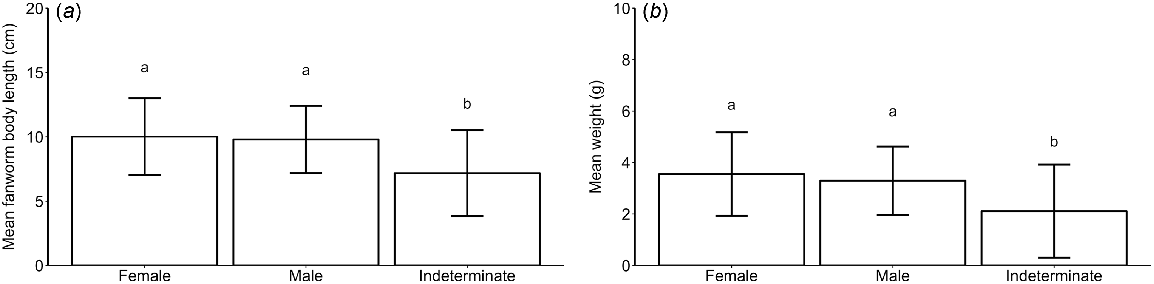
Oogenesis
In total, 22 885 eggs were measured in 230 female fanworms that were sampled over 12 consecutive months (i.e. ~20 female fanworms sampled every month).
Both of the covariate factors, ‘fanworm size’ (F(1, 206) = 15.65, P < 0.001) and ‘month’ (F(11, 206) = 4.94, P < 0.001), as well as the interaction of fanworm size and month (F(11, 206) = 2.90, P < 0.01) had a significant effect on egg size (Fig. 4).
(a) Monthly mean (±s.d.) egg diameter measured from a total of 230 female fanworms resulting from monthly samples over a 1-year period starting in April 2018. (b) Mean (±s.d.) egg diameter in a total of 230 female fanworms over a range of sizes that were sampled monthly over a 1-year period. (c) Mean (±s.d.) size (i.e. body length) of fanworms for monthly samples of 230 female fanworms sampled over 1 year, starting in April 2018.
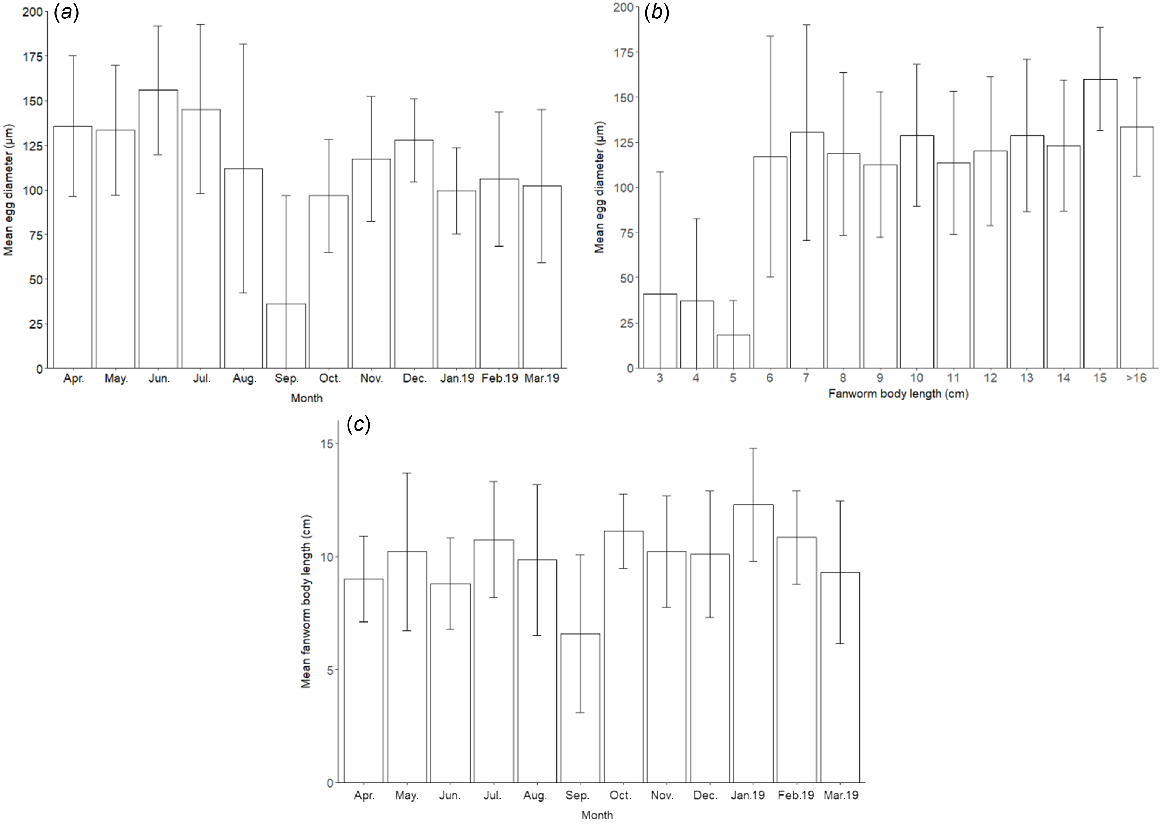
The smallest mean egg diameters were measured for the months September 2018 (36 ± 61 μm) and October 2018 (97 ± 32 μm) regardless of fanworm size. The months with the largest mean egg diameters were June 2018 (156 ± 36 μm) and July 2018 (145 ± 47 μm). Tukey’s pairwise comparison showed that the mean diameter of eggs in fanworm differed significantly between June and January–March (January: P = 0.0004; February: P = 0.009; March: P = 0.007) and between June and August–November (P < 0.05), although the P-values for October and November were marginally significant (October–November: P = 0.047; Fig. 4a), which was similar to the mean egg diameter in July v. October (P = 0.047). There was extensive variability in the measured egg diameter within each month, contributing to large standard deviations around the means. The measured egg diameter was most variable in August and September 2018, at the same time as the mean egg diameter was decreasing, because mainly smaller eggs were present, although some smaller numbers of larger eggs remained in some fanworms (Fig. 4a, b).
The mixed effect model showed an interactive effect among egg size, fanworm size and month (F(11, 206) = 2.90, P < 0.01). The body size of fanworms varied considerably among the different months (Fig. 4c). Mean diameter of the eggs in small fanworm of 3 cm in body length (41 ± 68 μm), 4 cm (37 ± 46 μm) and 5 cm (18 ± 19 μm) was considerably smaller than was the egg diameter in larger fanworms, i.e. from >6 cm in body length onward (117 ± 67 μm). The largest mean egg diameter was measured in a fanworm with a body length of >15 cm (160 ± 29 μm). For every sampled fanworm, the measured egg diameters were highly variable, indicating that eggs of a wide range of sizes were typically present in all fanworms of a body length of 6–16 cm (Fig. 4b). Fanworms of various length were sampled in every month and the mean fanworm length for most monthly samples was ~10 ± 2 cm, except for September 2018, when the mean fanworm length was 6.6 ± 4 cm, suggesting that in addition to the high variation, a greater proportion of smaller fanworms containing small eggs was sampled in September (P < 0.001; Fig. 4a, c).
Gametogenesis stages
There were marked differences in the gametogenesis stages of fanworms among the 12 months of sampling. Stage 1 gametogenesis (i.e. initiation of gametogenesis) in fanworms was higher than for all other months in the months of April and May (Fisher’s exact test, P < 0.001). Stage 2 gametogenesis in sampled fanworms was elevated in the months of August and September, whereas Stage 3 was similar among all sampled months. Stage 4 gametogenesis was most prevalent in January, March and June, and absent or barely present in September and October (Fig. 5). Despite haphazard sampling of fanworms, more female than male fanworms were collected and dissected for the histological analysis. Hence, there were only eight male fanworms included in Stage 3, spread over May, June, September, November and February and nine male fanworms at Stage 4 found in April, May and June. In Bybee’s et al. (2007) study, male Stage 3 worms were more abundant from March to June and Stage 4 worms from March to November.
The proportion of four different gametogenesis stages (Bybee et al. 2007) in monthly samples of fanworms over 12 consecutive months (N = 607), with the total number of fanworms sampled each month given in parentheses below the month.
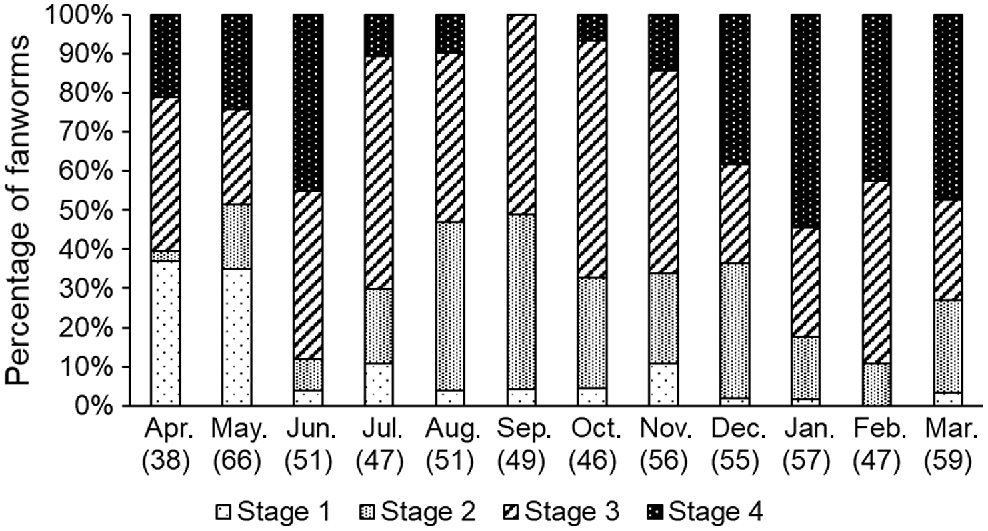
There was a significant interaction among fanworm size, gametogenesis stage and month (F(45, 575) = 5.65, P < 0.01), where fanworm size varied among the four gametogenesis stages among the various months (F(31, 575) = 1.86, P < 0.05). Specifically, fanworm size differed among the following months regardless of the gametogenesis stage: January and March, May, June, July, November and December (P < 0.05; Fig. 5).
Discussion
Over a year of sampling of S. spallanzanii in the Waitemata Harbour in Auckland indicated that female fanworms were more abundant than were males, except for September and October. This is markedly different from previous studies elsewhere, which have consistently found a 1:1 sex ratio (Currie et al. 2000; Giangrande et al. 2000).
The presence of generally larger eggs in fanworms sampled in June and July than those subsequently sampled in September and October suggests that one or several spawning events took place from July to November (Fig. 4). The presence of one or several seasonal spawning events during this time was also supported by a corresponding marked decrease in the proportion of mature gametogenesis stages at this time for both male and female fanworms (Fig. 5). However, eggs of a variety of sizes were frequently simultaneously present within individual female fanworms, ranging from the smallest egg measured (5 μm diameter) up to large eggs (220 μm diameter). In June and July, mainly larger eggs (>140-μm diameter) were measured in female fanworms, indicating that when the coelom is densely packed with mature gametes and less coelomocytes are present, that spawning is more likely to be imminent (Read et al. 2014).
The proportion of sampled fanworms, of both male and female, at Stage 4 (Bybee et al. 2007), which indicates the coelomic cavity is packed with gametes and low in coelomocyte numbers, was lower from July until November, further indicating that a single or multiple spawning events had occurred during that time (Fig. 1, 5). Furthermore, most male and female fanworms collected during these months were at Stages 1 and 2, indicating that they had no or very few gametes within the coelom, consistent with post-spawning recovery (Read et al. 2014). Regardless, fanworms at Stage 3, which indicates the presence of active sperm in males and eggs of mature sizes in females, were sampled in almost every month, suggesting the capacity for some individuals to be releasing mature gametes throughout the year (Fig. 1, 4 and 5).
Although it is not uncommon for polychaete worms to have a female-biased sex ratio, it typically occurs when polychaetes are protandric hermaphrodites and the sexes have different average body sizes (Petraitis 1991; Gosselin and Sewell 2013). However, in this current study in New Zealand, sex ratio was not a function of size in the Mediterranean fanworm, with no difference in the body length or wet weight between female and male fanworms, meaning that body length or weight in S. spallanzanii is not indicative of their sex (Fig. 2a, b). In this current study, no individual fanworms were observed to contain both types of gamete, which would have been indicative of hermaphroditism. Although it has been hypothesised at first, that S. spallanzanii is a protandric hermaphrodite (Giangrande and Petraroli 1994), subsequent research has confirmed that the species is dioecious, because only ever one type of gamete has been found to be present in each specimen at a time, and individuals are capable of repeated spawning, because eggs of a range of sizes are present within fanworms throughout the year (Read et al. 2014; Lee et al. 2018). Among the 607 fanworms closely examined in this current study, there was no evidence of hermaphroditism, confirming that S. spallanzanii is dioecious, and because there was no difference in body length or weight between male and female fanworms, it confirmed they are gonochoric and not protandric. It is possible for some species to vary their sex determination within different populations in response to local conditions, population structure and ecology that can affect sex ratio by local mate competition (Godfray and Werren 1996; Cook 2002). Some organisms have the capacity to adjust their sex ratios depending on population density (Gertzen et al. 2011), whereas others possess a flexible life-history strategy that allows them to adjust their physiological responses to increase their reproductive fitness depending on local environmental conditions (Collins et al. 2019).
In the current study, both female and male fanworms were larger in terms of both body length and weight than were fanworms of indeterminate sex (Fig. 2a, b). A lack of identifiable gametes makes sex classification of smaller fanworms impossible because they are most likely immature and unable to spawn (Fig. 2a, b). In this current study and in an Australian study (Lee et al. 2018), fanworms of indeterminate sex were found throughout the year. However, some larger fanworms were also found to be of indeterminate sex, ranging in body length from 6 to 19 cm, suggesting that these individuals had just spawned and released their gametes and, therefore, their coelomic cavity was empty, which prevented their sex from being determined. Alternatively, these fanworms could still be in the early stages of gametogenesis and had not yet started to produce new gametes (Olive 1970; Read et al. 2014).
It appears that in S. spallanzanii, gametogenesis can take place all year round, with female fanworms being at different stages of oogenesis, having different-sized eggs and differing quantities of coelomocytes at different times throughout the year (Murray et al. 2011). However, in the current study, eggs sampled in June and July were larger than those subsequently sampled in September and October. A previous study in New Zealand also found an absence of gametes among all sampled fanworms in the months of August and September (Jute 2015). By contrast, fanworm populations in the Gulf of St Vincent, Australia, have no clear difference in egg sizes among female fanworms sampled in the different months of the year and no indication of a spawning event for particular months (Lee et al. 2018). The greatest density of sperm in those Australian fanworms occurred from April to August; however, no clear pattern was detected and gamete abundance varied between the two tested locations (Lee et al. 2018). Male gamete density was low in austral summer months, but no synchronicity of gamete abundance between males and females was detected and both male and female gamete abundance showed high variation in density throughout the year (Lee et al. 2018). Therefore, although it appears that fanworms in this study were showing increased spawning activity in winter–spring, it is also likely that fanworms are spawning throughout the year (Lee et al. 2018).
Among smaller fanworms (<5 cm) it is most likely that they are insufficiently mature to produce viable gametes (Olive 1970, 1992). Smaller female fanworms (3–5 cm) tended to have mainly smaller eggs (20–40 μm in diameter) and only a few larger eggs or no larger eggs at all. By contrast, larger female fanworms that were >6 cm in body length generally contained eggs of a wide size range but tended to be dominated by larger eggs (i.e. >117 μm in diameter; Fig. 4). This indicated that fanworms in New Zealand with a body length of <5 cm have a greatly limited capacity to produce larger eggs (>200 μm in diameter) in significant quantities. By contrast, fanworms in the Mediterranean Sea only started producing large eggs (250 μm in diameter) at a body length of 15 cm (Giangrande et al. 2000), whereas fanworm populations in Port Phillip Bay and Gulf of St Vincent, Australia, started to produce large eggs (100–170 μm in diameter) at a body length of 5–7 cm (Currie et al. 2000; Lee et al. 2018). This indicates that the size of female fanworms at sexual maturity, as well as mature egg size, vary markedly among fanworm populations in different locations. It is common in marine invertebrates to have reproductive flexibility in populations in terms of body size in different geographic locations (Olive 1995; Sanford and Kelly 2011; AE Bates et al. 2013). Successful invasive or biofouling species often have wide tolerances (i.e. temperature, settlement structure, depth, environmental conditions), allowing them to establish in new environments (Lenz et al. 2011; AE Bates et al. 2013; Tepolt and Somero 2014; Wang and Feng 2016).
Oogenesis in fanworms can take between 9 and 12 months in the Mediterranean Sea until the eggs are mature, whereas spermatogenesis is a relatively rapid process, which is completed within 3 months (Giangrande et al. 2000). Similar observations have been made of fanworms in Port Phillip Bay, Australia, where oogenesis, spermatogenesis and the number of coelomocytes present followed a cycle (7–10 months) and resulted in roughly synchronised spawning events between June and August, on the basis of histological examination of male and female fanworms sampled monthly (Currie et al. 2000). By contrast, no synchronicity for male and female gametogenesis or seasonality has been found in a fanworm population in the Gulf of St Vincent, Australia (Lee et al. 2018). The results of the current study are similar to the study in Port Phillip Bay, Australia, in that both the observed reduction of ripe Stage 4 eggs in July to November and the measured larger-sized eggs in June and July are indicative of spawning taking place during those southern hemisphere winter–spring months (Currie et al. 2000).
Conclusions
Successful establishment of an invasive species is highly dependent on its tolerance to the environmental conditions in the new habitats and reproduction may be a key factor (Sakai et al. 2001; Weis 2010). Factors that influence reproductive success include early maturity, high fecundity and rapid growth rates, with all of these factors playing a significant role in the successful establishment and spread of an invasive species (Sakai et al. 2001; Weis 2010; Geburzi and McCarthy 2018). The high variability in egg size and ongoing production of eggs in addition to the greater proportion of females are indicative that S. spallanzanii has a high reproductive flexibility, which may help facilitate establishment and expansion of invasive populations. This resilience and strong reproduction appear to make the fanworm a highly successful invasive species; this would help explain the rapid colonisation and spread of this species in new locations, and also why elimination attempts have been so unsuccessful.
Data availability
The datasets generated during or analysed during the current study are available from the corresponding author on reasonable request. This paper forms part of the PhD thesis of Brand (2022).
Conflicts of interest
The authors declare that they have no known competing financial interests or personal relationships that could have appeared to influence the work reported in this paper.
Declaration of funding
This project was financially supported by the Coromandel Marine Farmers’ Association, Ministry for Primary Industries, Bay of Plenty Regional Council, Waikato Regional Council, Auckland Council and Northland Regional Council.
Acknowledgements
We thank Orakei Marina, Auckland, for providing access to the marina and support in conducting this study. We thank Sophie Roberts and Holly Fleming for their assistance with taking fanworm measurements and microscope images. We thank two anonymous reviewers for their helpful comments that improved the original manuscript. We are grateful to Kathy Walls who was instrumental in initiating and facilitating this research.
References
Atalah J, Floerl O, Pochon X, Townsend M, Tait L, Lohrer AM (2019) The introduced fanworm, Sabella spallanzanii, alters soft sediment macrofauna and bacterial communities. Frontiers in Ecology and Evolution 7, 481.
| Crossref | Google Scholar |
Bates AE, McKelvie CM, Sorte CJB, Morley SA, Jones NAR, Mondon JA, Bird TJ, Quinn G (2013) Geographical range, heat tolerance and invasion success in aquatic species. Proceedings of the Royal Society of London – B. Biological Sciences 280, 20131958.
| Crossref | Google Scholar |
Bates D, Mächler M, Bolker B, Walker S (2015) Fitting linear mixed-effects models using lme4. Journal of Statistical Software 67, 1-48.
| Crossref | Google Scholar |
Bishop JDD, Pemberton AJ (2006) The third way: spermcast mating in sessile marine invertebrates. Integrative and Comparative Biology 46, 398-406.
| Crossref | Google Scholar | PubMed |
Burnham KP, Anderson DR (2004) Multimodel inference: understanding AIC and BIC in model selection. Sociological Methods & Research 33, 261-304.
| Crossref | Google Scholar |
Bybee DR, Bailey-Brock JH, Tamaru CS (2007) Gametogenesis and spawning periodicity in the fan worm Sabellastarte spectabilis (Polychaeta: Sabellidae). Marine Biology 151, 639-648.
| Crossref | Google Scholar |
Clapin G, Evans DR (1995) The status of the introduced marine fanworm Sabella spallanzanii in Western Australia: a preliminary investigation. Technical report 2. Centre for Research on Introduced Marine Pests, CSIRO Division of Fisheries. doi:10.4225/08/585d669107bbc
Collins GE, Hogg ID, Convey P, Barnes AD, McDonald IR (2019) Spatial and temporal scales matter when assessing the species and genetic diversity of springtails (Collembola) in Antarctica. Frontiers in Ecology and Evolution 7, 76.
| Crossref | Google Scholar |
Cook MJ (2002) Sex determination in invertebrates. In ‘Sex ratios: concepts and research methods’. (Ed. ICW Hardy) pp. 178–194. (Cambridge University Press: Cambridge, UK) doi:10.1017/cbo9780511542053.009
Currie DR, Parry GD (1999) Changes to benthic communities over 20 years in Port Phillip Bay, Victoria, Australia. Marine Pollution Bulletin 38, 36-43.
| Crossref | Google Scholar |
Currie DR, McArthur MA, Cohen BF (2000) Reproduction and distribution of the invasive European fanworm Sabella spallanzanii (Polychaeta: Sabellidae) in Port Phillip Bay, Victoria, Australia. Marine Biology 136, 645-656.
| Crossref | Google Scholar |
Davidson AM, Jennions M, Nicotra AB (2011) Do invasive species show higher phenotypic plasticity than native species and, if so, is it adaptive? A meta-analysis. Ecology Letters 14, 419-431.
| Crossref | Google Scholar | PubMed |
Dijkstra JA, Westerman EL, Harris LG (2017) Elevated seasonal temperatures eliminate thermal barriers of reproduction of a dominant invasive species: a community state change for northern communities? Diversity and Distributions 23, 1182-1192.
| Crossref | Google Scholar |
Douglas EJ, Townsend M, Tait LW, Greenfield BL, Inglis GJ, Lohrer AM (2020) Sabella spallanzanii and seafloor biodiversity enhancement in a marine soft-sediment system. Diversity 12, 228-242.
| Crossref | Google Scholar |
Fletcher L (2014) Background information on the Mediterranean fanworm Sabella spallanzanii to support regional response decisions. Report Number 2479A. (Cawthron Institute: Nelson, New Zealand) Available at https://www.nrc.govt.nz/media/a2rniyui/cawthronsabellabackgroundreport.pdf
Floerl O, Inglis GJ, Dey K, Smith A (2009) The importance of transport hubs in stepping-stone invasions. Journal of Applied Ecology 46, 37-45.
| Crossref | Google Scholar |
Geburzi JC, McCarthy ML (2018) How do they do it? – understanding the success of marine invasive species. In ‘YOUMARES 8–oceans across boundaries: learning from each other’. pp. 109–124. (Springer Open) doi:10.1007/978-3-319-93284-2_8
Gertzen EL, Leung B, Yan ND (2011) Propagule pressure, Allee effects and the probability of establishment of an invasive species (Bythotrephes longimanus). Ecosphere 2, art30.
| Crossref | Google Scholar |
Giangrande A, Petraroli A (1994) Observations on reproduction and growth of Sabella spallanzanii (Polychaeta, Sabellidae) in the Mediterranean Sea. Memoires du Museum National d’Histoire Naturelle 162, 51-56.
| Google Scholar |
Giangrande A, Licciano M, Pagliara P, Gambi MC (2000) Gametogenesis and larval development in Sabella spallanzanii (Polychaeta: Sabellidae) from the Mediterranean Sea. Marine Biology 136, 847-861.
| Crossref | Google Scholar |
Giangrande A, Cavallo A, Licciano M, Mola E, Pierri C, Trianni L (2005) Utilization of the filter feeder polychaete Sabella spallanzanii Gmelin (Sabellidae) as bioremediator in aquaculture. Aquaculture International 13, 129-136.
| Crossref | Google Scholar |
Giangrande A, Caruso LPG, Musco L, Licciano M (2014) Variability among Mediterranean populations of Sabella pavonina (Annelida: Sabellidae). Italian Journal of Zoology 81, 100-111.
| Crossref | Google Scholar |
Giangrande A, Pierri C, Del Pasqua M, Gravili C, Gambi MC, Gravina MF (2020) The Mediterranean in check: biological invasions in a changing sea. Marine Ecology 41, e12583.
| Crossref | Google Scholar |
Godfray HCJ, Werren JH (1996) Recent developments in sex ratio studies. Trends in Ecology & Evolution 11, 59-63.
| Crossref | Google Scholar | PubMed |
Gosselin LA, Sewell MA (2013) Reproduction, larval development and settlement of the intertidal serpulid polychaete Spirobranchus cariniferus. Journal of the Marine Biological Association of the United Kingdom 93, 1249-1256.
| Crossref | Google Scholar |
Holloway MG, Keough MJ (2002) Effects of an introduced polychaete, Sabella spallanzanii, on the development of epifaunal assemblages. Marine Ecology Progress Series 236, 137-154.
| Crossref | Google Scholar |
Inglis GJ, Ahyong S, Cox SL, Unwin M, Ponder-Sutton A, Seaward K, Kospartov M, Read GB, Gordon D, Hosie A, Nelson W, d’Archino R, Bell A, Kluza D (2010) The biosecurity risk associated with biofouling on international vessels arriving in New Zealand: summary of the patterns and predictors of fouling. Biosecurity New Zealand Technical Paper Number 2008, Project RFP0811321 Number 182. NIWA.
Knight-Jones P, Perkins TH (1998) A revision of Sabella, Bispira and Stylomma (Polychaeta: Sabellidae). Zoological Journal of the Linnean Society 123, 385-467.
| Crossref | Google Scholar |
Lee AL, Dafforn KA, Hutchings PA, Johnston EL (2018) Reproductive strategy and gamete development of an invasive fanworm, Sabella spallanzanii (Polychaeta: Sabellidae), a field study in Gulf St Vincent, South Australia. PLoS ONE 13, e0200027.
| Crossref | Google Scholar | PubMed |
Lenz M, da Gama BAP, Gerner NV, Gobin J, Gröner F, Harry A, Jenkins SR, Kraufvelin P, Mummelthei C, Sareyka J, Xavier EA, Wahl M (2011) Non-native marine invertebrates are more tolerant towards environmental stress than taxonomically related native species: results from a globally replicated study. Environmental Research 111, 943-952.
| Crossref | Google Scholar | PubMed |
Llodra ER (2002) Fecundity and life-history strategies in marine invertebrates. In ‘Advances in Marine Biology’ 43. (Ed. MP Lesser) pp. 87–170. (Elsevier) doi:10.1016/s0065-2881(02)43004-0
Molnar JL, Gamboa RL, Revenga C, Spalding MD (2008) Assessing the global threat of invasive species to marine biodiversity. Frontiers in Ecology and the Environment 6, 485-492.
| Crossref | Google Scholar |
Murray JM, Watson GJ, Giangrande A, Bentley MG, Farrell P (2011) Reproductive biology and population ecology of the marine fan worm Sabella pavonina (Savigny) (Polychaeta: Sabellidae). Invertebrate Reproduction & Development 55, 183-196.
| Crossref | Google Scholar |
Olive PJW (1970) Reproduction of a Northumberland population of the polychaete Cirratulus cirratus. Marine Biology 5, 259-273.
| Crossref | Google Scholar |
Olive PJW (1992) The adaptive significance of seasonal reproduction in marine invertebrates: the importance of distinguishing between models. Invertebrate Reproduction & Development 22, 165-174.
| Crossref | Google Scholar |
Olive PJW (1995) Annual breeding cycles in marine invertebrates and environmental temperature: probing the proximate and ultimate causes of reproductive synchrony. Journal of Thermal Biology 20, 79-90.
| Crossref | Google Scholar |
O’Brien AL, Ross DJ, Keough MJ (2006) Effects of Sabella spallanzanii physical structure on soft sediment macrofaunal assemblages. Marine and Freshwater Research 57, 363-371.
| Crossref | Google Scholar |
Petraitis PS (1991) The effects of sex ratio and density on the expression of gender in the polychaete Capitella capitata. Evolutionary Ecology 5, 393-404.
| Crossref | Google Scholar |
Phillips Dales R (1961) The coelomic and peritoneal cell systems of some sabellid polychaetes. Journal of Cell Science S3-102, 327-346.
| Crossref | Google Scholar |
Read GB, Inglis G, Stratford P, Ahyong ST (2011) Arrival of the alien fanworm Sabella spallanzanii (Gmelin, 1791) (Polychaeta: Sabellidae) in two New Zealand harbours. Aquatic Invasions 6, 273-279.
| Crossref | Google Scholar |
Ross DJ, Longmore AR, Keough MJ (2013) Spatially variable effects of a marine pest on ecosystem function. Oecologia 172, 525-538.
| Crossref | Google Scholar | PubMed |
Rouse G, Fitzhugh K (1994) Broadcasting fables: is external fertilization really primitive? Sex, size, and larvae in sabellid polychaetes. Zoologica Scripta 23, 271-312.
| Crossref | Google Scholar |
Sakai AK, Allendorf FW, Holt JS, Lodge DM, Molofsky J, With KA, Baughman S, Cabin RJ, Cohen JE, Ellstrand NC, McCauley DE, O’Neil P, Parker IM, Thompson JN, Weller SG (2001) The population biology of invasive species. Annual Review of Ecology and Systematics 32, 305-332.
| Crossref | Google Scholar |
Sanford E, Kelly MW (2011) Local adaptation in marine invertebrates. Annual Review of Marine Science 3, 509-535.
| Crossref | Google Scholar | PubMed |
Soliman T, Inglis GJ (2018) Forecasting the economic impacts of two biofouling invaders on aquaculture production of green-lipped mussels Perna canaliculus in New Zealand. Aquaculture Environment Interactions 10, 1-12.
| Crossref | Google Scholar |
Stabili L, Schirosi R, Licciano M, Giangrande A (2009) The mucus of Sabella spallanzanii (Annelida, Polychaeta): its involvement in chemical defence and fertilization success. Journal of Experimental Marine Biology and Ecology 374, 144-149.
| Crossref | Google Scholar |
Tait LW, Lohrer AM, Townsend M, Atalah J, Floerl O, Inglis GJ (2020) Invasive ecosystem engineers threaten benthic nitrogen cycling by altering native infaunal and biofouling communities. Scientific Reports 10, 1581.
| Crossref | Google Scholar | PubMed |
Tepolt CK, Somero GN (2014) Master of all trades: thermal acclimation and adaptation of cardiac function in a broadly distributed marine invasive species, the European green crab, Carcinus maenas. Journal of Experimental Biology 217, 1129-1138.
| Crossref | Google Scholar | PubMed |
Wang R-F, Feng Y-L (2016) Tolerance and resistance of invasive and native Eupatorium species to generalist herbivore insects. Acta Oecologica 77, 59-66.
| Crossref | Google Scholar |
Weis JS (2010) The role of behavior in the success of invasive crustaceans. Marine and Freshwater Behaviour and Physiology 43, 83-98.
| Crossref | Google Scholar |